Dysmorphology evolved as a subset of clinical genetics that focused on standardizing the descriptive terminology used to define deviations from normal structural development in the context of syndromic disorders. These traits were termed birth defects and result from malformations, deformations, or disruptions, which generally have a significant and obvious effect on appearance ( Table 25.1 and Fig. 25.1 ). To identify the abnormal state, one has to be familiar with normal developmental stages, the timing of specific organ development, and developmental vulnerable periods ( Fig. 25.2 ). The dysmorphic physical examination is directed to overcome some of the clinical challenges of identifying and describing birth defects by providing a framework in which to differentiate normal human variable morphology from the abnormal in the context of a specific diagnosis.
Terminology | Definition | Example |
---|---|---|
Malformation sequence | Single, local tissue morphogenesis abnormality that produces a chain of subsequent defects | DiGeorge sequence of primary 4th brachial arch and 3rd and 4th pharyngeal pouch defects that lead to aplasia or hypoplasia of the thymus and parathyroid glands, aortic arch anomalies, and micrognathia |
Deformation sequence | Mechanical (uterine) forces that alter structure of intrinsically normal tissue | Oligohydramnios produces deformations by in utero compression of limbs (dislocated hips, equinovarus foot deformity), crumpled ears, dislocated nose, or small thorax |
Disruption sequence | In utero tissue destruction after a period of normal morphogenesis | Amnionic membrane rupture sequence, leading to amputation of fingers/toes, tissue fibrosis, and destructive tissue bands |
Dysplasia sequence | Poor organization of cells into tissues or organs | Neurocutaneous melanosis sequence with poor migration of melanocyte precursor cells from the neural crest to the periphery, manifesting as melanocytic hamartomas of skin, meninges, and so forth |
Malformation syndrome | Appearance of multiple malformations in unrelated tissues without an understandable unifying cause; with enhanced genetic investigation, a single etiology may become identified | Trisomy 21 Teratogens |


An international initiative to standardize the nosology used in clinical dysmorphology has been adapted to the Internet as an online resource supported by the National Human Genome Research Institute (NHGRI): https://elementsofmorphology-nih-gov.easyaccess2.lib.cuhk.edu.hk/ . These terms in themselves are of no clinical utility (aside from communicating the appropriate malformation to other providers) but when used with available database tools, can be a powerful adjunct to determining the final diagnosis. Examples of these terms are noted in Table 25.2 . The importance of reaching a diagnosis is to provide insight into the nature of the condition, enable appropriate counseling of recurrence risk, guide the necessary management recommendations, and provide the family with an overall framework of the natural history and prognosis of the disorder.
Terms Pertaining to the Face and Head
|
(See Nelson Textbook of Pediatrics, p. 899.)
Diagnostic Approaches
Often the geneticist-dysmorphologist is asked to view a child with the expectation that the total picture will lead to an instant identification of a syndrome or condition. Instant identification happens more frequently with the more common or better known conditions. Most often a diagnosis is difficult with many complex disorders; knowledge, skill, attention to detail, the use of the current tools, review of literature, and standard reference sources are required for diagnosis.
Human Variation
Normal human variation is enormous. A common sense argument can be made for variation by pointing out the ability of people to recognize and differentiate thousands of individuals whom they have met; computer programs for facial recognition are based on this premise. Nonetheless, humans differ little from one another at their DNA level; variation is currently estimated at approximately 0.1% or 1 base of DNA/1000 bases, which equates to roughly 6 coding variants/gene. The advent of molecular and biochemical diagnostic methods for identifying genes and gene products has begun to ease the burden on the geneticist by providing diagnostic and confirmatory tests for syndrome identification. Sequencing of the Human Genome clarified many prior preconceived notions regarding human genetics. Prior to the completion of the project, it was estimated that humans had approximately 100,000 genes, whereas in reality this number is closer to 23,000 genes. Of these genes, less than half have been associated with human disease and many have no clear function assigned to them at this time. This gene coding portion of the genome only accounts for approximately 1% of the total genomic code, which consists of roughly 6 billion nucleotides. DNA analysis when available may provide the genotype and confirm the diagnosis, but it cannot unerringly define the phenotype.
There is still much to learn about our genetic code and how genes are expressed and regulated; even if we could perform genomic sequencing on every patient, there would be a number of patients in whom the molecular diagnosis remained elusive. The current diagnostic rate for exome sequencing is approximately 25%, this improves to about 30% with exome trios in which selected relatives are sequenced along with the affected individual and used to assess allele segregation with the phenotype.
There are common genetic pathways that relate genes within a pathway to common groups of disorders. This has provided an explanation to clinicians why seemingly disparate disorders share certain disease associations but remain clinically distinct. An example of this is the RASopathies ( Fig. 25.3 ), in which germline pathogenic variants in KRAS can result in the classic Noonan phenotype or cardiofaciocutaneous syndrome. There is genetic heterogeneity in this group of disorders which share several overlapping features. In addition, somatic mosaicism for genes in this pathway has been identified to cause several capillary/vascular malformation disorders. Another example is the allelic disorders involving the TRPV4 gene, which include brachyolmia type 3, digital arthropathy–brachydactyly, hereditary motor and sensory neuropathy type IIc, metatropic dysplasia, parastremmatic dwarfism, scapuloperoneal spinal muscular atrophy, spondyloepiphyseal dysplasia Maroteaux type, spinal muscular atrophy, and spondylometaphyseal dysplasia Kozlowski type. The wide phenotypic variability ranging from primary skeletal dysplasias to isolated neuromuscular disease speaks to the complexities of gene regulation and tissue-specific expression.

In addition to the primary gene code, there are tertiary elements that can be imprinted , in which gene expression is controlled by parent of origin or even be affected by the environment, the concept of epigenetic control. Further variability exists in genomic copy number variations , some of which are considered normal variants, while others result in recognizable microdeletion or microduplication disorders such as velocardiofacial syndrome (VCFS) and Smith–Magenis syndrome.
Not all congenital malformations or birth defects are primarily genetic. Teratogenic exposure, vascular events, and extrinsic factors, such as amniotic bands, all have the potential to result in deviations from normal morphologic development ( Fig. 25.4 ).

Teratology
(See Nelson Textbook of Pediatrics, p. 814.)
Teratogens are agents that affect normal development and can give rise to congenital birth defects. For the most part, teratogens are considered to be chemical agents, such as thalidomide or alcohol. However, perinatal infections with cytomegalovirus would fall into this broad category as would significant radiation exposure. The minority of fetuses exposed to potential teratogens show effects, even if exposed at the same time with the same dose of the agent (e.g., alcohol, 30%; thalidomide, 20%; hydantoins, 10%; warfarin, 8%; lithium, 7%; and diazepam, 1%). The exact determinants why some fetuses are affected are poorly understood.
Embryologic timing is one of the critical elements that define the final outcome. There are broadly 3 periods identified in fetal development (see Fig. 25.2 ).
-
Implantation: Period of fertilization through gastrulation and formation of the embryonic plate (first 2 weeks after fertilization). Significant interference with development during this time usually results in loss of conceptus.
-
Embryonic: This is the period of primary tissue differentiation, and thus, the period at greatest risk for major malformations (weeks 3 through 8).
-
Fetal: At this time, primary organogenesis is complete, but growth and neuronal migration proceed. The central nervous system (CNS) is at risk and many of the minor birth defects arise during this time (9 weeks through birth).
Some teratogens may have delayed effects, and these do not result in an overt congenital malformation; diethylstilbestrol (DES) exposure in a female fetus can predispose to vaginal clear cell carcinoma in puberty.
Embryogenesis
The developmental timing of the event that results in the final phenotype is one of the critical determinants of the phenotypic outcome. When considering embryologic processes, timing is one element but other important concepts are important to aid understanding of the final outcome. The timing is important because multiple developmental processes are occurring at the same time and thus a number of malformations present concomitantly as a result of interference with everything developing at the same embryonic time; radial ray defects may be seen with cardiac septal defects as in Holt–Oram syndrome. The common embryologic origin of various elements can give rise to overlapping disorders with shared elements: branchial arch developmental field defects in VCFS or disorders caused by abnormal neural crest cell migration. Critical embryologic events can give rise to disorders due to failure of a specific embryologic process: Neural tube defects arise as a result of abnormal neural tube fusion/closure.
Birth Defects
(See Nelson Textbook of Pediatrics, p. 899.)
It is estimated that approximately 15% of newborns have 1 minor anomaly; 0.8% have 2 minor anomalies, and 0.5% have 3. The more minor anomalies that are present, the greater is the probability that an underlying syndrome or a major organ anomaly is also present. Statistically, this equates to a 5-fold risk if 2 minor anomalies are present and a 20-30% probability that there is a major anomaly (congenital heart disease, renal, CNS, limb) if 3 minor anomalies are present. Approximately 50% of major anomalies involve the head and neck region. The Centers for Disease Control and Prevention statistics for the United States assert that a baby is born with a birth defect every 4.5 minutes; in 2010, birth defects accounted for about 1 in 5 infant deaths in the United States. Examples and potential etiologies are noted in Table 25.3 .
Monogenic (7.5% of Serious Anomalies) |
X-linked hydrocephalus |
Achondroplasia |
Ectodermal dysplasia |
Apert disease |
Treacher Collins syndrome |
Chromosomal (6% of Serious Anomalies) |
Trisomies 21, 18, 13 |
XO, XXY |
Deletions 4p– 5p–, 7q–, 13q–, 18p–, 18q–, 22q– |
Prader–Willi syndrome (50% have partial deletion of chromosome 15) |
Maternal Infection (2% of Serious Anomalies) |
Intrauterine infections (e.g., herpes simplex, CMV, varicella-zoster, rubella, and toxoplasmosis) |
Maternal Illness (3.5% of Serious Anomalies) |
Diabetes mellitus |
Phenylketonuria |
Hyperthermia |
Uterine Environment (% Unknown) |
Deformation |
Uterine pressure, oligohydramnios: clubfoot, torticollis, congenital hip dislocation, pulmonary hypoplasia, 7th nerve palsy |
Disruption |
Amniotic bands, congenital amputations, gastroschisis, porencephaly, intestinal atresia |
Twinning |
Conjoined twins, intestinal atresia, porencephaly |
Environmental Agents (% Unknown) |
Polychlorinated biphenyls |
Herbicides |
Mercury |
Alcohol |
Medications (% Unknown) |
Thalidomide |
Diethylstilbestrol |
Phenytoin |
Warfarin |
Cytotoxic drugs |
Isotretinoin (vitamin A) |
d -Penicillamine |
Valproic acid |
Unknown Etiologies |
Polygenetic |
Anencephaly/spina bifida |
Cleft lip/palate |
Pyloric stenosis |
Congenital heart disease |
Imprinting of Genes |
Prader–Willi syndrome |
Beckwith–Wiedemann syndrome |
Sporadic Syndrome Complexes (Anomalads) |
CHARGE syndrome |
VATER syndrome |
Pierre Robin syndrome |
Prune-belly syndrome |
Nutritional |
Low folic acid–neural tube defects |
Persons in the same family or ethnic groups may superficially resemble one another; any attempt at identifying a condition as an abnormality should include inspection of close relatives. Unusual morphologic findings in a child who resembles his or her parents does not exclude a dysmorphic condition. The parents might have variation in expression of the disorder or there could be additional features which are distinct that need to be separated from the common familial morphology.
Clinical Classification
Single-System Defects
- •
Most common of all birth defects
- •
Isolated to a single organ system
- •
Clinically similar to organ malformations seen in syndromes due to common pathways and same-organ end-point
- •
Examples: isolated cleft lip/palate; congenital heart disease; distal limb anomalies
Association
- •
Statistically ascertained nonrandom co-occurrences of multiple anomalies in which a single underlying cause is not identifiable. Usually, a diagnosis of exclusion.
- •
Creates an awareness to evaluate for associated anomalies
- •
<1% risk for recurrence
- •
Example: VATER/VACTERL (vertebral, anal atresia, cardiac, tracheoesophageal fistula, renal anomalies, limb malformations—typically radial ray) ( Figs. 25.5 and 25.6 )
FIGURE 25.5 VATER association as initially set forth. A, Young infant with vertebral anomalies, anal atresia, esophageal atresia with tracheoesophageal fistula, radial aplasia on the left, and thumb hypoplasia on the right. B, Relative frequencies of some of the other VATER association defects when the patient is ascertained by virtue of having 1 of the defects. C, Same patient at 2 years of age, with normal intelligence.(From Jones KL, Jones MC, Del Campo M, eds. Smith’s Recognizable Patterns of Human Malformation . 7th ed. Philadelphia: Elsevier; 2013:852.)FIGURE 25.6 Left, Expanded VACTERL association of defects. Right, Note the relatively severe thumb (radial) defect of the right hand and the much more subtle “radial” defect of the left hand (arrow). The arrow depicts a hypoplastic thenar eminence and crease.(From Jones KL, Jones MC, Del Campo M, eds. Smith’s Recognizable Patterns of Human Malformation . 7th ed. Philadelphia: Elsevier; 2013:853.)
Sequence
- •
A cascade of effects from a single localized abnormality in early morphogenesis that results in multiple congenital anomalies
- •
Example: Potter sequence secondary to renal agenesis and severe oligohydramnios ( Figs. 25.7 and 25.8 )
FIGURE 25.7 A–C, The consequences of renal agenesis. Note the multiple deformational defects in B, and the amnion nodosum (brown-yellow granules from vernix that have been ribbed into defects of the amniotic surface) in C .(From Jones KL, Jones MC, Del Campo M, eds. Smith’s Recognizable Patterns of Human Malformation . 7th ed. Philadelphia: Elsevier; 2013:821.)FIGURE 25.8 A, This diagram demonstrates the etiologically heterogeneous phenotype that results from fetal akinesia. B, This infant was born with myotonic dystrophy to a mother with the same condition. He had multiple joint contractures with thin bones and respiratory insufficiency. C, This infant was immobilized in a transverse lie after amnion rupture at 26 weeks. D, This fetus had bilateral renal agenesis resulting in oligohydramnios.(From Graham JL. Smith’s Recognizable Patterns of Human Malformation . 3rd ed. Philadelphia: Elsevier; 2007:287; Figure 47-2.)
Syndrome
- •
The presence of multiple structural/functional defects due to a single cause.
- •
Example: Down syndrome caused by trisomy for chromosome 21 ( Fig. 25.9 ) or other trisomies ( Fig. 25.10 and Tables 25.4, 25.5, and 25.6 )
FIGURE 25.9 Facial appearance of a child with Down syndrome.(From Wiedemann HR, Kunze J, Dibbern H. Atlas of Clinical Syndromes: A Visual Guide to Diagnosis . 3rd ed. St. Louis: Mosby; 1989.)

Full access? Get Clinical Tree
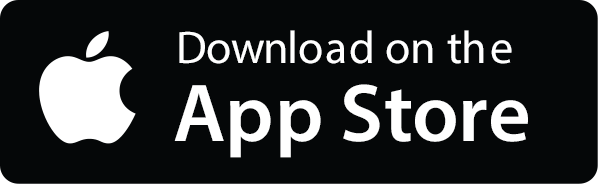
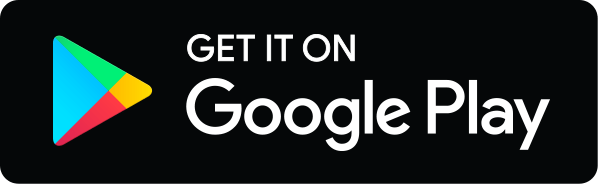