Drug Therapy in the Pediatric Emergency Department*
Shannon F. Manzi
Michael W. Shannon
Introduction
Pharmacotherapy is an essential part of pediatric emergency medicine. Although few data are available, an estimated 70% to 85% of emergency department (ED) encounters result in the prescription of a medication. Illnesses range from minor respiratory infections to cardiorespiratory arrest, and the majority have the administration of medications as a critical intervention. In addition, a significant percentage of children who come to pediatric EDs have a chronic or preexisting condition requiring routine medications (e.g., asthma, seizure disorder, cystic fibrosis, or sickle cell anemia). One study describes the use of an urban pediatric ED documented one-quarter of patients presenting for care had a preexisting diagnosis of a chronic condition (1). For example, between 2002 and 2005, one study demonstrated a 100% increase in the number of prescriptions for type 2 antidiabetic agents prescribed to children between the ages of 5 and 19 years (2). Thus, an understanding of the scope of pediatric drug therapy, including the pharmacology of the drugs commonly prescribed in the pediatric ED setting, is crucial.
Weighted data estimate that the percentage of US children requiring a visit to EDs between 2000 and 2002 was 11.4%, and 14.6% for children of low-income families (2). An urban, tertiary care pediatric hospital and trauma center can average more than 60,000 visits annually. According to the Database for Pediatric Studies statistics for 2000, between 9% and 23% of hospital admissions originate from the ED (3).
Although not exhaustive, this chapter will review the more common complaints and associated drug therapies managed in a pediatric ED. Attention to airway, breathing, and circulation (ABCs) is always the first priority in managing all ED patients. The following discussion describes the “D” in the ABCD algorithm, that is, drug therapy.
Anaphylaxis and Allergic Reactions
Anaphylaxis is a life-threatening emergency in children, requiring urgent intervention. Usually precipitated by an identifiable antigen, anaphylaxis is a systemic allergic response mediated by immunoglobulin E (IgE) and subsequent inflammatory processes (4). Signs and symptoms of anaphylaxis include bronchospasm, urticaria, angiodema, and hypotension. Anaphylactoid reactions present with similar signs and symptoms to anaphylaxis; however, the mechanism is felt to be a direct release of inflammatory components of the cells and not mediated by IgE. For the purposes of this chapter, the term “anaphylaxis” will refer to both true anaphylactic and anaphylactoid reactions.
Treatment
The drug of choice for the initial treatment of anaphylaxis is epinephrine. Epinephrine is a potent sympathomimetic agent and stimulates both α- and β;-adrenergic receptors. Producing vasoconstriction via α-receptor agonism, epinephrine increases blood pressure and decreases capillary leakage (5). As a bronchodilator (acting via β;2-receptor agonism-induced relaxation of the bronchial muscle), epinephrine improves ventilation in the bronchioles and concomitantly increases tidal volume (5). The β;1-receptor agonism results in greater myocardial contraction.
The ideal route of administration of epinephrine in anaphylaxis is intramuscular (IM). In the United States, many practitioners historically administered epinephrine subcutaneously (SC). Although it is effective via this route, when given subcutaneously, epinephrine induces a local vasoconstriction and resultant slow absorption (6). In Canada, Europe, and the United Kingdom, the majority of practitioners prefer the intramuscular route, citing better blood flow and greater predictability of absorption (6,7,8). This has now been adopted by leading experts in the United States as well (9). Intravenous (IV) epinephrine is used in advanced
anaphylactic shock, when blood flow to the extremities is compromised and SC/IM epinephrine is less effective. Caution should be exercised when calculating and infusing the dose if it is given by the intravenous route. In uncompromised anaphylaxis, administration of epinephrine should not be delayed by placement of an intravenous line.
anaphylactic shock, when blood flow to the extremities is compromised and SC/IM epinephrine is less effective. Caution should be exercised when calculating and infusing the dose if it is given by the intravenous route. In uncompromised anaphylaxis, administration of epinephrine should not be delayed by placement of an intravenous line.
Practitioners need to be aware of the correct dosing for epinephrine and potential errors associated with the availability of multiple concentrations. Epinephrine exists as 1:10,000 (0.1 mg per mL) and 1:1,000 (1 mg per mL) concentrations. For intramuscular administration in anaphylaxis, 0.01 mg per kg (0.01 mL per kg, max 0.3 mL per dose) of the 1:1,000 concentration is used to minimize the volume injected. If the intravenous route is used, epinephrine 0.005 mg per kg (0.05 mL per kg) of the 1:10,000 concentration is given. Common adverse reactions include tachycardia and arrhythmia, hypertension, tremor, and headache.
All patients who have experienced an anaphylactic reaction should be given a prescription for an epinephrine autoinjector along with proper instruction for use at discharge (4,6). Autoinjectors are available in 0.15-mg strength for children younger than 8 years (weighing <30 kg) and 0.3 mg for those 8 years or older (weighing at least 30 kg).
Other agents used in the mitigation of anaphylaxis include H1-antagonists. H1-antagonists block the binding of histamine to the receptors on effector cells in the gastrointestinal (GI) tract, blood vessels, and respiratory tract and inhibit vasodilation and vasoconstriction, increased capillary permeability, and edema formation (10). Diphenhydramine is the most commonly used agent for allergic reactions and is available in both injectable and oral (PO) preparations. Dosing is 1.25 mg per kg per dose (max 50 mg per dose) IV/PO every 6 hours around the clock for 24 to 48 hours. Onset of the intravenous preparation occurs within 1 hour and the duration of action is approximately 6 hours. Side effects include sedation, hypotension, and tachycardia.
Corticosteroids are also useful in the treatment of allergic reactions and anaphylaxis, although it is important to note that the peak of action is delayed, approximately 4 to 6 hours following an intravenous dose (6,11). Corticosteroids mitigate both immediate and delayed phases of hypersensitivity reaction. Chemotaxis of white blood cells (WBCs) and circulating inflammatory mediators such as histamine, kinins, prostaglandins, and leukotrienes are decreased following the administration of a corticosteroid (11). Corticosteroids also decrease both vasodilation and vessel permeability, leading to a reduction in edema. Methylprednisolone sodium succinate injection is usually initiated at 2 mg per kg and then continued at a dose of 0.5 to 1 mg per kg per dose (max 80 mg per dose) IV every 6 hours for 24 hours. Methylprednisolone can be converted to oral prednisone or prednisolone when the patient is able to tolerate oral medications at a dose of 1 mg per kg per dose (max 80 mg per dose) PO every 6 to 12 hours. Given the potentially biphasic nature of anaphylaxis, corticosteroids are generally continued for 24 to 48 hours, although their efficacy in preventing a resurgence of symptoms has been questioned (12). Side effects include mood changes, electrolyte alterations, and hypertension.
H2-antagonists are adjuvant medications that may also be added to the patient’s regimen. These agents competitively antagonize the histamine (predominantly H2) receptors primarily in the GI tract and include ranitidine, famotidine, and cimetidine. They are available as injectable and oral preparations. Ranitidine injection is dosed at 1 mg per kg per dose (max 50 mg per dose) IV every 8 hours; it can be converted to oral dosing at 2 mg per kg per dose (max 150 mg per dose) PO every 12 hours. Famotidine injection and oral preparations are dosed at 0.5 mg per kg per dose IV/PO every 12 hours (max 40 mg per dose). The dose of intravenous and oral cimetidine is 5 mg per kg per dose IV/PO divided every 6 to 12 hours depending on age. Because cimetidine is a potent inhibitor of several cytochrome (CYP) isozymes, including CYP1A2, 2D6, 2E1, 2C19, and 3A3/4, there is potential for significant drug interactions if it is used (13). Side effects of the H2-antagonists are similar and include headache, dizziness, GI intolerance, and rare thrombocytopenia.
More often, allergic reactions of a less severe nature are seen in the ED. These may include food, drug, and environmental allergen exposures that result in rash, rhinorrhea, and/or itchy, watery eyes. Often these allergic reactions can be treated with discontinuation or avoidance of the offending agent. In some cases, a traditional antihistamine, such as diphenhydramine, or a nonsedating antihistamine, such as loratadine, fexofenadine, or cetirizine, may be needed. Intranasal steroids and ophthalmic antihistamine preparations may also be helpful.
Infection
Sepsis and Meningitis
Sepsis is defined as the systemic inflammatory response to infection (14). Elevated temperature, tachycardia, hypotension or shock, tachypnea, and elevated WBC count are common findings in a patient with sepsis. Septic infants and children can become seriously ill in a rapid fashion. Infants in particular may present with nonspecific signs and symptoms such as fever and lethargy. Therefore, febrile infants younger than 4 weeks generally require a full “septic workup,” consisting of a lumbar puncture and the collection of blood and urine cultures (15).
Sepsis can progress quite rapidly to septic shock. The resultant hypotension leads to decreased organ perfusion, with altered mental status reflecting the lack of oxygen and nutrients reaching the brain (14). Septic shock carries a mortality of 27% to 43%, depending on time of onset and delay in seeking medical attention (16). Treatment should begin with fluid resuscitation and vasopressors (see later discussion of shock), with the early addition of broad-spectrum antibiotics. Acyclovir should be added if the diagnosis of herpes simplex virus (HSV) sepsis is suspected (17). The most recent Surviving Sepsis Campaign update advocates the use of steroids only in children with suspected or proven adrenal insufficiency (18).
Meningitis may have a presentation similar to sepsis in infants and young children. Nonspecific signs and symptoms include irritability, vomiting, decreased oral intake, lethargy, inconsolable crying, and fever. Seizures and a bulging fontanel are late signs of meningitis. Older patients
may complain of nuchal rigidity, headache, photophobia, and fever. Meningitis may be bacterial, partially treated bacterial, or aseptic in origin. Aseptic meningitis includes viral, fungal, mycoplasma, and drug-induced causes.
may complain of nuchal rigidity, headache, photophobia, and fever. Meningitis may be bacterial, partially treated bacterial, or aseptic in origin. Aseptic meningitis includes viral, fungal, mycoplasma, and drug-induced causes.
Bacterial colonization of mucosal surfaces with subsequent mucosal invasion leads to bacteremia (19). After crossing the blood—brain barrier (BBB), the bacteria enter the central nervous system (CNS) and induce cytokine production (19). Leukocytes accumulate in the cerebrospinal fluid (CSF), and albumin begins to pass through the intercellular junctions of the meninges. Brain edema ensues, increasing intracranial pressure (ICP) and compromising cerebral blood flow (20). Cranial nerve injury, seizures, ischemic injury, and brain herniation can result if the infection is left untreated. Treatment consists of early administration of intravenous antibiotics and may also include dexamethasone in some cases.
Treatment of Sepsis and Meningitis
Detailed discussion of the anti-infective agents can be found in Chapter 29. A brief overview of the anti-infective agents used for the treatment of sepsis and meningitis follows. Penicillins and cephalosporins, the most commonly prescribed antibiotics, are classified as β;-lactam agents based on their essential chemical structure. The β;-lactam antibiotics destroy cell wall–containing bacteria by inactivating the enzyme peptidoglycan transpeptidase (21). By binding irreversibly to the penicillin-binding proteins, β;-lactam antibiotics interrupt the synthesis of the cell wall and subsequently cause the bacteria to rupture.
A major mechanism of penicillin resistance is bacterial production of a β;-lactamase enzyme, usually occurring secondary to gene transfer. The β;-lactamase enzyme hydrolyzes the β;-lactam ring, destroying the structure of the antibiotic (21). To circumvent β;-lactamase production as a means of resistance, a β;-lactamase inhibitor such as clavulanic acid or sulbactam is added to the β;-lactam antibiotics. Although these inhibitors possess weak or no intrinsic antimicrobial activity, they protect the β;-lactam antibiotic from hydrolysis and thus expand the spectrum of activity (22). Examples include amoxicillin–clavulanic acid and ampicillin–sulbactam.
Table 24.1 Empiric Treatment of Sepsis | ||||||||||||||||||||||||||||||||||||||||
---|---|---|---|---|---|---|---|---|---|---|---|---|---|---|---|---|---|---|---|---|---|---|---|---|---|---|---|---|---|---|---|---|---|---|---|---|---|---|---|---|
|
Aminoglycoside antibiotics exert their antimicrobial effect by binding to the 30S ribosome, subsequently inhibiting bacterial protein synthesis (23). The primary target of aminoglycoside antibiotics is gram-negative organisms, although they are often used synergistically with β;-lactam antibiotics for certain gram-positive infections. Aminoglycosides are widely distributed in extracellular fluid and have relatively poor tissue penetration. Dosing for obese patients should be based on adjusted body weight. Aminoglycosides are concentration-dependent bactericidal antibiotics and extended-interval dosing (i.e., “once daily”) has garnered interest in the pediatric population. Many regimens for extended-interval dosing have been proposed and are still being debated in the literature (24). The elimination of aminoglycosides is dependent on renal function; therefore, patients with reduced creatinine clearance (calculated creatinine clearance of <60 mL per minute per 1.73 m2) should not receive extended-interval dosed aminoglycosides. Aminoglycosides can be inactivated by penicillin derivatives, possibly from the formation of an inactive amide with the open β;-lactam ring (25). Coadministration should be separated by at least 30 minutes.
Vancomycin is a glycopeptide antibiotic primarily effective against gram-positive organisms. Similar to β;-lactam antibiotics, vancomycin inhibits synthesis of the cell wall. However, vancomycin binds at the d-alanyl–d-alanine terminus and inhibits the release of building blocks necessary for cell wall synthesis (26). Vancomycin is a large molecule with poor distribution into the CNS, although greater penetration occurs when the meninges are inflamed. The elimination of vancomycin is also dependent on renal function and requires dosage adjustment in patients with calculated creatinine clearances of less than 70 mL per minute per 1.73 m2.
Age-specific bacterial pathogen patterns for sepsis and meningitis must be appreciated to choose the most appropriate empiric antibiotic therapy. Neonates and infants should receive antimicrobial agents that cover organisms acquired during birth (27). Immunocompromised hosts presenting with fever should be treated with an agent that provides adequate gram-negative antibiotic coverage, including activity against Pseudomonas aeuriginosa. Vancomycin should be added if there is evidence of infection around a central line or other indwelling catheter (28) (Table 24.1).
|
As with sepsis, age-specific bacterial pathogen patterns for meningitis must be appreciated to choose the most appropriate antibiotic therapy. Neonates and infants younger than 1 month are most likely to develop meningitis secondary to vaginal flora acquired during birth. Group B streptococcus, Listeria monocytogenes, and Escherichia coli are common causes of neonatal meningitis (27,29). Infants between the ages of 1 and 3 months require coverage for the same pathogens as neonates; however, Streptococcus pneumoniae and Neisseria meningitidis must also be considered (30). Infants older than 3 months and children are most likely to present with meningitis caused by S. pneumoniae and N. meningitidis (20). Immunocompromised patients may present with atypical organisms such as L. monocytogenes or gram-negative bacilli (30). Cochlear implants have been associated with an increased risk of meningitis, predominantly with S. pneumoniae (31,32). Patients with ventriculoperitoneal shunts or other hardware may present with Staphylococcal meningitis. All patients with suspected S. pneumoniae or staphylococcal meningitis should receive vancomycin until susceptibility results are available.
With meningitis, several factors affect drug penetration into the CNS. Inflammation of the meninges allows larger and more-polar drug molecules to penetrate into the CSF (30). Degree of ionization, lipid solubility, and protein binding are all characteristics that influence the efficacy of antibiotics chosen to treat meningitis. High doses of β;-lactam antibiotics and vancomycin are required to achieve necessary CSF concentration to minimum inhibitory concentration ratios for bactericidal activity. A lower pH of the CSF in meningitis decreases the activity of aminoglycoside and macrolide antibiotics (30) (Table 24.2).
Steroid use in childhood meningitis continues to be a controversial topic, reignited in part by recent studies in adults indicating a possible benefit in patients who receive dexamethasone prior to or just at the time of antibiotic administration (33,34). The greatest benefit of dexamethasone in pediatric patients has been demonstrated in Haemophilus influenzae meningitis, a disease that is now rarely seen following the widespread immunization of children (34,35,36). A possible explanation for the positive outcomes in patients receiving dexamethasone is depression of inflammatory response that follows the antibiotic-induced lysis of bacteria. Bacterial cell wall breakdown leads to the release of cytokines such as tumor necrosis factor, interleukin-6 (IL-6), and IL-1 into the subarchnoid space, increasing leukocyte accumulation and inflammation (29,30,33,37). By decreasing inflammation, corticosteroids may mitigate or prevent sequelae such as hearing loss, and therefore should be considered in S. pneumoniae and N. meningitidis meningitis (29,30). Other experts have cautioned against the use of corticosteroids in children with presumed meningitis who have been vaccinated against H. influenzae (38). Concerns around the use of steroids in meningitis include masking of clinical
response, GI hemorrhage, and decreases in the CNS penetration of antibiotics with large molecular weights, such as vancomycin (33,37). However, one study in pediatric patients and a recent adult study demonstrated no reduction in cephalosporin or vancomycin CSF penetration with concomitant steroid therapy (39,40).
response, GI hemorrhage, and decreases in the CNS penetration of antibiotics with large molecular weights, such as vancomycin (33,37). However, one study in pediatric patients and a recent adult study demonstrated no reduction in cephalosporin or vancomycin CSF penetration with concomitant steroid therapy (39,40).
Acyclovir is an antiviral agent that is incorporated into the viral DNA and competes for DNA polymerase, inhibiting viral replication. Acyclovir should be added in all children in whom the diagnosis of HSV meningoencephalitis is suspected, and particularly in infants younger than 30 days (41,42). Lack of prompt treatment can have devastating consequences, including permanent neurologic damage. Dosing for intravenous acyclovir is doubled to 60 mg per kg per day IV divided every 8 hours when HSV encephalitis/meningitis is suspected. Acyclovir is widely distributed into body tissues, with CSF concentrations reaching 50% of the serum concentrations. Renal excretion accounts for up to 90% of acyclovir elimination, and therefore the dose must be adjusted for renal insufficiency (43). Slow infusions and adequate hydration are necessary to prevent drug crystallization into the renal tubules and subsequent renal damage.
Prophylaxis of day care and household contacts may be necessary when a patient is diagnosed with meningococcal meningitis (44). In addition, persons directly exposed to secretions (e.g., during endotracheal intubation) may also require postexposure prophylaxis (45). In outbreaks caused by a serotype contained in the vaccine (A, C, Y, and W-135), immunization of the exposed groups may be recommended by public health authorities (Table 24.3).
Cellulitis
Cellulitis is a localized bacterial infection of the skin and soft tissue, most often caused by staphylococcal and streptococcal species (46). The incidence of community-acquired methicillin-resistant staphylococcus aureus (CA-MRSA) has been rising exponentially in the past 5 years. One study demonstrated a rate increase from 9% in 2004 to 21% in 2006, necessitating a shift in empiric treatment (47). Emergent cellulitis infections include orbital and periorbital cellulitis and necrotizing fascitis. Cellulitis secondary to human or animal bites will be covered later in the chapter.
Cellulitis can follow minor trauma, such as a cut or a scratch. Because skin floras are likely to be the causative agents, empiric antibiotic therapy should have adequate staphylococcal and streptococcal coverage. If a mixed anaerobic infection is suspected, ampicillin–sulbactam or clindamycin provide additional coverage. Mild to moderate cellulitis can be successfully treated with oral agents on an outpatient basis (46). First-line antibiotics for cellulitis include oral penicillinase-resistant β;-lactams such as dicloxacillin or a first-generation cephalosporin such as cephalexin (46). If CA-MRSA is suspected, first-line agents may include clindamycin or trimethoprim-sulfamethoxazole depending on local resistance patterns. Cellulitis with purulent drainage may require hospitalization and parenteral antibiotics.
Table 24.3 Postexposure Prophylaxis for Neisseria Meningitidis | |||||||||||||||||||||||
---|---|---|---|---|---|---|---|---|---|---|---|---|---|---|---|---|---|---|---|---|---|---|---|
|
Periorbital cellulitis usually occurs secondary to trauma, although it can also be the result of contiguous microbial spread from the sinuses or be hematogenous in origin. The affected eye is red with significant eyelid swelling. Seventy-five percent of patients with periorbital cellulitis have fever (47). If bacteremia is present, the child may have a temperature of more than 39°C and a WBC count of more than 15,000 cells per mm3 (47). Prior to the introduction of the vaccine, H. influenzae was one of the most common pathogens implicated in periorbital and orbital cellulitis. Since 1990, when universal vaccination began, the number of cases of H. influenzae periorbital and orbital cellulitis and the overall number of cases have declined (48). Currently, staphylococcal and streptococcal species are responsible for the majority of periorbital cellulitis. Periorbital cellulitis can be treated with intramuscular ceftriaxone or oral antibiotics such as a first-generation cephalosporin, amoxicillin–clavulanate, clindamycin, or bactrim on an outpatient basis if there is no retrobulbar involvement (48).
Orbital cellulitis is typically a complication of sinusitis (47). With these infections, eyelid tissue becomes edematous, and a periorbital purple discoloration may ensue. Proptosis, ophthalmoplegia, pain on movement of the eye, and decreased visual acuity necessitate rapid imaging and ophthalmologic consultation. Orbital cellulitis requires hospitalization and treatment with parenteral antibiotics, primarily ceftriaxone or cefotaxime plus vancomycin (49). Infectious organisms generally include Staphylococcus aureus, Streptococcus pyogenes, and S. pneumoniae (50).
Necrotizing fasciitis is an infection of the skin and subcutaneous soft tissue resulting in necrosis, often caused by invasive group A β;-hemolytic streptococcus (GABHS).
Superantigens, in the form of exotoxins, are released by GABHS and induce massive cytokine release (51). Local inflammation produces extensive tissue damage and shock. Despite a high mortality rate for adults of 30% to 80%, childhood mortality from invasive GABHS infections has a rate of 5% to 10% (51). The highest risk factor in children for the development of invasive GABHS is intercurrent varicella infection (51,52).
Superantigens, in the form of exotoxins, are released by GABHS and induce massive cytokine release (51). Local inflammation produces extensive tissue damage and shock. Despite a high mortality rate for adults of 30% to 80%, childhood mortality from invasive GABHS infections has a rate of 5% to 10% (51). The highest risk factor in children for the development of invasive GABHS is intercurrent varicella infection (51,52).
Treatment of Necrotizing Fasciitis
Treatment of necrotizing fasciitis includes early surgical debridement and administration of parenteral antibiotics. Intravenous penicillin is the drug of choice for GABHS, dosed at 400,000 units per kg per day IV divided every 4 hours. Clindamycin is advocated by many experts as an adjuvant therapy following reports of improved survival in animal trials (53). Theoretically, clindamycin may be more efficacious in overcoming the large inoculum of organisms due to slow replication and decreased number of penicillin-binding proteins, which may inhibit β;-lactam antimicrobial efficacy (51). Clindamycin also inhibits exotoxin production via inhibition of protein synthesis by binding to the 50S subunit on bacterial ribosomes (26,53,54). Because of the rising incidence of bacterial resistance and the bacteriostatic properties (dependent on concentration) of clindamycin, it should not be used as the sole agent (51). Clindamycin is dosed at 40 mg per kg per day IV divided every 6 hours (max 4.8 g per day). Although rare, pseudomembranous colitis with severe, persistent diarrhea can occur with clindamycin administration and may be fatal. Prophylaxis of contacts exposed to necrotizing fasciitis is controversial, and data on effectiveness are lacking.
Pneumonia
Community-acquired pneumonia is common in children, especially those younger than 5 years (55,56,57,58). Signs and symptoms include fever, acute respiratory distress, and infiltrates on chest radiograph. The prevalent bacterial organisms vary based on age, and therefore the most appropriate empiric antibiotics vary (see Table 24.4) (55,59). The resistance patterns of S. pneumoniae in pneumonia are similar to that in meningitis, although the serum concentrations of β;-lactam antibiotics in the serum generally exceed the minimum inhibitory concentration severalfold (36). Recently, there has been an estimated 35% reduction in the incidence of S. pneumoniae pneumonia following the release of the heptavalent pneumococcal vaccine (Prevnar®) (55).
Atypical organisms such as Chlamydia pneumoniae and Mycoplasma pneumoniae are increasingly common pathogens found in children older than 4 months, particularly those older than 4 years (55,58). Concomitant infection with mixed bacterial organisms or bacterial with viral infection can occur in up to 25% of children with pneumonia, most frequently S. pneumoniae with respiratory syncytial virus (RSV) or M. pneumoniae (59,60). In cases of severe necrotizing pneumonia, addition of antimicrobial agents with activity against S. aureus, such as oxacillin or nafcillin, is warranted (55,56). Vancomycin should be added if the child is at risk for infection with MRSA.
Viral and bacterial pneumonia may have similar appearance on chest radiograph. Clinically, pneumonia accompanied by wheezing is more likely to be viral in origin, whereas chest pain as a result of pleural irritation is likely to be bacterial (55). RSV will be discussed further in the “Respiratory Distress Syndromes” section of this chapter.
Treatment
Empiric treatment of community-acquired pneumonia in children includes penicillins, macrolides, and doxycycline (55). Aminoglycosides, cephalosporins, and antistaphylococcal agents are added for severe pneumonia. The pharmacology of penicillins, cephalosporins, vancomycin, and aminoglycosides has been discussed previously.
The most common macrolides used for the treatment of community-acquired pneumonia in children include azithromycin and erythromycin. Azithromycin and erythromycin are bacteriostatic antibiotics that bind to the 50S ribosome in susceptible organisms, inhibiting protein synthesis (26). Azithromycin displays extensive tissue distribution, resulting in a high intracellular concentrations and subsequent long half-life. Erythromycin is widely distributed in body fluids except the CNS, although it has less tissue binding than azithromycin. Both azithromycin and erythromycin are available in intravenous and oral preparations. Erythromycin is acid sensitive and is therefore administered in enteric-coated formulations to improve stability (61). Azithromycin and erythromycin are metabolized in the liver. Erythromycin inhibits isozymes CYP1A2 and 3A3/4, resulting in multiple reported drug interactions (61). Azithromycin is a mild inhibitor of CYP3A3/4 and demonstrates few clinically significant drug–drug interactions (62). Common side effects include nausea, vomiting, and diarrhea, especially with erythromycin. Studies recently published describe pyloric stenosis occurring in neonates who have received erythromycin and suggest that it is not a class effect seen with all macrolides (63,64). Therefore, azithromycin may be a better option for infants requiring a macrolide antibiotic.
Doxycycline is a tetracycline antibiotic, binding to the 30S ribosome in susceptible organisms and inhibiting protein synthesis (26). Doxycycline has a broad spectrum of activity, including gram-positive, gram-negative, and atypical organisms. Distribution is extensive and tissue penetration is excellent, although CNS penetration is poor. Doxycycline is partially chelated in the GI tract and minimally excreted via the kidneys. Side effects include GI distress, photosensitivity, hepatotoxicity, and teeth staining in children younger than 8 years (Table 24.4).
A lower hospital admission rate has been demonstrated in children with pneumococcal pneumonia with associated bacteremia when treated with an initial parenteral dose of antibiotics prior to outpatient oral therapy as compared with those who receive oral antibiotics alone (60). Parenteral antibiotics may achieve higher concentrations in sites of consolidation, resulting in more rapid improvement.
Aspiration pneumonia, especially in children with chronic airway problems or severe reflux, can present in the ED. If antibiotic therapy is necessary, antimicrobial coverage should cover oral anaerobes. Despite concerns over penicillin-resistant Bacteroides species, one study has
shown equivalent efficacy between intravenous penicillin G and intravenous clindamycin (65). Other empiric choices may include ampicillin–sulbactam with or without an aminoglycoside, dependent on severity.
shown equivalent efficacy between intravenous penicillin G and intravenous clindamycin (65). Other empiric choices may include ampicillin–sulbactam with or without an aminoglycoside, dependent on severity.
|
Osteomyelitis and Septic Arthritis
Bacterial infections of bone (osteomyelitis) and joint (septic arthritis) are a significant cause of morbidity in children (66,67,68). Presentation may vary from unwillingness to move an extremity, to limp, to pseudoparalysis, to local swelling. Fever, pain, and elevated erythrocyte sedimentation rate and C-reactive protein are common features of both infections, although neonates may present with nonspecific signs such as lethargy and decreased oral intake. Delay in treatment, particularly with septic arthritis, can result in irreversible damage to the articular cartilage (66). As with other infectious processes, H. influenzae was the dominant pathogen prior to widespread vaccination against it. Currently, the most common causes of osteomyelitis and septic arthritis are S. aureus and Streptococcus species (67,68). Neonates and immunocompromised hosts can present with infection by gram-negative organisms; empiric antimicrobial coverage should reflect this possibility. Sickle cell patients demonstrate higher rates of Salmonella osteomyelitis than the general population (69).
Treatment
Antistaphylococcal β;-lactam antibiotics are recommended as first-line agents for the treatment of both osteomyelitis
and septic arthritis (67,68). Agents chosen must possess good bone and synovial fluid penetration. Initial intravenous therapy can be transitioned to oral antibiotics. Length of intravenous and subsequent oral therapy is patient specific. Traditionally, 6 to 8 weeks of antibiotic treatment was thought to be necessary, although recent studies have shown similar outcomes with shorter, 3-week courses of antibiotics (70,71).
and septic arthritis (67,68). Agents chosen must possess good bone and synovial fluid penetration. Initial intravenous therapy can be transitioned to oral antibiotics. Length of intravenous and subsequent oral therapy is patient specific. Traditionally, 6 to 8 weeks of antibiotic treatment was thought to be necessary, although recent studies have shown similar outcomes with shorter, 3-week courses of antibiotics (70,71).
Patients with sickle cell disease and suspected osteomyelitis should receive coverage for both Salmonella and S. aureus until culture results are available. Empiric choices include a third-generation cephalosporin or a fluoroquinolone plus an antistaphylococcal β;-lactam (67). Puncture wounds with resulting osteomyelitis may require pseudomonal coverage. Four to 6 weeks of therapy is suggested to prevent relapse (72).
Otitis Media
Otitis media is defined as the inflammation of the middle ear and is classified as either acute otitis media (AOM) or otitis media with effusion (73). AOM is more commonly encountered in the ED and will be discussed here. AOM is characterized by fever, pain in the affected ear(s), otorrhea, or a bulging tympanic membrane. It has been well established that up to 80% of AOM episodes will spontaneously resolve if left untreated, although identifying those cases not requiring therapy may be difficult (36). The most common bacterial causes of AOM include S. pneumoniae, H. influenzae, and Moraxella catarrhalis (21,74). Given recent resistance patterns of penicillin resistance in S. pneumoniae AOM, it is important to achieve adequate antibiotic levels in the middle ear fluid. Pneumococci and other organisms develop resistance through development of penicillin-binding protein mutations. High-dose penicillin (usually amoxicillin) may be efficacious when otitis media secondary to penicillin-resistant organisms.
Treatment
In the child older than 2 years, AOM can usually be treated symptomatically with analgesic agents alone. If antibiotic treatment is warranted, the drug of choice for otitis media is high-dose amoxicillin at 80 to 100 mg per kg per day PO divided thrice daily for 5 to 7 days (74,75,76). The higher dosing range of amoxicillin should provide adequate coverage against penicillin-nonsusceptible S. pneumoniae (76). For patients allergic to penicillins, alternative agents include azithromycin 30 mg per kg PO × 1 dose (62). Azithromycin can also be given as a 5-day regimen dosed at 10 mg per kg on the first day, followed by 5 mg per kg per day for 4 days. The primary side effects of azithromycin are GI cramping, vomiting, and diarrhea (62).
Treatment failures following high-dose amoxicillin may be due to resistant S. pneumoniae or β;-lactamase–producing H. influenzae, and may respond to amoxicillin–clavulanic acid, cefuroxime, or intramuscular ceftriaxone (76). Amoxicillin–clavulanic acid distributes well into middle ear effusions, achieving bactericidal concentrations (21). High-dose amoxicillin–clavulanic acid contains 90 mg per kg per day of the amoxicillin component and may be necessary in cases of penicillin-resistant S. pneumoniae. Twice-daily administration of the high-dose amoxicillin–clavulanic acid has produced similar response rates as three divided doses per day (21). The recommended length of treatment is generally 5 to 7 days, unless the patient is at risk for treatment failure (36). The patients at risk for treatment failure tend to be younger than 18 to 24 months, have a history of recurrent AOM, or have underlying immunologic or anatomic abnormalities (36). These patients should receive 10 days of treatment. Common side effects of amoxicillin-clavulanic acid include diarrhea, vomiting, and rash.
Streptococcal Pharyngitis
Symptoms of pharyngitis include sore throat, headache, and fever. Viral pharyngitis can be virtually indistinguishable from bacterial pharyngitis on physical examination. However, the presence of rhinorrhea, conjunctivitis, or cough is more suggestive of a viral etiology. GABHS is the most common causative organism of bacterial pharyngitis and can have serious sequelae, including poststreptococcal glomerulonephritis and acute rheumatic fever/carditis, if left untreated (77,78). Other bacterial causes of pharyngitis do not require treatment, as no benefit from antimicrobial therapy has been demonstrated (77). Therefore, a rapid strep antigen detection test and/or throat culture is necessary to make the diagnosis. A positive result is the criterion for antibiotic therapy (36). The main goal of antibiotic therapy is the prevention of rheumatic fever. This complication can be averted if treatment is instituted within 9 days of onset of symptoms (78).
Treatment
First-line treatment with penicillin is recommended due to its efficacy and safety profile, narrow spectrum, and low cost (77,78). Amoxicillin is often substituted due to better tolerability of the oral suspension. Alternatively, benzathine penicillin G can be given intramuscularly to patients who are unlikely to be compliant with therapy. Benzathine penicillin G should never be given via the intravenous route, as fatalities have occurred (79). Peak serum levels are achieved within 12 to 24 hours following intramuscular administration and remain detectable for 1 to 4 weeks. Pain on injection is common and can be ameliorated by warming the injection to room temperature prior to administration.
Macrolides are acceptable alternatives in children with penicillin allergy (78). For patients who are penicillin allergic, but do not exhibit type-I hypersensitivity to penicillin, first-generation cephalosporins may be used. For patients who have failed treatment despite adequate antimicrobial therapy, amoxicillin–clavulanate and clindamycin are excellent choices for eradication of group A streptococcus (77) (Table 24.5).
The course of therapy is traditionally 10 days to eradication of the organism. Shorter courses have been recommended, although concerns regarding equivalence to standard therapy have been raised (77).
|
Pain
Acute pain is one of the most common adverse experiences among pediatric patients (80). Pain in children presenting to the ED has varied etiologies. Common causes include fractures and sprains, sickle cell disease, and migraine headaches. The goal of pain management is early, effective control with appropriate monitoring (80,81). Pain medication options include opioids, nonsteroidal anti-inflammatory drugs (NSAIDs), acetaminophen, and adjuvant agents such as topical anesthetics. Each drug used for pain has a unique profile and different benefits and risks. Distraction techniques, relaxation, and physical therapy are also important components of effective pain management (80).
Traditionally, practitioners have been reluctant to utilize appropriate doses of pain medications, particularly opioids, for fear of causing respiratory depression and creating addiction from prolonged use (74,81). Although drug-seeking behavior does occasionally occur in those who take opioids regularly, the vast majority of patients who come to the ED reporting pain are truly in distress and require analgesia.
Opioids are classified as centrally acting receptor agonists, partial agonists, or mixed agonist–antagonists (82). The opioid receptors are categorized as mu (μ), kappa (κ), delta (δ), and sigma (σ) (83). μ-Receptor activation results in analgesia, respiratory depression, miosis, decreased GI motility, and euphoria. The κ- and σ-receptors are responsible for analgesia, dysphoria, and psychomimetic reactions, primarily acting in the spinal cord (84). The δ-receptors may be responsible for some analgesic responses to thermal stimuli.
Adverse reactions are similar within the class and include respiratory depression, sedation, nausea and vomiting, and constipation. Histamine release more commonly occurs with morphine, meperidine, and codeine, resulting in urticaria, generalized pruritis, and hypotension (84).
Morphine is the most commonly used opiate (82,84). As a μ-receptor agonist, morphine is a potent pain reliever. Onset occurs within 15 to 30 minutes following IV administration and within 30 to 60 minutes following IM administration (84). Duration is usually 3 to 5 hours after IV, IM, or SC administration. The parenteral dose of morphine is commonly 0.05 to 0.1 mg per kg per dose IV/IM/SC. Hypotension, respiratory depression, miosis, bronchospasm, and decreased GI motility are adverse effects of morphine administration (82,84).
Parenteral hydromorphone is a powerful opioid, approximately 6.5 times as potent as morphine. Onset time is similar to morphine, occurring within 15 to 30 minutes following an intravenous dose. Dosing references have recently revised initial dose recommendations due to reports of adverse effects from clinical experts (85). The initial intravenous dose in an opiate-naive patient should start at 0.015 mg per kg per dose, and the usual maximum first dose range from 0.2 to 0.6 mg. Duration is approximately 4 to 5 hours. Common adverse reactions include hypotension, bradycardia, sedation, and GI disturbances.
Fentanyl is potent lipophilic opioid available in parenteral, transdermal, and oral lozenge forms. As a μ-receptor agonist, fentanyl is 100 times more potent than morphine (86). In the ED, fentanyl injection is commonly used for pain relief and procedural sedation and analgesia (PSA). Fentanyl does not release histamine and thus results in negligible changes in hemodynamic status (86). Therefore, it is the agent of choice for patients with mild hypotension who require pain control. Onset of action is rapid, approximately 30 seconds, following intravenous administration (84). Duration of action is approximately 30 to 60 minutes when given at a dose of 1 to 2 μg per kg
intravenously. Hypoxemia and apnea occur more frequently when fentanyl is combined with a sedative, such as midazolam (84,87). Chest wall and tongue rigidity, marked by muscle rigidity, respiratory distress, hypercapnia, hypoxia, and difficult intubation, can occur with rapid administration of fentanyl (88). If it does occur, naloxone at 10 μg per kg per dose can effectively reverse chest wall rigidity (88,89). Neuromuscular blocking agents such as succinylcholine or pancuronium have also been successfully used to reverse chest wall rigidity but will mandate endotracheal intubation (88).
intravenously. Hypoxemia and apnea occur more frequently when fentanyl is combined with a sedative, such as midazolam (84,87). Chest wall and tongue rigidity, marked by muscle rigidity, respiratory distress, hypercapnia, hypoxia, and difficult intubation, can occur with rapid administration of fentanyl (88). If it does occur, naloxone at 10 μg per kg per dose can effectively reverse chest wall rigidity (88,89). Neuromuscular blocking agents such as succinylcholine or pancuronium have also been successfully used to reverse chest wall rigidity but will mandate endotracheal intubation (88).
Meperidine is generally not used as a first-line agent in childhood acute pain management. Nausea and vomiting are common side effects and the drug is difficult to titrate (84). Neurotoxicity may occur with meperidine due to formation of its primary metabolite, normeperidine. Accumulation of normeperidine can result in tremors, irritability, and seizures (87). Meperidine is a potent inhibitor of serotonin reuptake into presynaptic neurons and therefore can interact with other medications that also affect serotonin, such as selective serotonin reuptake inhibitors, tricyclic antidepressants (TCAs), and amphetamines (90). Concurrent use of meperidine with monoamine oxidase inhibitors should always be avoided because the combination can lead to severe serotonin syndrome characterized by malignant hypertension and can be fatal (87,90,91).
The oral opioids frequently used in the ED are summarized in Table 24.6.
Acetaminophen is the most commonly used nonopioid analgesic in children. Its mechanism of action involves the inhibition of cyclooxygenase and prostaglandin synthetase in the CNS in a greater proportion than the periphery, therefore accounting for acetaminophen’s antipyretic effect and apparent lack of anti-inflammatory effects (82,91). Dosing is 10 to 15 mg per kg per dose PO/PR every 4 to 6 hours as needed. Single-dose protocols for 30 mg per kg oral loading doses have been shown to lower fever more quickly than the traditional 15 mg per kg dose with no increase in adverse effects (92). Maximum daily dose should not exceed 90 mg per kg per day or 4 g per day, whichever is less. Acetaminophen is metabolized in the liver, primarily via the sulfation pathway in children (91). In overdose settings, a greater amount of acetaminophen is metabolized outside the major pathways of sulfation and glucuronidation, via the CYP450 isozyme system (93). The resultant metabolite is toxic to the hepatocytes. Adverse effects are minimal at therapeutic doses, but hepatotoxicity leading to liver failure and death can occur with overdose.
Table 24.6 Oral Opioid Agents | |||||||||||||||||||||||||||||||||||||||||||||||||||||||||||||||||||||||||||||||||||||||||
---|---|---|---|---|---|---|---|---|---|---|---|---|---|---|---|---|---|---|---|---|---|---|---|---|---|---|---|---|---|---|---|---|---|---|---|---|---|---|---|---|---|---|---|---|---|---|---|---|---|---|---|---|---|---|---|---|---|---|---|---|---|---|---|---|---|---|---|---|---|---|---|---|---|---|---|---|---|---|---|---|---|---|---|---|---|---|---|---|---|
|
NSAIDs can be used for pain control instead of or in conjunction with opioid analgesics. NSAIDs are cyclooxygenase inhibitors and prevent the formation of prostaglandins. Prostaglandins, particularly PGE2, are released when cells are damaged or when the level of circulating cytokine increases (82). Therefore, NSAIDs are excellent choices for the treatment of pain associated with inflammation. Ibuprofen is widely used, dosed at 10 mg per kg per dose (max 600 to 800 mg per dose) PO every 6 to 8 hours as needed. Onset occurs in approximately 60 minutes and the duration is usually 6 to 8 hours. Side effects include GI irritation and ulceration, GI hemorrhage, impaired platelet function, and allergy. The propensity to cause GI hemorrhage is greater with ketorolac and naproxen compared with ibuprofen (87).
Ketorolac is the only available injectable nonspecific NSAID in the United States (ibuprofen injection is indicated only for PDA closure in the newborn at this time). Although not approved by the Food and Drug Administration (FDA) for use in children younger than 17 years, ketorolac is commonly used in pediatric patients older than 1 year (84,94). Pain control with 30 mg of intravenous ketorolac has been demonstrated comparable with 4 mg of intravenous
morphine (95). Single-dose treatment doses range from 0.4 to 1 mg per kg IV/IM to a maximum of 30 mg in children weighing less than 50 kg and 60 mg in children weighing more than 50 kg. Multiple dosing should be no greater than 0.5 mg per kg per dose (maximum 30 mg per dose) IV/IM every 6 hours not to exceed 20 doses (84). Onset of action occurs 10 minutes after administration, peak effect occurs within 40 to 60 minutes, and the duration of action is approximately 6 hours (84). The risk for GI hemorrhage increases exponentially after day 5 of therapy and is the subject of a “black box” warning for ketorolac (95). Additional adverse effects include hemorrhage outside the GI tract, nausea, diarrhea, headache, and drowsiness.
morphine (95). Single-dose treatment doses range from 0.4 to 1 mg per kg IV/IM to a maximum of 30 mg in children weighing less than 50 kg and 60 mg in children weighing more than 50 kg. Multiple dosing should be no greater than 0.5 mg per kg per dose (maximum 30 mg per dose) IV/IM every 6 hours not to exceed 20 doses (84). Onset of action occurs 10 minutes after administration, peak effect occurs within 40 to 60 minutes, and the duration of action is approximately 6 hours (84). The risk for GI hemorrhage increases exponentially after day 5 of therapy and is the subject of a “black box” warning for ketorolac (95). Additional adverse effects include hemorrhage outside the GI tract, nausea, diarrhea, headache, and drowsiness.
Local anesthetic agents will be covered later in the chapter during the discussion of laceration management.
Procedural Sedation
PSA, also erroneously termed conscious sedation, refers to the process of inducing sedation in a patient for the purpose of obtaining stillness for imaging or for completion of a painful procedure such as laceration repair or fracture reduction. Opioids, benzodiazepines, barbiturates, ketamine, propofol, and chloral hydrate are the most common medications use to facilitate PSA in children. A concern with using procedural sedation is the need for appropriate monitoring of adverse events both during and following the procedure (84,96,97,98). Death, permanent neurologic injury, and prolonged hospitalization are potential adverse outcomes of procedural sedation (98). Prolonged recovery effects can include ataxia, agitation, GI effects, and restlessness (97). Medication errors and drug interactions can contribute to an increased rate of adverse side effects associated with procedural sedation (98). However, one study demonstrated a low incidence of adverse events (2.3%) associated with procedural sedation in more than 1,000 patients in a pediatric ED (99).
Treatment
Fentanyl is the most commonly used opioid for PSA. Fentanyl is frequently used in conjunction with benzodiazepines, primarily midazolam, for procedural sedation. See the “Pain” section for a discussion of fentanyl pharmacology.
Midazolam is a relatively short-acting benzodiazepine, which exerts a sedative effect by binding to the benzodiazepine receptor on the γ-aminobutyric acid (GABA) complex (100). This action enhances GABA binding to the receptor and increases chloride currents into the cell, inhibiting action potential generation (87). Hypnotic effect is usually seen at doses lower than those producing respiratory depression. Midazolam is dosed at 0.05 to 0.1 mg per kg per dose (max 2 mg per dose) IV every 3 to 5 minutes as needed to obtain adequate sedation. It is important to note that adolescents and adults do not require as high a dose of midazolam to achieve sedation as do younger children. Onset of sedation occurs within 1 to 5 minutes after IV administration and within 5 minutes following IM administration. Duration is commonly 20 to 30 minutes after IV administration. Intramuscular administration results in a significantly longer duration of action, ranging from 2 to 6 hours in some cases. Adverse effects include prolonged sedation, hypotension, bradycardia, paradoxical reactions, muscle tremors, and respiratory depression. Because midazolam is a sedative hypnotic and does not possess analgesic effects, fentanyl is often used in conjunction for painful procedures. Fentanyl and midazolam combinations result in greater respiratory depression than midazolam alone (87).
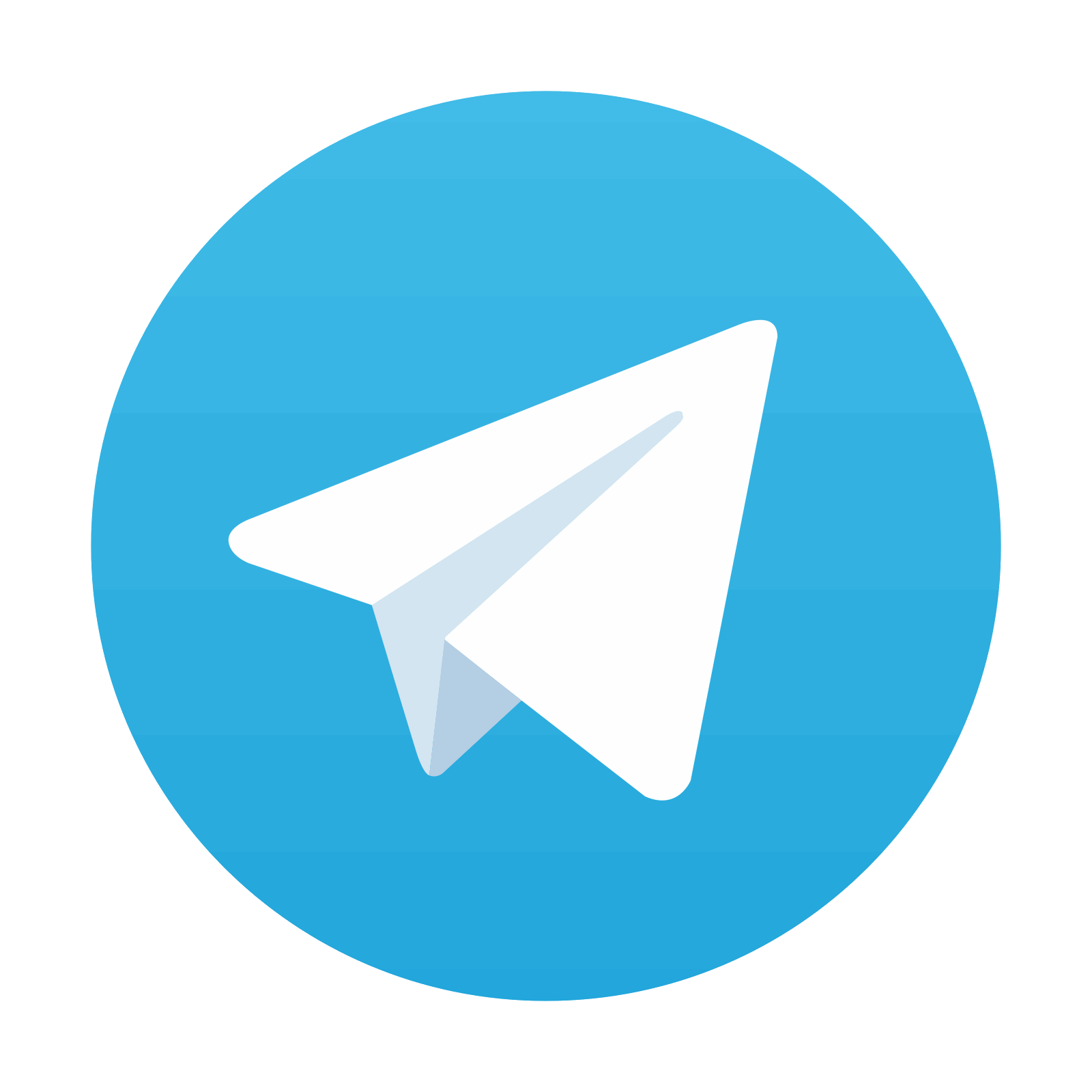
Stay updated, free articles. Join our Telegram channel

Full access? Get Clinical Tree
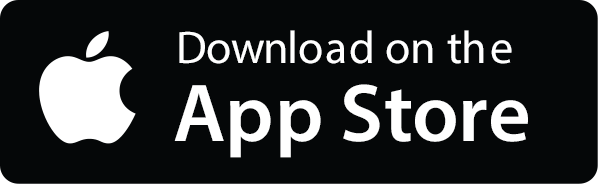
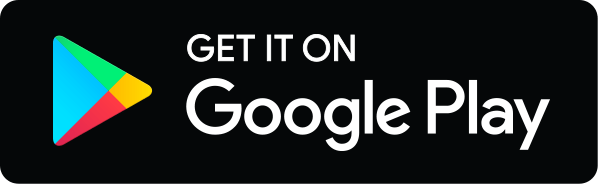