INTRODUCTION
There are many inborn errors of metabolism (IEMs) that cause disease in humans, and hundreds of these can produce encephalopathy and seizures. In fact, we now know of 7,000 rare diseases affecting 25 to 30 million people in the United States, and many of these conditions affect the developing brain. It is a formidable challenge for the clinician, to say the least. How can one promptly and efficiently identify these conditions? In some cases, the clinical manifestations are distinct enough to allow skilled clinicians to suspect specific disorders, but in many other circumstances, a wide variety of disorders present with similar manifestations: early onset of encephalopathy, seizures with a mixture of diffuse and focal elements, and diffuse background EEG slowing with admixed multifocal spikes. To complicate matters further, the full phenotypic spectrum of many of these disorders has not yet been identified, so selective biochemical testing may miss important conditions if one were to rely entirely upon clinical suspicion. At the same time, widespread biochemical screening tests of affected children may not be cost efficient, are certainly time consuming, and can produce false positives. Newborn screening of treatable conditions has helped and could be considered a model of rational public health intervention. The rationale of neonatal blood screening is to identify treatable conditions early, before they exert their damaging effects, and over the years there has been an expansion in the testing repertoire. As clinicians, we ultimately will prefer to be proactive in protecting the presymptomatic patient, rather than reactive in attempting to rescue the postsymptomatic patient. Phenylketonuria is the perfect example. In Illinois, screening began with a screen for phenylketonuria in 1965. Currently, more than 40 conditions are evaluated, including urea cycle defects, biotinidase deficiency, fatty acid oxidation disorders, lysosomal storage disorders, and organic acid disorders. Useful as this is, only a minority of the IEMs is covered.
The technological advances behind next-generation sequencing offers the promise that a large number of genetically determined disorders may someday soon be screened in one fell swoop, recognizing that the depth of coverage of this technology, the interpretive complexities involving variants of unknown significance, and the ethics of discovering unrelated genetic information are persistent thorny issues. Notwithstanding these issues, the increasing use of epilepsy panels to screen for genes known to be associated with early-life epilepsy is producing dramatic results and suggests that widespread genetic screening will become a reality at some point. In the present day, however, the clinician must continue to struggle with intellectual mastery of IEMs or risk missing important opportunities to effectively treat patients and better understand the pathophysiology of epilepsy.
Taking all of this into consideration, what follows in this chapter is a practical guide to some of the most important IEMs causing pediatric epilepsy. It is by no means encyclopedic or exhaustive; given the pace of discovery, it will also lack some recent insights, but fortunately excellent web-based resources, like Online Mendelian Inheritance in Man (www.omim.org), exist. Our purpose is to provide the reader with a broad overview of the topic and, wherever possible, relevant and practical clinical clues to accurate diagnosis.
When to Suspect an Inborn Error of Metabolism
An IEM should be considered when epilepsy presents early in life, is refractory, has features that are not consistent with an otherwise explanatory electroclinical syndrome, has an unexplained encephalopathy, or the child has neurologic regression. A combination of all of these is very suggestive. Other important clues might include family history, the presence of other unexplained neurologic or physical signs (e.g., ataxia, retinopathy, rash, organomegaly, peculiar odors, etc.), and distinctive findings on neuroimaging (see Tables 36.1, 36.2, and 36.3).
EEG Can Help: Supportive EEG abnormalities commonly observed in IEMs include background slowing, lack of the expected organization for age, multifocal spikes, and loss of normal physiological signposts of maturation. These individual features are etiologically nonspecific, but they often occur in a cluster and are frequently found in children with epileptogenic encephalopathies, of which a subset will be caused by IEMs.
TABLE 36.1
INBORN ERRORS OF METABOLISM CAUSING EME |
Organic/amino acidurias Urea cycle defects Disorders of subcellular organelles: Peroxisomes Mitochondria Menkes disease Pyridoxine dependency |
The most severe disorders of metabolism will produce dramatic EEG changes with epochs of discontinuity and electrodecrements. In these conditions the epilepsy itself may contribute to encephalopathies, and these are referred to as “epileptic encephalopathies.” There is a spectrum of these disorders where the precise clinical and electrographic manifestations are age-dependent. Unexplained early-onset epileptic encephalopathy, including early myoclonic epilepsy as described by Aicardi, West syndrome, and Lennox–Gastaut syndrome, may be caused by IEMs.
Common Pathogenic Mechanisms
Neuronal health and well-being depends upon the intricate balance of thousands of simultaneous chemical reactions involving hundreds of pathways and scores of different classes of compounds. Molecules shuttle between one pathway and the next, sometimes acting as building blocks for one pathway and at other times as a part of the metabolism of another. An individual compound might simultaneously modulate the speed of several enzymatic reactions, influence the bioenergetics of the cells, or act as a critical neurotransmitter. It is no surprise, therefore, that some of the IEMs have only one mechanism of action responsible for the generation of seizures and neurological injury. More often, several mechanisms are at work, including the imbalance of excitatory and inhibitory neurotransmission, a reduction in available fuel for normal cell processes, accumulation of toxic substances, cytotoxic cell edema with resultant poor perfusion, and interference with normal maturation of the nervous system.
TABLE 36.2
IMPORTANT DIAGNOSTIC TESTS FOR EME |
Complete blood count Chemistry profile including liver function tests Urine organic acids Amino acid profile in CSF, blood, and urine Lactate/Pyruvate in CSF and blood Serum ammonia Urine copper, serum cerruloplasmin (check for Menkes) Very long chain fatty acids Pyridoxine challenge, pyridoxal 5-phosphate challenge, or both |
TABLE 36.3
INBORN ERRORS OF METABOLISM CAUSING SEIZURES IN THE NEWBORN |
Nonketotic hyperglycinemia Isovaleric, methylmalonic, and propionic aciduria Maple syrup urine disease Pyridoxine dependency, pyridoxal phosphate dependency Biotinidase deficiency D-2-hydroxyglutaric aciduria 3-phosphoglycerate dehydrogenase deficiency Adenylsuccinate lyase deficiency Methylene tetrahydrofolate reductase deficiency (MTHFR deficiency) GABA transaminase deficiency Congenital disorders of glycosylation Developmental delay, epilepsy, and neonatal diabetes (DEND) Hyperinsulinism, hyperammonemia (HiHA) Neonatal ceroid lipofuscinosis with cathepsin deficiency Creatine deficiency Peroxisomal disorders Urea cycle disorders Dihydropyrimidine dehydrogenase deficiency Molybdenum cofactor deficiency Carnitine palmitoyltransferase types I and II Pyruvate carboxylase deficiency Pyruvate dehydrogenase deficiency Disorders of biotin |
Energy Failure
A central and critical element of epileptogenesis in an IEM is energy failure. glutamine transporter 1 deficiency syndrome (Glut1 DS) can be considered a prototype (1). In this condition there is a failure of adequate transport of glucose into the central nervous system because of a deleterious mutation in the glucose transporter gene. The body has an adequate concentration of glucose, so there are no systemic symptoms and no ketosis, which deprives the brain of both of its typical energy sources and results in both paroxysmal symptoms and continual dysfunction. The disease commonly presents within the first year of life when the relative energy demands of the young brain markedly exceed those at any other time of life. Not all regions of the brain appear to be equally effected; involvement of the thalamus and other deep gray structures is commonly seen in PET studies (2). This explains the development of generalized spike–wave discharges and refractory absence seizures, since these are known to arise from pathology in the thalamo-cortical regions. Other IEMs that deprive the brain of adequate energy, including respiratory chain defects and mitochondrial disorders, often involve the deep gray nuclei to an even more profound degree, so that visible changes are seen on MR imaging (3). Since energy is required to move ions across electrochemical gradients and regulate the production of the essential neurotransmitters, it is easy to understand how energy failure could produce refractory seizures, particularly in metabolically vulnerable regions of the brain.
Imbalance of Excitation and Inhibition
In other circumstances IEMs can produce an imbalance in the ratio of excitatory to inhibitory neurotransmitters. A good example of this category is pyridoxine-dependent epilepsy, where the pretreatment depleted pyridoxine reserves limit the decarboxylation of glutamate to GABA (4). This results in excessive excitation, seizures, and profound EEG abnormalities, including burst-suppression and hypsarrhythmia.
Accumulation of Toxic Substances
A buildup of toxic substances occurs in a variety of storage disorders, urea cycle defects, amino acidurias, and organic acidurias. Substances like ammonia may directly and severely impair neurologic function, resulting in encephalopathy and seizures.
NEONATAL
There are four well-established epilepsy syndromes presenting in the newborn. Two have largely normal EEG backgrounds and are not associated with IEMs; namely, benign neonatal convulsions and benign familial neonatal convulsions. Two others have markedly disorganized backgrounds with burst-suppression; namely, early myoclonic encephalopathy (EME) and Ohtahara syndrome. EME has been associated with IEMs.
Early Myoclonic Encephalopathy
EME is characterized by refractory myoclonic or epileptic spasms as well as focal seizures accompanied by a state-dependent burst-suppression EEG pattern with variable intervals. Published causes of EME include nonketotic hyperglycinemia, methylmalonic acidemia, propionic acidemia, D-glyceric acidemia, sulfite oxidase deficiency/molybdenum cofactor deficiency, Leigh’s syndrome, carbamyl–phosphate synthetase deficiency, pyridoxine dependency, Menkes disease, and peroxisomal disorders. Clearly, organic and amino acidurias are the most important contributors, and the most prominent of these is nonketotic hyperglycinemia. One could simplify this list to the following six considerations: organic/amino acidemias including nonketotic hyperglycinemia, urea cycle defects, mitochondrial disorders, defects of the peroxisomes, Menkes disease, and pyridoxine dependency.
Nonketotic Hyperglycinemia or Glycine Encephalopathy
Nonketotic hyperglycinemia is an autosomal recessive inborn error of amino acid metabolism, also known as glycine encephalopathy, where large amounts of glycine accumulate in the body fluids because of a defect in the multienzyme complex for glycine cleavage. This mitochondrial enzyme system has four protein components: P, H, T, and L. The most common mutation causes P protein dysfunction (involving the GLDS gene), but the exact pathophysiological processes have not been fully elucidated. Glycine level is an inhibitory transmitter in the brainstem and spinal, cord but is also an excitatory transmitter acting upon NMDA receptors in the cortex. Glycine may also impair cellular energetics by interfering with citrate synthetase function and mitochondrial electron transport.
Early descriptions of this disorder in the 1960s characterized the phenotype: affected neonates usually have lethargy, respiratory difficulties, and seizures in the first 48 hours (5). Hiccoughs (hiccups) may be seen by the fourth or fifth day of life. Seizures are often myoclonic or spasms and are accompanied by a burst-suppression pattern (6). As patients with these disorders mature, infantile spasms may persist, and the EEG often reveals hypsarrhythmia. Cortical malformations or corpus callosum defects may be present. Diffusion-weighted changes have been noted on MRI in the acute stages that may be suggestive of the disorder (7).
Of note, a transient form of nonketotic hyperglycinemia exists with similar early clinical and biochemical findings. In this condition, however, glycine concentrations normalize between 2 and 8 weeks after birth, resulting in a favorable prognosis (8).
Treatment strategies involving benzoate, carnitine supplementation, ketamine, and dextromethorphan have reported improvement in EEG and seizure control, but no normalization of development (9–12).
Other Important IEMs Causing Nonsyndromic Neonatal Seizures
Some newborns with IEM do not present with well-characterized epilepsy syndromes. Some could be considered to have an early-onset epileptic encephalopathy (EOEE) (13). Still other patients may show a mixture of focal, myoclonic, and tonic seizures with backgrounds that are abnormal but do not meet criteria for burst-suppression. As a consequence, neonates with persistent seizures without a clearly identifiable cause should be evaluated for an IEM and the newly discovered channelopathies.
IEMs that present in the newborn include: nonketotic hyperglycinemia; isovaleric, methylmalonic, and propionic aciduria; maple syrup urine disease; pyridoxine dependency and pyridoxal phosphate dependency; biotinidase deficiency; D-2-hydroxyglutaric aciduria and 3-phosphoglycerate dehydrogenase deficiency; adenylsuccinate lyase deficiency; methylene tetrahydrofolate reductase deficiency (MTHFR deficiency); GABA transaminase deficiency; congenital disorders of glycosylation; developmental delay, epilepsy, and neonatal diabetes (DEND); hyperinsulinism, hyperammonemia (HiHA); neonatal ceroid lipofuscinosis with cathepsin deficiency; creatine deficiency; peroxisomal and urea cycle disorders; dihydropyrimidine dehydrogenase deficiency; molybdenum cofactor deficiency; carnitine palmitoyltransferase types I and II; pyruvate carboxylase deficiency; pyruvate dehydrogenase deficiency, and disorders of biotin.
Pyridoxine Dependency
Pyridoxine dependency presents with refractory seizures typically within the first several days after birth, characterized by infantile spasms or a variety of focal, myoclonic, and atonic seizures (14). The condition is caused by mutations in the antiquitin gene, which codes for an enzyme in lysine metabolism (α-aminoadipic semialdehyde dehydrogenase) (4). As a result, a variety of substrates accumulate, including α-aminoadipic semialdehyde (AASA) and L-Δ1-piperideine-6-carboxylate (P6C). The latter undergoes a chemical condensation with pyridoxal 5-phosphate (PLP), thereby lowering PLP-free levels. Both AASA and P6C may be neurotoxic by themselves, and low PLP levels most likely alter the balance between glutamate and GABA, since PLP is a necessary cofactor for glutamate decarboxylase function. It is important to note that there may be later presentations of pyridoxine dependency with seizures later in infancy or seizure-free intervals of several months without supplementation (15).
The EEG is characterized by generalized bursts of high-voltage delta with intermixed spike-and-sharp waves and periods of asynchronous attenuation. Since 1954 it has been known that treatment with high doses (100–200 mg intravenously) of pyridoxine can have a dramatic early effect on seizures and the resting EEG (14). There may be immediate cessation of seizures, conversion of the EEG burst-suppression pattern, and later normalization of the EEG with subsequent oral doses (16).
A related condition is pyridoxal 5′-phosphate dependent epilepsy (PLP-DE). This is most often due to mutations in the gene encoding pyridoxine 5′-phosphate oxidase (PNPO). Like pyridoxine-dependent epilepsy (PDE), this condition presents with intractable neonatal or infantile seizures, but instead responds only to PLP, the active form of pyridoxine.
Evaluation and Treatment of PDE and PLP-DE
Plasma pipecolic acid as well as urine and plasma samples of AASA can help to support the diagnosis. The recommended oral dose of either pyridoxine or PLP is 30 mg/kg/day (17,4). Since there is no readily available intravenous preparation of PLP, some authors administer 100 mg of IV pyridoxine and if there is no clear EEG response, repeat the dose and then treat with oral PLP while the results of biochemical tests (including CSF, serum markers, and urine markers of PDE and PLP-DE) are awaited. Suspected cases can be confirmed with genetic mutational analysis of either condition. Intravenous treatment or even oral replacement can result in transient apnea, so practitioners should be prepared to support respirations before administering pyridoxine (18).
Molybdenum Cofactor Deficiency and Sulfite Oxidase Deficiency
These rare conditions manifest shortly after birth with a progressive encephalopathy, feeding difficulties, hypotonia, and refractory partial, myoclonic, or apparently generalized seizures. Dysmorphic features, lens dislocation, and hepatomegaly are characteristic (19–21). Neuroimaging may show poor differentiation between the gray and white matter, severe cerebral and cerebellar atrophy, and multiple cystic cavities in the white matter. The relatively more common molybdenum cofactor deficiency results from an absence of the hepatic cofactor, which leads to a combined enzyme deficiency of the three molybdenum-dependent enzymes: sulfite oxidase, xanthine dehydrogenase, and aldehyde oxidase. Reduction in sulfite oxidase activity causes an accumulation of sulfite in the brain which is toxic to its function. This disease may be caused by mutations in the genes MOCS1, MOCS2, or gephyrin (GEPH) (22). The EEG shows multifocal spikes with or without a burst-suppression. Burst-suppression may evolve in the second day of life and persist despite treatment with anticonvulsant medications (23).
Peroxisomal Disorders
Disorders of the peroxisome have been divided into three categories: disorders of peroxisomal biogenesis (Zellweger’s syndrome, neonatal adrenoleukodystrophy [NALD], infantile Refsum’s disease), disorders of a single peroxisomal enzyme (X-linked adrenoleukodystrophy, acyl–coenzyme A [CoA] oxidase deficiency [AOXD]), and disorders with deficiencies of multiple peroxisomal enzymes (rhizomelic chondrodysplasia punctata). The discussion in this chapter is limited to Zellweger’s syndrome, AOXD, and NALD.
Zellweger’s Syndrome
Zellweger’s syndrome is the most frequent peroxisomal disorder in early infancy. It is caused by mutations in any of several genes involved in peroxisome biogenesis and results in severe brain dysfunction, craniofacial deformities, liver disease, and absence of peroxisomes.
Dysmorphic features may be noted shortly after birth. Within the first week, the affected child develops a severe encephalopathy, hypotonia, and hyporeflexia. Findings on neuroimaging and pathological examination are distinctive, with pachygyria or polymicrogyria localized to the opercular region and cerebellar heterotopias. Other pathogenic mechanisms may apply, including mitochondrial dysfunction, protein misfolding, abnormal cell signaling, and inflammation (24).
Patients with Zellweger’s syndrome have focal motor seizures of the arms, legs, or face. Their seizures do not usually culminate in generalized seizures. Myoclonic seizures and spasms have also been described. Interictal EEGs of patients with Zellweger’s syndrome showed infrequent bilateral independent multifocal spikes, predominantly in the frontal motor cortex and its surrounding regions (25). Less frequently, hypsarrhythmia is observed. Some have noted prolonged runs of vertex negative spikes (26,27).
Neonatal Adrenoleukodystrophy (NALD)
NALD, an autosomal recessive disorder with pathological and biochemical findings resembling those of X-linked adrenoleukodystrophy, bears some similarity to Zellweger’s syndrome but differs in some respects, including the ability to synthesize plasmalogens in NALD (28). Distinguishing features include absent or minimal facial dysmorphisms, later onset of seizures, absent or minimal cerebral malformations, and longer life span. Described seizures include tonic, clonic, myoclonic, or epileptic spasms (29). No characteristic EEG pattern has been defined, but descriptions have included high-voltage slowing, polymorphic delta activity, multifocal paroxysmal discharges, burst-suppression, and hypsarrhythmia.
Acyl-Coenzyme A Oxidase Deficiency (AOXD)
AOXD was first described in two siblings in 1988 (30). Clinical features include hypotonia, pigmentary retinopathy, hearing loss, developmental delay, adrenocortical insufficiency, absence of dysmorphic features, and onset of seizures shortly after birth. Serum levels of very long chain fatty acids are elevated, with normal levels of pipecolic acid. Cortical malformations are generally absent, and the interictal EEG may show continuous, diffuse, high-voltage theta activity (25).
Urea Cycle Disorders
There are six enzymes in the urea cycle that convert ammonia to urea; five of these may have defects that cause neonatal seizures. The associated diseases and their incidences are as follows: carbamoyl phosphate synthetase deficiency, 1 in 800,000; ornithine transcarbamylase deficiency, 1 in 80,000; argininosuccinate synthetase deficiency, 1 in 250,000; argininosuccinate lyase deficiency, 1 in 70,000; and N-acetylglutamate synthetase deficiency, with only a few cases reported. The clinical disorder resulting from a deficiency in the sixth enzyme, arginase, manifests later in infancy. All of these disorders are autosomal recessive except for ornithine transcarbamylase deficiency, which is X-linked dominant.
The clinical manifestations are similar and result, at least in part, from ammonia elevations. Typically, affected newborns exhibit poor feeding, emesis, hyperventilation, lethargy, or convulsions 1 to 5 days after birth. These signs give way to deepening coma with decorticate and decerebrate posturing and progressive loss of brainstem function. Brain imaging and pathological studies reveal cerebral edema with pronounced astrocytic swelling. Ammonium is taken up by the astrocytes and rapidly converted to glutamine in an energy-dependent process. The edema is associated with glutamine accumulation and energy depletion. In experimental models, blocking glutamine synthesis has prevented cerebral edema associated with hyperammonemia (31). Unfortunately, there does not appear to have been significant impact on the clinical outcome of these disorders in the past three decades (32).
The EEG shows a low-voltage pattern with diffuse slowing and multifocal epileptiform discharges and sometimes sustained monorhythmic theta activity (33). In acute neonatal citrullinemia, a burst-suppression pattern has been described, correlating with the degree of hyperammonemia (34).
Maple Syrup Urine Disease
Menkes first reported maple syrup urine disease in 1954 (35). This disease results from a defect in the branched-chain α-ketoacid dehydrogenase complex, and leads to accumulation of the branched-chain amino acids (valine, leucine, isoleucine) and their keto acids in the body tissues and fluids. Pathological studies have revealed diffuse myelin loss and increased total brain lipid content. Cystic degeneration of the white matter associated with gliosis is seen. There is speculation that DNA damage may be caused by oxidative stress (36). Disordered neuronal migration may occur with heterotopias and disrupted cortical lamination.
Feeding difficulties and lethargy are observed during the first to second weeks after birth. If left untreated, symptoms may progress to stupor, apnea, opisthotonus, myoclonic jerks, and partial and generalized seizures. A characteristic odor can be detected in urine and cerumen, but this may not be detectable until several weeks after birth. Liver transplantation by itself does not completely protect against the metabolic consequences of the disease (37). Dietary treatment consists of restriction of the branched-chain amino acids.
The EEG shows diffuse slowing and a loss of reactivity to auditory stimuli. A distinctive comb-like rhythm has been described with excessive bursts of a centrally predominant mu-like rhythm, which resolve with dietary therapy (38–39).
Organic Acidurias
The disorders of organic acid metabolism comprise a large number of inborn errors, including isovaleric aciduria and several ketotic hyperglycinemic syndromes (propionic acidemia, methylmalonic acidemia, and α ketothiolase deficiency).
Symptoms of isovaleric aciduria, which develop during the neonatal period in half of affected children, include poor feeding, vomiting, dehydration, and a progressive encephalopathy manifested by lethargy, tremors, seizures, and coma. Platelet and leukocyte counts may be depressed, and the odor of the urine has been described as similar to that of sweaty feet (13). Cerebral edema is present, and seizures are most often focal motor or generalized tonic. The initial EEG may be normal, but later studies may show deterioration, including dysmature features during sleep (40).
The symptoms of propionic acidemia also appear during the neonatal period, with seizures as the first of five symptoms. Characteristic features include vomiting, lethargy, ketosis, neutropenia, periodic thrombocytopenia, hypogammaglobulinemia, developmental retardation, and intolerance to protein. Patients may have very puffy cheeks and an exaggerated Cupid’s bow–shaped upper lip. Convulsions are the rule, although more limited focal seizures have also been reported. The EEG shows background disorganization with marked frontotemporal and occipital slow wave activity (40). In 40% of affected children, myoclonic seizures develop in later infancy, and older children may have atypical absence seizures.
Methylmalonic acidemia is the metabolic signature of seven biochemically distinct entities, all of which show decreased activity of methyl-malonyl-CoA mutase. Stomatitis, glossitis, developmental delay, failure to thrive, and seizures are the major features. Lesions of the globus pallidus, visible on computed tomography or magnetic resonance images, are characteristic. Diffuse tonic seizures and focal seizures with secondary generalization are the most frequent seizure types. Seizures may also be characterized by eyelid clonus with simultaneous upward deviation of the eyes. As in many other epileptogenic encephalopathies, the described EEG findings are multifocal spike discharges, depressed or slowed background activity, and sometimes lack of sleep spindles (40). A review of the impact of age upon the outcome in organic acidurias found evidence that newborn screening improved outcome in glutaric aciduria type 1 and methylmalonic aciduria (41).
Pyruvate Dehydrogenase Deficiency, Pyruvate Carboxylase Deficiency, and Leigh’s Syndrome
Pyruvate dehydrogenase complex (PDC) is a multienzyme conglomeration of six subunits: pyruvate dehydrogenase (E1), dihydrolipoyl acetyl transferase (E2), dihydrolipoyl dehydrogenase (E3), an E3-binding protein (E3BP), pyruvate dehydrogenase kinase (PDK), and pyruvate dehydrogenase phosphatase (PDP) [5,6]. The latter two components serve regulatory functions. A majority of the mutations affect the E1a subunit of the PDC, which impairs either stability or enzyme efficiency. Epilepsy may be due to a combination of structural abnormalities and energy failure, leading to elastic lesions (42–43).
There are various clinical manifestations of pyruvate dehydrogenase deficiency, ranging from acute lactic acidosis in infancy with severe neurologic impairment to a slowly progressive neurodegenerative disorder (43,44). It is one of the most commonly defined genetic defects of mitochondrial energy metabolism. Structural abnormalities, such as agenesis of the corpus callosum, can be visible on neuroimaging. Seizures frequently occur and may take the form of infantile spasms and myoclonic seizures. In addition to hypsarrhythmia, reported electrographic findings include multifocal slow spike-and-wave discharges. Treatment with the ketogenic diet helps with the paroxysmal aspects of the disorder and may benefit from individualized adjustment to optimize glucose intake without producing elevated lactate levels (45).
Three clinical types of pyruvate carboxylase deficiency are recognized. The neonatal type manifests with severe lactic acidemia, hepatomegaly, pyramidal tract signs, and death in the first few months of life. The infantile form is usually lethal in infancy or early childhood and begins in the first 6 months of life with episodes of lactic acidemia precipitated by an infection. Individuals with the intermittent form have normal or mildly delayed neurologic development and episodic metabolic acidosis. Seizures in the more severe forms are related to the hypoglycemia that occurs secondary to dysfunction of the Kreb’s cycle. Hypsarrhythmia, with or without infantile spasms, may be seen (46). Treatment with ACTH or the ketogenic diet can be very hazardous (47,48).
Leigh’s syndrome (subacute necrotizing encephalomyelopathy) may be caused by various metabolic defects, including cytochrome c oxidase deficiency and defects in other enzymes involved in energy metabolism. In the discussion section of his case presentation, Leigh noted that similar pathological changes could be seen in immature animals that were completely deprived of nourishment (49). Mutations in various mitochondrial respiratory chain complexes (I, II, and V) as well as any of the three catalytic subunits (E1, E2, or E3) of the pyruvate dehydrogenase complex may result in the same phenotype (50). Within the first year of life, classic features include failure to thrive, lactic acidosis, and developmental delay. As the disease progresses, infants develop spasticity, abnormalities of eye movements, and central respiratory failure. A variety of seizures may occur in Leigh’s syndrome, including focal and generalized (51). Cases of infantile spasms and hypsarrhythmia have also been reported (52), and several cases of epilepsia partialis continua (53) have also been reported. As is the case with the vast majority of epileptogenic encephalopathies associated with IEMs, EEG abnormalities, although present, do not appear to be distinctive enough to allow identification of the syndrome based on electrographic criteria alone (54).
Disorders of Biotin Metabolism
Early-Onset Multiple Carboxylase Deficiency (Holocarboxylase Synthetase Deficiency)
This rare but treatable disorder manifests in the first week after birth with lethargy, respiratory abnormalities, irritability, poor feeding, and emesis. A rash is present in more than 50% of patients. Generalized tonic convulsions, focal motor seizures, and multifocal myoclonic jerks develop in 25% to 50% of cases. A deficiency in the enzyme holocarboxylase synthetase leads to a decrease in holocarboxylase. Because this enzyme links biotin to four carboxylases in the mitochondria and one in the cytosol, an inactivity of all carboxylases results. Most patients have a good clinical response to 10 to 20 mg of biotin per day (55). Common imaging findings include subependymal cysts, ventriculomegaly, and intraventricular hemorrhage (56). Electrographically, either multifocal spikes or a burst-suppression pattern is seen (57).
METABOLIC DISORDERS OF EARLY INFANCY
The epilepsy syndrome most closely associated with IEM in infancy is West syndrome (WS). The classic triad of WS is infantile spasms, developmental delay, and hypsarhythmia. A large variety of different factors can cause WS, including IEMs. The most commonly observed are pyruvate dehydrogenase deficiency, pyruvate carboxylase deficiency, congenital disorder of glycosylation type III, organic and amino acidurias, peroxisomal disorders, and lysosomal disorders.
Some infants have forms of epilepsy that do not yet fit into one of the recognized syndromes. Those infants with unexplained, poorly controlled seizures and encephalopathy should be evaluated for IEMs. Some additional causes of infantile seizures are GM1 and GM2 gangliosidoses, neuronal ceroid lipofuscinosis, infantile neuroaxonal dystrophy, Glut-1 deficiency, late-onset multiple carboxylase deficiency, disorders of cerebral and peripheral folate metabolism, disorders of neurotransmitter synthesis, arginase deficiency (urea cycle defect), amino and organic acidurias, and sialidoses.
Lysosomal Disorders
Tay-Sachs Disease
GM2 gangliosidosis is a lysosomal disorder that invariably includes seizures as a prominent feature. The infantile form of GM2 gangliosidosis includes Tay–Sachs disease, caused by deficiency of hexosaminidase A, and Sandhoff’ s disease, caused by deficiency of hexosaminidases A and B.
Tay–Sachs disease, an autosomal recessive disorder, is found in the Ashkenazi Jewish population of Eastern or Central European descent. The enzymatic defect leads to intraneuronal accumulation of GM2 ganglioside. Development is normal until the age of 4 to 6 months, when hypotonia and loss of motor skills occur. Within the next 1 to 2 years, spasticity, blindness, and macrocephaly develop. The classic cherry-red spot is present in the ocular fundi of more than 90% of patients. At this stage, frequent focal and atypical absence seizures develop that respond poorly to medications. Myoclonic jerks are frequent and are often triggered by the exaggerated startle response to noise. The EEG is normal early in the course of the disease. Gradually, background activity slows, with bursts of high-voltage delta waves and very fast central spikes (58). Diffuse spike-and-sharp-wave activity can be abundant, and acoustically induced myoclonic seizures appear. As the disease progresses, EEG voltage declines, and background activity becomes undifferentiated.
Krabbe’s Disease (Globoid-Cell Leukodystrophy)
Another lysosomal disorder occurring at this age is globoid-cell leukodystrophy (Krabbe’s disease). This disorder is caused by a deficient activity of galactosylceramidase. There are four forms of galactosylceramidase: infantile, with onset before the age of 6 months; late infantile, with onset between the ages of 6 months and 3 years; juvenile, with onset between the ages of 3 and 7 years; and adult, with onset after the age of 7 years (59). The majority of cases begin within 3 to 6 months of life with irritability, poor feeding, emesis, and rigidity. Muscular spasms induced by stimulation are prominent. Blindness and optic atrophy ensue. Initially, tendon reflexes are increased; then they gradually diminish as peripheral myelin breaks down. Focal or generalized clonic or tonic seizures and infantile spasms are seen; they may be difficult to distinguish from muscular spasms (60,61). In contrast to what is observed in many classic white-matter diseases, seizures occur early in the course of 50% to 75% of infants with Krabbe’s disease. EEG characteristics include a hypsarrhythmia-like pattern with irregular slow activity and multifocal discharges of lower amplitude than that typically seen with West syndrome (62). In a study of seven infants by Kliemann et al in 1969, six children had prominent beta activity independently occurring in the posterior temporal regions and vertex that was superimposed over slower, high-amplitude waves. This activity was observed to be state-dependent and to occur in long runs without any observed clinical manifestations. In the terminal stages of the disease, little electrical activity is detected. Hematopoietic stem cell transplantation is an available option for presymptomatic patients.
GM1 Gangliosidosis, Type I and Type II
The infantile form of this disorder, type I, is caused by a deficiency of β-galactosidase activity that leads to the accumulation of GM1 ganglioside and degradation products in nerve cells and other tissues. Regression of development begins at 3 to 6 months of age with rapid neurological deterioration, and seizures develop by 2 years of age. Clinical features may include coarse facial features, hepatomegaly, bone deformities (dysostosis multiplex), visual abnormalities, hypotonia, progressive microcephaly, and hematologic abnormalities. Examination of the ocular fundi reveals a macular cherry-red spot. A late infantile/juvenile form is recognized, as it presents with cognitive delay between 7 months and 3 years of age with slow progression.
Neurologic deterioration in the adult form is generally slower than in the others and presents with a progressive extrapyramidal disorder. In an illustrative example of the benefits of whole exome sequencing, an individual who had escaped diagnosis for more than a decade was diagnosed by this technique (63).
Cerebral manifestations, with regression of developmental milestones and visual symptoms, typically manifest by the age of 2 to 4 years. EEG features include background slowing with increasing, irregular slow activity as the disease progresses (64). In older patients, a fluctuating 4- to 5-Hz temporal rhythmic discharge has been observed.
Disorders of Vitamin Metabolism
Biotinidase Deficiency
Disorders of vitamin metabolism with symptoms that appear in early infancy include biotinidase deficiency and disorders of folic acid. Biotinidase deficiency is the second disorder that can produce multiple carboxylase deficiency. Early-onset multiple carboxylase deficiency due to holocarboxylase synthase deficiency is the other disorder, discussed earlier.
Late-Onset Multiple Carboxylase Deficiency (Biotinidase Deficiency)
Seizures are a prominent feature of late-onset multiple carboxylase deficiency, occurring in approximately 75% of affected children, and are the presenting clinical symptoms in one-third of cases. There is a distinctive organic acid pattern, reflective of the various carboxylase deficiencies. The onset of the disease process begins at the age of 3 to 6 months with hypotonia and psychomotor delay. Seborrheic or atopic dermatitis and alopecia are common. As the disease progresses, intermittent ataxia, optic atrophy, and sensorineural hearing loss develop. Associated seizure types include generalized tonic–clonic, partial, myoclonic, and infantile spasms (65). EEG findings may include a burst-suppression pattern, absence of physiological sleep patterns, poorly organized and slow waking background activity, and frequent multifocal spike and diffuse spike-and-slow-wave discharges (65). Screening newborns for this disease is important because high-dose biotin initiated at birth or before symptoms develop can essentially prevent all the symptoms of this disorder (66).
Methylene Tetrahydrofolate Reductase Deficiency
Methylene tetrahydrofolate reductase deficiency is the most common inborn error of folate metabolism. This causes insufficient production of 5-methyltetrahydrofolate, which is needed for the remethylation of homocysteine to methionine. As a result, there is an accumulation of homocysteine and hypomethionine in the blood and body fluids. A progressive neurologic syndrome can develop in infancy with acquired microcephaly, hypotonia, lethargy, recurrent apneas, and seizures. The latter are characterized by intractable infantile spasms, generalized atonic and myoclonic seizures, and focal motor features. EEG findings vary from diffuse slowing of background activity to continuous spike-and-wave complexes or multifocal spikes to paroxysmal bursts of generalized polyspikes followed by periods of relative attenuation with a pseudoperiodic pattern (67). A meta-analysis of reports on 36 patients suggested improved survival and psychomotor development with early betaine treatment (68).
Defects in methionine biosynthesis are also associated with seizures. Convulsions are frequent and are predominantly generalized, although myoclonic seizures with hypsarrhythmia have been reported.
Glucose Transporter 1 Deficiency Syndrome
The glucose transporter 1 (Glut-1) deficiency syndrome, previously referred to as the glucose transporter protein deficiency syndrome, was first described in 1991 (69,70). This may be considered the prototype disorder of energy failure producing seizures and EEG abnormalities. Affected infants become encephalopathic with seizures and delays of motor and mental development. Seizures are present in 90% of patients and typically begin after the second month of life. Initially, the presenting symptoms are paroxysmal with seizures, eye movement abnormalities, or subtle behavioral alterations consistent with infantile focal seizures. The initial EEG may be normal or may show interictal epileptiform discharges in the posterior quadrants, but only 17% show persistently normal features (71). As the child matures, more generalized seizures occur, manifested by nonconvulsive events, myoclonic jerks, or atypical absence seizures. A recent review found that, generalized tonic–clonic seizures and absence attacks predominate and are seen in about half the patients. These same patients may exhibit 3-Hz spike-and-wave discharges that look very similar to those seen in childhood absence epilepsy (pyknolepsy) (72). On a careful inspection of the individual spike components, however, one may note some variability in the morphology and amplitude that, in addition to a mild degree of background slowing, may be important clues to the presence of symptomatic epilepsy. It is interesting to reflect on the early observations of Gibbs, Gibbs, and Lennox in this context (73). They showed a strong influence of blood sugar levels on absence seizures and bursts of 3-Hz spike–wave discharges. In Glut1 DS, acute hyperglycemia can produce a transient improvement in the background EEG features and abundance of epileptiform discharges, so pre- and postprandial tracings might be suggestive of the condition (74,75). Other observed seizures include focal, myoclonic, drop, tonic, and very rarely infantile spasms (71). As is seen in most of the IEMs associated with epilepsy, the EEG features in patients with Glut1 DS may change over time in a given individual (71). Early treatment with the ketogenic diet is indicated.
Organic Acidurias
Seizures in early infancy may be the presenting symptom of branched-chain organic acidurias. These include isovaleric aciduria, 3-methylcrotonyl CoA carboxylase deficiency, 3-methylglutaconic aciduria with normal 3-methylglutaconyl-CoA hydratase, and 3-hydroxy-3-methylglutaric aciduria. Seizures, including convulsions and infantile spasms, tend to be prominent in 3-methylcrotonyl CoA carboxylase deficiency.
Severe developmental delay, progressive encephalopathy, and seizures are the most common features of 3-methylglutaconic aciduria with normal 3-methylglutaconyl-CoA hydratase (76). Seizures occur in one-third of the cases, and infantile spasms have been reported early on. In another study, eight patients with this disorder were studied, and the most typical finding was mild to moderate slowing of the EEG background. One patient had multifocal sharp-wave discharges as well (40).
Seizures are the presenting symptom in 10% of patients with 3-hydroxy-3-methylglutaric aciduria, a disorder caused by a deficiency of the lyase enzyme that mediates the final step of leucine degradation and plays a pivotal role in hepatic ketone body production. This disorder is one of an increasing list of IEMs that clinically present as Reye’s syndrome or nonketotic hypoglycinemia. The odor of the urine may resemble that of a cat. In many affected patients, EEGs are normal between crises. EEGs in one girl with progressive encephalopathy showed multifocal spikes and intermittent episodes of background attenuation between crises, and focal epileptiform activity during an episode of clinical deterioration (40).
Infantile spasms have been reported in patients with 3-hydroxybutyric aciduria. Facial dysmorphism and brain dysgenesis are prominent manifestations.
Type I glutaric acidemia is a more common autosomal recessive disorder of lysine metabolism that is caused by a deficiency of glutaryl-CoA dehydrogenase. Seizures are often the first clinical signs of metabolic decompensation after a febrile illness. EEGs are initially normal, but slight background slowing may develop during times of seizure exacerbation (40).
Aminoacidurias
Phenylketonuria and Hyperphenylalaninemias
One of the most frequent IEMs, occurring in 1 in 10,000 to 15,000 live births, phenylketonuria is caused by a deficiency of the hepatic phenylalanine hydroxylase. As a consequence of the metabolic defect, toxic levels of the essential amino acid phenylalanine accumulate. If these toxic levels are left untreated, severe mental retardation, behavioral disturbances, psychosis, and acquired microcephaly can result. Seizures are present in 25% of affected children. The majority of children with phenylketonuria (80%–95%) are found to have abnormalities on EEG. An age-related distribution of EEG findings and seizure types has been observed. Low et al (77) described characteristic features in 1957. Infantile spasms and hypsarrhythmia predominate in the young affected infant. As the children mature, tonic-clonic and myoclonic seizures become more frequent, and the EEG pattern evolves to mild diffuse background slowing, focal sharp waves, and irregular generalized spike-and-slow waves (78). An increase in delta activity has been seen as levels increased during phenylalanine loading (79).
Tyrosinemia, Type III
An inborn error of tyrosine metabolism, type III tyrosinemia (4-hydroxyphenylpyruvate dioxygenase deficiency), has been reported in a newborn with intractable seizures and in children who later developed infantile spasms (80). The EEG pattern has been described as low voltage with spike and polyspike discharges in the parietal–occipital regions.
Menkes Disease (Kinky Hair Disease)
This X-linked disorder of copper absorption due to mutations in ATP7A was first described by Menkes et al in 1962 (81). A feature of this disorder is the “kinky hair” of the head and eyebrows. A characteristic twisting of the hair shaft is noted on microscopic examination of these poorly pigmented hairs. Affected boys may be premature, may have neonatal hyperbilirubinemia, or may have hypothermia. Progressive neurologic deterioration with spasticity manifests by the age of 3 months, and affected children may have associated bone and urinary tract abnormalities as well. The disease has a rapidly fatal course. Even in utero injections of copper histidine have been unsuccessful in curing the disorder (82).
Seizures, in the form of intractable generalized or focal convulsions, are a prominent feature in Menkes disease. Stimulation-induced myoclonic jerks are also present. Multifocal spike-and-slow-wave activity can be seen on EEG, sometimes resembling hypsarrhythmia (83).
Progressive Encephalopathy With Edema, Hypsarrhythmia, and Optic Atrophy (PEHO Syndrome)
PEHO syndrome, described by Salonen et al in 1991, is characterized by infantile spasms, arrest of psychomotor development, hypotonia, hypsarrhythmia, edema, and visual failure with optic atrophy (84). Characteristic features include epicanthal folds, midfacial hypoplasia, protruding ears, gingival hypertrophy, micrognathia, and tapering fingers. Edema develops over the limbs and face. The progressive decline seen with this disease originally suggested a metabolic defect, although no biochemical marker was identified. It was presumed to be an autosomal recessive disorder by its pattern of inheritance, but a recent report suggests that some cases may be due to a de novo disease-causing variant in KIF1A impacting the motor domain of the protein (85). This protein is involved in the anterograde transport of synaptic-vesicle precursors along axons. Neuroimaging shows progressive brain atrophy and abnormal myelination. Hypoplasia of the corpus callosum has been reported. Seizures generally begin as infantile spasms with associated hypsarrhythmia on the EEG. Later, other seizure types may be seen, including tonic, tonic–clonic, and absence seizures. The EEG pattern may evolve to a slow spike-and-wave pattern.
METABOLIC DISORDERS OF LATE INFANCY
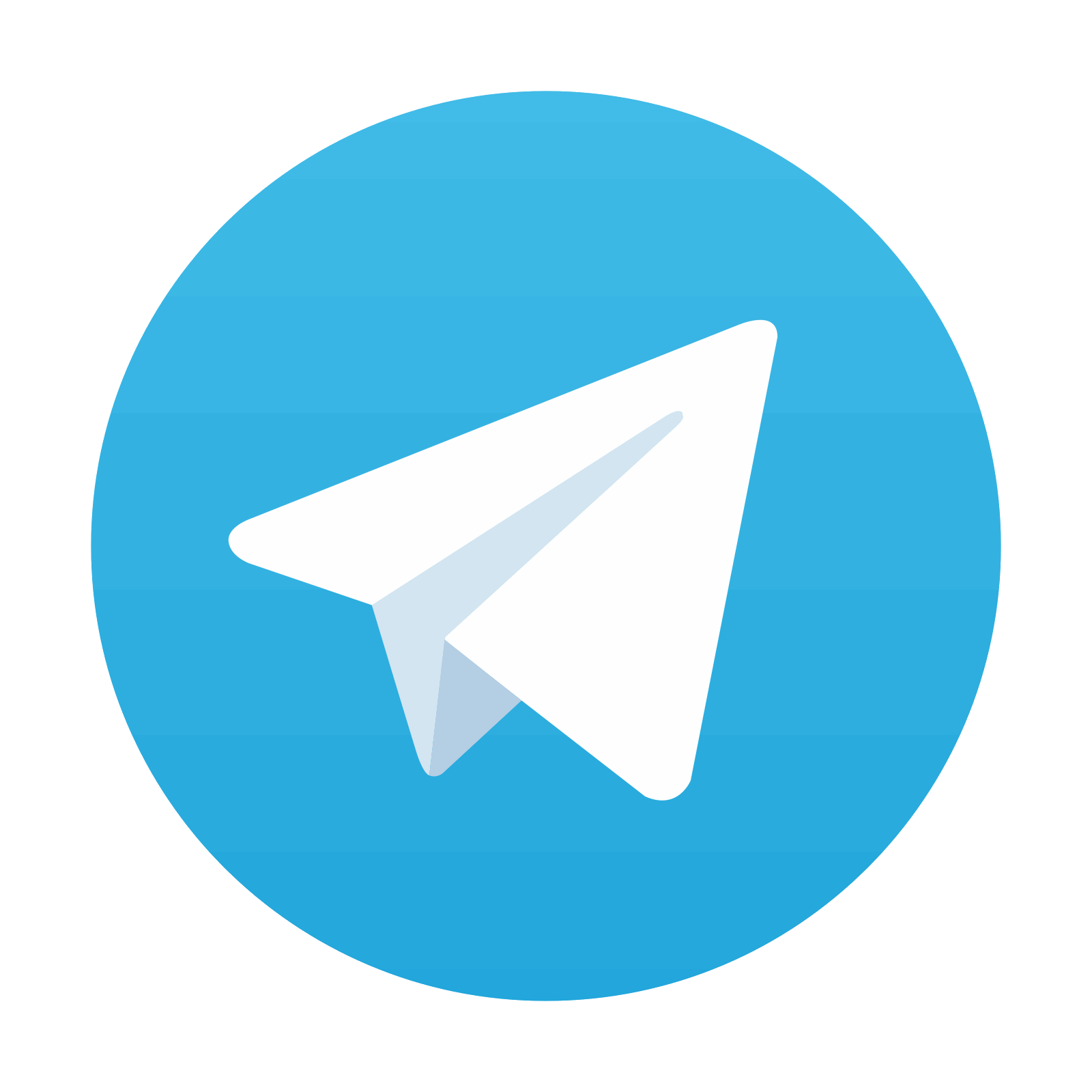
Stay updated, free articles. Join our Telegram channel

Full access? Get Clinical Tree
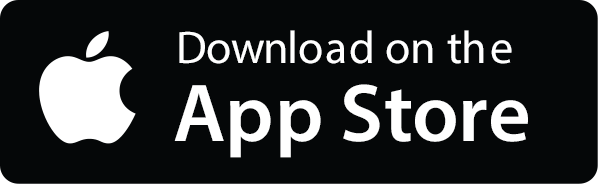
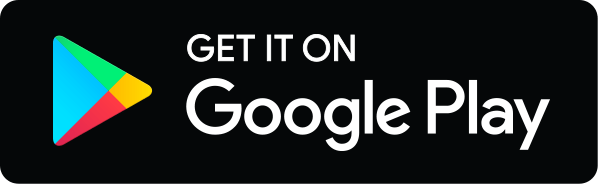