Drugs and Perinatal Brain Injury
Maria Delivoria-Papadopoulos
Agustín Legido
Ignacio Valencia
Om Prakash Mishra
Severe fetal asphyxia may have a broad range of effects on the brain from no evident alteration in brain function to profound cerebral palsy. A large amount of information has been collected on the fetal cardiovascular and respiratory response to oxygen limitations, giving rise to a better understanding and management of neonatal deterioration induced by asphyxia. Besides these physiologic studies, cellular and biochemical mechanisms that result in brain cell death are being increasingly explored, particularly in the adult with respect to focal (stroke) and global (cardiac arrest) hypoxia–ischemia (1). These studies have shown that complex and interrelated biochemical alterations are triggered during hypoxia–ischemia in mature subjects that ultimately result in neuronal death. Studies are in progress to investigate mechanisms of hypoxic brain injury in the fetus and newborn brain (2,3,4,5,6). A thorough knowledge of these biochemical events may lead to recognition of certain steps that may be amenable to pharmacologic intervention to limit or even prevent neuronal cell damage during fetal asphyxia.
Hypoxic injury in the fetal and newborn brain results in neonatal morbidity and mortality as well as long-term sequelae such as mental retardation, seizure disorders, and cerebral palsy (2,7). Although the consequences of antepartum or perinatal hypoxia can be observed in babies, the specific mechanisms of pathologic processes preceding the onset of cerebral dysfunction are not well understood. To focus on the cellular and molecular mechanisms of hypoxic injury in the developing brain, it is important to recognize the factors that may determine the susceptibility of the developing brain to prenatal and perinatal hypoxia.
The determinants of the susceptibility of the developing brain to hypoxia include the lipid composition of the brain cell membrane, the rate of lipid peroxidation, the presence of antioxidant defenses, the development and modulation of the excitatory neurotransmitter receptors such as the N-methyl-D-aspartate (NMDA) receptor, and the intracellular Ca2+ and intranuclear Ca2+ influx mechanisms. In addition to the developmental status of these cellular components, the response of these potential mechanisms to hypoxia determines the fate of the hypoxic brain cell in the developing brain in the fetus and the newborn. Elucidating basic cellular mechanisms in response to hypoxia of the developing brain will enable the development of novel strategies for preventing or attenuating the deleterious effects of hypoxia in the human newborn. Several excellent reviews on different aspects of hypoxic/ischemic cell injury in the developing brain have been published recently (4,8,9,10,11).
Perinatal hypoxic ischemia is the most common cause of neurologic disease during the neonatal period [hypoxic–ischemic encephalopathy (HIE)], and is associated with a high mortality and morbidity rate, including cerebral palsy, mental retardation, and seizures. The incidence of perinatal asphyxia is about 1.0% to 1.5% in most centers and is usually related to gestational age and birth weight. It occurs in 9.0% of infants less than 36 weeks’ gestation and in 0.5% of infants more than 36 weeks’ gestation (12,13,14). The etiology of perinatal HIE includes those circumstances that can affect the cerebral blood flow in the fetus and newborn compromising the supply of oxygen to the brain. They may develop antepartum (20%), intrapartum (30%), intrapartum and antepartum (35%), or postpartum (10%) (1).
HIE develops in the setting of perinatal asphyxia, which is a multiorgan system disease (12,13,14,15,16). Assessment and management of these complications is an integral part of the treatment of perinatal asphyxia/HIE (12,13,14,15).
This chapter primarily focuses on the neuroprotective agents that have suggested to be effective in human clinical studies, and highlights alternative approaches for the treatment of HIE in the future.
Neuroprotective Treatments with Suggested Efficacy in Humans
Magnesium Sulfate
Magnesium is a naturally occurring NMDA receptor antagonist that blocks the neuronal influx of Ca2+ within the receptor ion channel (3,4,17,18). Experimental studies in newborn animals have shown a neuroprotective effect of magnesium sulfate (MgSO4). MgSO4 reduced brain injury in 7-day-old rats when administered 15 minutes after cerebral injection of NMDA (19). MgSO4 administration in
newborn piglets prevented hypoxia-induced modification of the NMDA receptor as well as alteration of neuronal membrane structure and function (20). In addition, MgSO4 administration in pregnant guinea pigs prior to hypoxia prevented the hypoxia-induced generation of oxygen free radicals, cell membrane peroxidation, and nuclear DNA fragmentation (21,22). MgSO4 also decreased excitotoxic brain damage in 50-day-old mice when given before or 10 minutes after an injection of ibotenate, a glutamatergic agonist (23). However, in fetal lambs and piglets subjected to hypoxia and/or ischemia, MgSO4 failed to prevent cerebral injury (24,25).
newborn piglets prevented hypoxia-induced modification of the NMDA receptor as well as alteration of neuronal membrane structure and function (20). In addition, MgSO4 administration in pregnant guinea pigs prior to hypoxia prevented the hypoxia-induced generation of oxygen free radicals, cell membrane peroxidation, and nuclear DNA fragmentation (21,22). MgSO4 also decreased excitotoxic brain damage in 50-day-old mice when given before or 10 minutes after an injection of ibotenate, a glutamatergic agonist (23). However, in fetal lambs and piglets subjected to hypoxia and/or ischemia, MgSO4 failed to prevent cerebral injury (24,25).
In the clinical setting, MgSO4 has been widely used in obstetrics practice for more than 60 years. Its indications include suppression of preterm labor and management of pregnancy-induced hypertension (26). A retrospective epidemiologic study by Nelson and Grether (27) suggested that premature fetuses whose mothers received MgSO4 for the treatment of preeclampsia or as a tocolytic agent are less likely to develop cerebral palsy compared with a gestational age-matched group of fetuses not exposed to the drug. The Collaborative Eclampsia Trial (28) reported that babies of women who had been given MgSO4 before delivery were significantly less likely to be intubated at the place of delivery or to be admitted to a special care nursery than the babies of mothers who had been given phenytoin. These studies suggested that MgSO4 might provide a protective effect against brain damage in immature fetuses and newborn infants. Randomized, controlled, double-blind trials were established to examine this hypothesis. One was discontinued after interim analysis showed that administration of MgSO4 to mothers in preterm labor before 34 weeks of gestation was associated with significant increase in infants’ mortality (29). However, other trials have not shown any difference in the mortality between the placebo and treatment groups (30).
Recent studies have been statistically more powerful. A multicenter randomized controlled trial of MgSO4 versus placebo, for the prevention of cerebral palsy, in 2,241 women at risk of imminent premature delivery at 24 to 31 weeks of gestation carried out in the United States was published in 2008 (31). The primary outcome was the composite of stillbirth or infant death by 1 year of corrected age or moderate or severe cerebral palsy at or beyond 2 years of corrected age. The primary outcome was not significantly different in the MgSO4 group and the placebo group. However, in a prespecified secondary analysis, moderate or severe cerebral palsy occurred significantly less frequently in the MgSO4 group (1.9% vs. 3.5%). A similar study in France followed up 606 infants less than 33 weeks of gestation, whose mothers were treated with MgSO4. Compared to placebo, treated infants showed a decrease of all primary end points (total mortality, severe white matter injury, and their combined outcome) and of all secondary end points (motor dysfunction, cerebral palsy, cognitive dysfunction, and their combined outcomes at 2 years of age). The decrease was nearly significant or significant for gross motor dysfunction and combined criteria: death and cerebral palsy, death and gross motor dysfunction, death, cerebral palsy, and cognitive dysfunction (32). A recent paper by Doyle et al. (33) reviewed the evidence of the neuroprotective effects of MgSO4 given to women considered at risk of preterm birth. The authors concluded that the neuroprotective role for antenatal MgSO4 therapy given to mothers at such risk is now established. The number of women needed to treat to benefit one baby by avoiding cerebral palsy is 63 (95% confidence interval 43 to 87). Given the beneficial effects of MgSO4 on substantial gross motor function in early childhood, outcomes later in childhood should be evaluated to determine the presence or absence of later potentially important neurologic effects, particularly on motor or cognitive function.
Allopurinol
Allopurinol, an inhibitor of the enzyme xanthine oxidase, blocks the synthesis of xanthine from hypoxanthine and therefore prevents the formation of the free radical superoxide. Ischemia–reperfusion results in injury to organs containing the highest amounts of xanthine oxidase, such as the liver and the intestine. The injury results in the release of xanthine oxidase into the circulation. Circulating xanthine oxidase in adults causes damage to remote nonischemic organs that possess low amounts of endogenous xanthine oxidase, such as the heart and the brain. It is not known whether allopurinol inhibits circulating xanthine oxidase or endogenous xanthine oxidase in the brain, or both (3,4,6,8,12).
In experimental animal models, administration of allopurinol to immature rats 30 minutes before inducing focal hypoxia–ischemia reduced the severity of the secondary edema and the extent of the neuropathologic lesion in the treated group compared with a control group (34). Similarly, pretreatment with allopurinol preserved cerebral energy metabolism of the 7-day postnatal rat during hypoxia–ischemia (35). The same group of researchers also found that oxypurinol, the active metabolite of allopurinol, administered at the same dose and at the same time as allopurinol after hypoxia–ischemia reduced brain injury in the immature rat (36). Administration of allopurinol in newborn piglets prevented the hypoxia-induced modification of NMDA receptor as well as cell membrane peroxidation and neuronal dysfunction (37,38).
In the clinical setting, a 7-day course of enteral allopurinol (20 mg per kg) given after birth to 400 infants between 24 and 32 weeks’ gestation did not change the incidence of periventricular leukomalacia (39). In a study of 22 asphyxiated newborn infants, intravenous allopurinol in a dose of 40 mg per kg given 4 hours after birth resulted in a decrease in mortality (2/11 vs. 6/11 in the control group), and in a beneficial effect on free radical formation, cerebral blood flow, and electrical brain activity, without toxic side effects (40). Clancy et al. (41) conducted a clinical trial to test the hypothesis that allopurinol could reduce death, seizures, coma, and cardiac events in infants who underwent heart surgery using deep hypothermic circulatory arrest. They studied a total of 318 infants, 131 hypoplastic left heart syndrome (HLHS) and 187 non-HLHS. In HLHS surgical survivors, 40 of 47 (85%) allopurinol-treated infants did not experience any end point event, compared with 27 of 49 (55%) controls (p = .002). There were fewer “seizure-only” (p = .05) and
“cardiac-only” (p = .03) events in the allopurinol versus placebo groups. Allopurinol did not reduce efficacy end point events in non-HLHS infants. Treated and control infants did not differ in adverse events. Recently, Benders et al. (42) investigated whether postnatal allopurinol would reduce free radical induced reperfusion/reoxygenation injury of the brain in severely asphyxiated neonates. In an interim analysis of a randomized, double-blind, placebo controlled study, 32 severely asphyxiated infants were given allopurinol or a vehicle within 4 hours of birth. The analysis showed an unaltered (high) mortality and morbidity in the infants treated with allopurinol. The authors concluded that allopurinol treatment started postnatally was too late to reduce the early reperfusion-induced free radical surge. Allopurinol administration to the fetus with (imminent) hypoxia via the mother during labor may be more effective in reducing free radical–induced postasphyxial brain damage.
“cardiac-only” (p = .03) events in the allopurinol versus placebo groups. Allopurinol did not reduce efficacy end point events in non-HLHS infants. Treated and control infants did not differ in adverse events. Recently, Benders et al. (42) investigated whether postnatal allopurinol would reduce free radical induced reperfusion/reoxygenation injury of the brain in severely asphyxiated neonates. In an interim analysis of a randomized, double-blind, placebo controlled study, 32 severely asphyxiated infants were given allopurinol or a vehicle within 4 hours of birth. The analysis showed an unaltered (high) mortality and morbidity in the infants treated with allopurinol. The authors concluded that allopurinol treatment started postnatally was too late to reduce the early reperfusion-induced free radical surge. Allopurinol administration to the fetus with (imminent) hypoxia via the mother during labor may be more effective in reducing free radical–induced postasphyxial brain damage.
Chaudhari and McGuire (43) performed a meta-analysis to evaluate the evidence of the effect of allopurinol on mortality and morbidity in newborn infants with suspected hypoxic–ischaemic encephalopathy. The authors concluded that the available data are not sufficient to determine whether allopurinol has clinically important benefits for newborn infants with hypoxic–ischaemic encephalopathy and, therefore, larger trials are needed. Such trials could assess allopurinol as an adjunct to therapeutic hypothermia in infants with moderate and severe encephalopathy and should be designed to exclude clinically important effects on mortality and adverse long-term neurodevelopmental outcomes.
Opioids
The antinociceptive effects of opioids are mediated through a combination of pre- and postsynaptic hyperpolarization, which produces a decrease in the release of and the sensitivity to endogenous mediators like glutamate (44,45). This suggests that they may have a neuroprotective effect. Indeed, studies in cell cultures have demonstrated that endogenous and exogenous opioids may protect cortical neurons from hypoxia-induced cell death (46,47). Similarly, opioids may induce ischemic tolerance in cerebellar Purkinje cells subject to ischemia-reperfusion (48). Antagonists of opioid receptors increase the survival time during severe hypoxia in intact animals (49,50) and enhance tissue preservation and survival time of organs used for transplants (51).
In 2005, Angeles et al. (52), published the results of a retrospective study of 52 term newborns with perinatal asphyxia, in which they analyzed the relationship between treatment with opioid analgesics (morphine or fentanyl) and neurological damage. A total of 33% of them received opioids; in spite of having a more severe degree of asphyxia (higher levels of lactate, lower 5-minute Apgar score), this group of patients had less severe signs of brain damage on the MRI performed after 7 days of life. Moreover, their neurologic outcome at a mean follow-up of 13 months was better than that of the group of newborns who did not receive opioids. The same group of researchers also performed a follow-up study with MR spectroscopy of 28 term newborns treated with opioids and 20 controls (53). The results showed that occipital gray matter NAA/Cr was significantly decreased and lactate was present in a significantly higher amount in non–opioid-treated neonates compared with opioid-treated neonates. Also, compared with controls, untreated neonates showed larger changes in more metabolites in basal ganglia, thalami, and occipital gray matter with greater significance than treated neonates. The authors concluded that the use of opioids during the first week following perinatal asphyxia has no long-term adverse effects and may increase brain resistance to hypoxic ischemia. The authors speculated that the neuroprotective effect of opioids may be mediated by increasing the levels of adenosine, an endogenous nucleoside with neuroprotective activity, or by inducing neuronal hyperpolarization, which results in diminishing intracellular penetration of calcium.
Despite the potential benefit of opioids on asphyxiated term neonates as indicated in these studies, caution must be exercised in the use of this class of medications. Available literature suggests that the routine use of opioid analgesics can be complicated by problems such as tolerance, withdrawal, and ventilator dependence. Very few studies have examined the long-term effects of exposure to opioids in the neonatal period. In addition, previous reports indicate that endogenous opioids can suppress DNA synthesis in vivo in immature cerebellar and glial cells (opioid receptors are widely distributed in the CNS with functions that include pain modulation, cardiorespiratory regulation), whereas exogenous opioids can exacerbate neurotoxicity in animal models of cerebral ischemia. Future prospective randomized trials are warranted to determine whether there is truly an immediate neuroprotective effect on hypoxic–ischemic brain injury and whether these agents can play a role in improving long-term outcome.
Hypothermia
Hypothermia has developed during the past few years as an alternative for treating perinatal asphyxia/HIE (54,55). Hypothermia during experimental cerebral ischemia is associated with potent dose-related, long-lasting neuroprotection. Conversely, hyperthermia of only 1°C to 2°C extends and markedly worsens damage, and in particular tends to promote pannecrosis (54). Although the majority of such studies involved global ischemia in adult rodents (56), similar results were reported from studies and hypoxia–ischemia in 7-day-old rats (57) and newborn piglets (58), kittens, rabbits, and puppies (59).
The study of the mechanisms of action of hypothermic neuroprotection suggests that cooling affects many or all of the pathways leading to delayed cell death (54). Hypothermia reduces the rate of oxygen-requiring enzymatic reactions and cerebral oxygen consumption, slows the fall of phosphocreatine/inorganic phosphate (PCr/Pi), and confers a protective effect of the brain after adenosine triphosphate (ATP) exhaustion. In addition, hypothermia decreases oxygen consumption of the brain by 6% to 7% and cerebral energy utilization rate by 5.3% per degree. Additional experimental evidence suggests that hypothermia suppresses cytotoxic excitatory amino acid accumulation, inhibits nitric oxide synthase activity,
decreases interleukin-1 levels, decreases the release of other cytotoxic cytokines by microglial cells, and suppresses free radical activity and delayed cell death by apoptosis. Hypothermia also decreases blood–brain barrier permeability and intracranial pressure and facilitates recovery of electrophysiologic function after cerebral ischemia.
decreases interleukin-1 levels, decreases the release of other cytotoxic cytokines by microglial cells, and suppresses free radical activity and delayed cell death by apoptosis. Hypothermia also decreases blood–brain barrier permeability and intracranial pressure and facilitates recovery of electrophysiologic function after cerebral ischemia.
The efficacy of hypothermia is dependent on a number of factors, such as the timing of initiation of cooling, its duration, and the depth of cooling attained (54). Mild hypothermia is defined as a reduction in core temperature of 1°C to 3°C, moderate as 4°C to 6°C, severe as 8°C to 10°C, and profound as 15°C to 20°C. Brief (0.5 to 3 hours), mild-to-moderate hypothermia immediately after hypoxia–ischemic injury may be most effective after relatively mild insults. Protection appears to be lost if brief hypothermia is delayed by as little as 15 to 45 minutes after the primary insult. A more recent approach has been to try to suppress the secondary encephalopathic processes by maintaining hypothermia throughout the course of the secondary phase. An extended period of cooling (between 5 and 72 hours) appears to be more consistently effective and remains effective after significant delays (possibly up to 6 hours) between the primary insult and the start of cooling; however, the degree of neuroprotection progressively declines if cooling is initiated more than a few hours postinsult (54). In addition, cerebral hypothermia is not neuroprotective when started after postischemic seizures occur (60).
Potential adverse effects of induced hypothermia (the risk increasing with depth of hypothermia) include increased blood viscosity, mild metabolic acidosis, cardiac arrhythmias, decreased oxygen availability, dysfunction of cellular immunity, coagulation abnormalities and platelet dysfunction, intracellular shift of potassium, and choreic syndrome (55).
The first study on neuroprotection of perinatal HIE with selective head cooling was published in 1998 by Gunn et al. (61), who basically proved the safety of this procedure. Later on, the same group of researchers published the results of other studies in a small number of patients, which confirmed the lack of side effects and a tendency to a better neurologic prognosis in those newborns with moderate or severe HIE treated with this hypothermia technique (62,63).
In 2005, Gluckman et al. (64) published the results of the most important study that has produced reliable and significant data about the neuroprotective effect of selective head cooling hypothermia. It was a multicenter investigation that included 234 newborns with HIE and estimated gestational age (EGA) above 36 weeks. HIE was defined according to the following criteria: pH less than 7.0, base excess equal to or higher than 16 mmol per L, Apgar store at 10 minutes equal to or less than 5, need for cardiorespiratory resuscitation for more than 10 minutes, abnormal neurological examination according to the Sarnat’s criteria of moderate or severe encephalopathy, and abnormal amplitude integrated electroencephalogram (EEG) (aEEG). Patients were randomized before 5.5 hours of life into two groups: body normothermia or hypothermia of 34.5°C, induced through selective head cooling during 72 hours. Patients treated with hypothermia had a significantly higher incidence of arrhythmia (mostly sinus bradycardia). A total of 218 infants were followed up until 18 months of age. The presence of death or neurological disability was found in 66% of patients in the control group and 55% in the hypothermia group (p < .1). However, when newborns with severe neurological depression or those who had seizures on the aEEG were excluded, 66% of infants in the control group and 48% in the hypothermia group died or had neurological disability (p < .02). Moreover, the presence of severe neurological disability was 28% and 12% in each group, respectively. The authors concluded that except in newborns with the most severe forms of HIE, selective head cooling applied immediately following delivery may be a feasible therapeutic technique to decrease neurological sequelae of perinatal HIE.
The first study with generalized body hypothermia in perinatal HIE was published in 2000 by Azzopardi et al. (65), who found that prolonged hypothermia of 33°C to 34°C was associated with minimal physiological changes (e.g., decreased heart rate, increased blood pressure) but was well tolerated. During the next 3 years, other research protocols in a limited number of patients corroborated that generalized body hypothermia was a feasible and clinically safe technique (66,67,68).
In 2005, Eicher et al. (69,70) published the results of a pilot multicenter study about the safety and efficacy of generalized body hypothermia in the treatment of 32 newborns with perinatal HIE. Adverse effects included bradycardia, hypotension, decreased platelets, increased prothrombin time, and higher incidence of seizures, but none of them was severe, and they all responded to treatment (69). The efficacy results showed a higher incidence of death or severe neurological motor involvement in the control group (82%) compared with the group of hypothermia-treated newborns (52%) (p = .019). A severe psychomotor developmental delay (<70%) was seen in 64% of infants in the control group and in 24% of those subjected to hypothermia (p = .053).
Also in 2005, Shankaran et al. (71) published a larger multicenter study on the use of body hypothermia to treat perinatal HIE. A total of 208 newborns with HIE, EGA more than 36 weeks, were included. HIE was defined according to the following criteria: need for neonatal resuscitation, abnormal neurologic examination compatible with moderate or severe encephalopathy, pH less than 7.0, and a base excess equal to or above 16 mmol per L. EEG criteria were not included in the diagnostic requirements. Patients were randomized before 6 hours of life into two groups: body normothermia or hypothermia of 33.5°C, induced by body cooling, during 72 hours. Patients were followed up until 18 to 22 months. The incidence of mild complications was similar in both groups. The incidence of death or moderate or severe neurological disability was 62% in the control group and 44% in the hypotermia-treated group (p = .01). The incidence of cerebral palsy was 30% in the control newborns and 19% in those treated with hypothermia (p = .20). The authors concluded that generalized body hypothermia reduces the risk of death and neurological disability in newborns with moderate or severe HIE. An MRI follow-up study of infants enrolled in the above-mentioned trial (71) aimed to measure relative volumes of subcortical white matter. They were significantly larger in hypothermia-treated than in control infants.
Furthermore, relative total brain volumes correlated significantly with death or neurosensory impairments. Relative volumes of the cortical gray and subcortical white matter also correlated significantly with Bayley scales psychomotor development index (72).
Furthermore, relative total brain volumes correlated significantly with death or neurosensory impairments. Relative volumes of the cortical gray and subcortical white matter also correlated significantly with Bayley scales psychomotor development index (72).
Hypothermia is currently in the process of translating from the clinical research experience to direct clinical application (73,74). However, the clinical trials with hypothermia have also addressed many questions, which need to be answered before it becomes the standard of care to treat newborns with perinatal asphyxia: Which should be the patients’ selection criteria? When should hypothermia be initiated? How long should hypothermia last? Which is the most effective technique? Is hypothermia a safe technique? Which is the long-term outcome? Should sedation be administered concomitantly with hypothermia? How should rewarming be done? and Could hypothermia efficacy be enhanced with simultaneously administering other neuroprotective agents? (6,8,75,76,77,78,79,80).
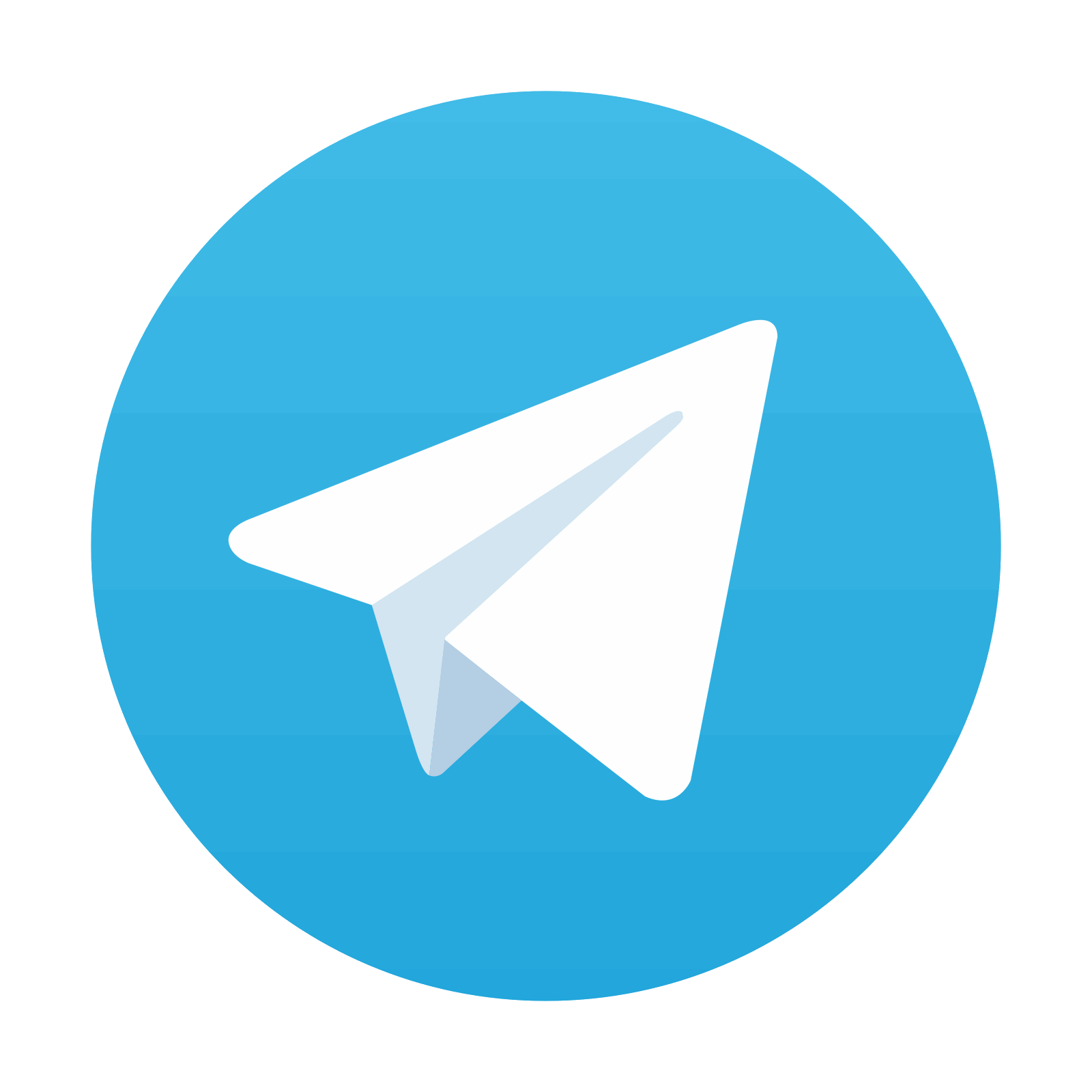
Stay updated, free articles. Join our Telegram channel

Full access? Get Clinical Tree
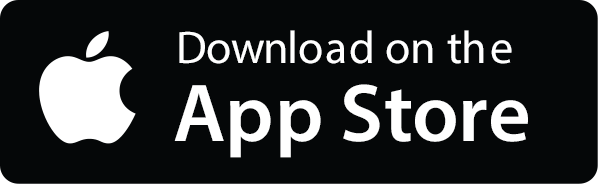
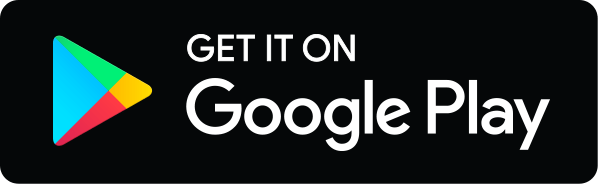