Drug Interactions in Newborns and Children
Kelly P. Shaw
Shannon Manzi
Introduction
The administration of multiple drugs to one patient is a common occurrence, in particular when the patient is a critically, or chronically, ill newborn or child. The risk of drug interactions increases in relation to the number of medications being administered. In addition to drug–drug interactions, more is being learned about drug–herb, drug-dietary supplement, and drug–food interactions. These interactions can range in severity from theoretical to clinically significant, including prolonged morbidity and even mortality. This chapter reviews the principal information concerning the properties and mechanisms of drug–drug interactions.
Absorption/Administration
Simultaneous administration of orally administered drugs sets the stage for potentially clinically significant drug–drug interactions. Absorption of drugs from the gastrointestinal (GI) tract is complex and may be impacted in a variety of ways. Numerous factors are accountable in determining the amount of drug that is absorbed by the body, including age, hydrochloric acid secretion, gastric emptying time, intestinal motility, and bile acid secretion. The primary mechanism of absorption is passive diffusion of nonionized drug molecules via the lipophilic GI mucosa. Subsequently, drugs that change the pH, gastric emptying time, or GI motility will interact with the absorption of other medications. Drug interactions related to GI absorption generally fall into one of eight categories: adsorption, complexation or chelation, resin binding, increased GI motility, decreased GI motility, increased gastric pH, decreased intestinal flora, and the modification of metabolism within the GI wall.
Interactions of adsorption are best described with activated charcoal. The large surface area of activated charcoal allows for adsorption of other drugs and this feature is utilized advantageously in the treatment of toxic exposures and overdose.
It is well documented that some antibiotics such as fluoroquinolones and tetracycline will bind to iron, calcium, calcium-fortified foods, and antacids if given concomitantly (1,2). This insoluble complex puts the patient at risk for potential of treatment failure based on little or no systemic absorption and may lead to the development of resistant organisms. Phenytoin is another example wherein orally administered drug may also bind to heavy metals, as well as enteral tube feedings (3,4). When these interactions go unrecognized, subtherapeutic phenytoin levels and a subsequent loss of seizure control may result. To best avoid these interactions, the iron, calcium, and antacids must be given either 2 hours before or 2 hours after the dose of the object drug. It is also suggested that continuous enteral feeding be halted for 2 hours before and after phenytoin administration.
Cholestyramine and sucralfate will physically bind other medications such as fluoroquinolones, ketoconazole, phenytoin, warfarin, valproic acid, and digoxin. In some circumstances, administering the drug 2 hours before cholestyramine or sucralfate and monitoring for effects will be adequate. In other cases, the combination may be best avoided entirely, such as with cholestyramine and warfarin.
Erythromycin, a macrolide antibiotic, is known to increase gut motility and, in recent years, has been exploited for this property as an alternative to cisapride. Cisapride was removed from the market secondary to life-threatening arrhythmias and torsades de pointes that occurred when combined with other drugs that inhibited the cytochrome P3A4 isoenzyme, depleted electrolytes, or prolonged QT interval. Historically there was concern in regard to the impact of increasing gut motility being tied to a potential decrease in the extent of drug absorption—for example, it was reported that the bioavailability of a digoxin tablet formulation (not Lanoxin) was reduced when taken concomitantly with metoclopramide therapy (5). More recently, however, reports of the opposite effect have surfaced where tacrolimus toxicity was associated with concomitant metoclopramide therapy (6). This drug–drug interaction is likely the result of enhanced
absorption of tacrolimus secondary to metoclopramide, whereby coadministration of the metoclopramide substantially improves gastric motility and promotes delivery of tacrolimus to the absorption sites in the small intestine. Drugs that delay gastric emptying will usually slow the transition of a drug into the small intestine, thus delaying and possibly decreasing absorption.
absorption of tacrolimus secondary to metoclopramide, whereby coadministration of the metoclopramide substantially improves gastric motility and promotes delivery of tacrolimus to the absorption sites in the small intestine. Drugs that delay gastric emptying will usually slow the transition of a drug into the small intestine, thus delaying and possibly decreasing absorption.
Other interactions can be exploited to enhance absorption of a drug. Didanosine liquid is prepared with antacid suspension to ensure adequate pH for optimal stability. The role of pH in the absorption of ketoconazole is well described, an acidic medium being required for dissolution and subsequent absorption. Administration of ketoconazole in the presence of proton-pump inhibitors, H2-blockers, or antacids severely hinders ketoconazole bioavailability. Ferrous sulfate is converted to the more absorbable ferric state in the presence of vitamin C. Many medications should be taken with food to enhance absorption, whereas some drugs should be given on an empty stomach. Often, the presence of food will delay the absorption but not impact the overall bioavailability of the drug. Consistency, either with or without food, should be the aim for patients taking medications with the potential for fluctuations in serum levels and resultant toxicities such as phenytoin, propranolol, and warfarin.
Intestinal flora plays an important role in body homeostasis. These bacteria, present widely in the large intestine, may have a role in drug interactions for medications that are recirculated back into the intestine after initial absorption, as in the case of oral contraceptives. It is understood that oral contraceptives are absorbed undergoing a significant first-pass effect, are conjugated in the liver, and are subsequently eliminated via the bile. Intestinal flora hydrolyzes the eliminated drug, yielding free estrogen that can then be reabsorbed. The presence of the intestinal flora is essential to maintain adequate serum concentrations of estrogen to prevent pregnancy. Although there is some controversy regarding this interaction, when a woman taking oral contraceptives is also subject to antibiotic treatment, if intestinal flora is compromised, the estrogen level may drop putting her at risk for unintended pregnancy (7).
In addition, P-glycoprotein, a genetically encoded and widely distributed drug efflux pump, has been described in significant drug interactions. P-glycoprotein is highly expressed in normal tissues, including the columnar epithelial cells in the intestine (8). Induction and inhibition of intestinal P-glycoprotein is reported to play a role in several drug interactions (8). The specific roles of P-glycoprotein in interactions are complex and are still being elucidated. For example, paclitaxel bioavailability rises in the presence of ciclosporin, a potent inhibitor or P-glycoprotein, suggesting that P-glycoprotein serves as an intestinal barrier preventing paclitaxel absorption. Likewise, P-glycoprotein inhibition by verapamil has been associated with improved digoxin absorption and elevated digoxin levels (8).
Drug interactions occurring during the administration phase are not only limited to the oral route. Intravenous aminoglycoside antibiotics can be inactivated if given within 30 minutes of a penicillin derivative. Postexposure prophylaxis that requires both passive immune globulin and active vaccine immunization should be given at distinct injection sites on different extremities to avoid diminishing the immune response to the vaccine.
Similar to taking advantage of enteral drug interactions to enhance a drug effect, injectable drug interactions can at times be useful. Epinephrine, a potent vasoconstrictor will decrease blood flow to an area. When added to a local anesthetic during laceration treatment, the epinephrine creates a more visible working environment. The lack of blood flow also enhances the efficacy by decreasing the absorption of the local anesthetic.
Distribution
Distribution of medications is dependent upon total body water, extracellular fluid, percentage of adipose tissue, and the capacity to bind to plasma proteins. Albumin and α-1 glycoprotein are the primary circulating plasma proteins to which drugs bind. Albumin is the predominant plasma protein and binds in particular to acidic and neutral drugs, whereas α-1 glycoprotein preferentially binds basic drugs (9). Variations in plasma protein concentration are seen secondary to certain disease states and can influence free drug concentration. Drug interactions occur because of competition for binding sites on these proteins. In effect, one drug displaces the other off the binding site, or alternatively, occupies the site, not allowing the other drug to bind.
An interaction will not be clinically significant unless the involved drugs are highly protein bound (>95%) or have very narrow therapeutic indices. A classic example is phenytoin, which not only is involved in physical binding interactions discussed previously, as well as cytochrome P450 interactions to be discussed later, but also is generally between 89% to 93% protein bound with a very narrow therapeutic window. When phenytoin is given in conjunction with salicylates or valproic acid, the free fraction of phenytoin increases because of competition for the same plasma protein binding sites. Should the free fraction of phenytoin rise above 2 μg per dL, toxicity is generally seen consisting of ataxia, nystagmus, increased seizure activity, and if high enough, coma.
Metabolism
The liver is the most important organ involved in drug metabolism, although metabolism also takes place to a degree in the blood, GI tract, kidney, lung, skin, and placenta. Drug metabolism is divided into two categories, phase I (oxidation, reduction, hydrolysis) and phase II (conjugation) reactions. Phase I reactions generally result in a compound that is less toxic and more hydrophilic, allowing for efficient excretion. At times, phase I reactions lead to the metabolism of a parent compound or prodrug into the active metabolite (e.g., acetaminophen, methanol, enalapril). Mixed function oxidases, or the cytochrome P450 (CYP450) system of enzymes, are responsible for the bulk of Phase I reactions. Phase II reactions generally terminate the biologic activity of the drug and prepare it for elimination through conjugation with glucuronide, sulfate,
or glycine; although the compounds have a larger molecular weight, they are more water-soluble.
or glycine; although the compounds have a larger molecular weight, they are more water-soluble.
The CYP450 enzymes are unique isoenzymes found primarily in the liver and are responsible for the metabolism of many drugs and toxins. (Table 64.1) The enzymes are so named because of the absorption of light at a wavelength of 450 nm and are grouped into families 1, 2, and 3 and then divided into subfamilies A to E. The individual member enzymes are then further designated by a number (e.g., 3A4, 2D6). These enzymes are genetically encoded and therefore are associated with interpatient variability; this variability makes it difficult to predict who will experience an adverse reaction or exaggerated drug interaction. Age-related development of CYP450 enzymes matures and even surpasses adult capacity during the first year of life (10).
Inducers are drugs that are capable of increasing CYP450 enzyme activity by increasing enzyme synthesis. These drugs will enhance the enzyme’s metabolizing capacity, speeding up substrate drug metabolism, and decreasing the object drug effect. The time onset of enzyme induction is dependent upon the half-life of the inducing drug. An inducer such as phenobarbital, with a long half-life can take up to a week to have an impact. In contrast, a decrease in the concentration of a drug metabolized by CYP2C9 can occur within 24 hours after the initiation of rifampin, a rapid and potent inducer (11).
The inhibition of CYP450 enzymes may occur secondary to competitive binding between two drugs or to permanent inactivation (12). Generally, inhibition begins after the first dose of the inhibitor and the duration of inhibition correlates with the half-life of the drug. Most commonly these interactions result in the slow down of substrate drug metabolism, yielding increased plasma concentrations, increased drug effect, and thereby increased risk of adverse events. Drugs have been intentionally combined to exploit CYP450 inhibition; the protease inhibitor ritonavir, a potent CYP3A4 inhibitor, is added to lopinavir and elvitegravir to boost serum levels in patients with human immunodeficiency virus (13,14).
Cytochrome P1A2
Nearly 15% of medications used today are metabolized by cytochrome P1A2 (CYP1A2), most notably caffeine, theophylline lidocaine, tricyclic antidepressants, and warfarin. The activity of CYP1A2 can be induced by cigarette smoke, charbroiled foods, and cruciferous vegetables (e.g., cabbage, broccoli). Several medications can also affect CYP1A2 activity. Phenobarbital, carbamazepine, and rifampin induce CYP1A2 as well as several other enzymes, leading to clinically relevant drug interactions. Inhibitors of CYP1A2 include cimetidine, ciprofloxacin, erythromycin, fluvoxamine, and grapefruit juice. Omeprazole and ritonavir simultaneously induce CYP1A2 and inhibit one or more other enzymes.
Cytochrome 2B6
The significance of cytochrome 2B6 (CYP2B6) in drug metabolism has only recently become apparent. CYP2B6 is genetically polymorphic and is implicated in the metabolism of a growing number of clinically important drugs. It is estimated that nearly 8% of drugs on the market are metabolized via CYP2B6 (15). Clinically used drugs that are known to be metabolized by CYP2B6 include chemotherapeutics such as cyclophosphamide, ifosfamide, the antiestrogen tamoxifen, as well as efavirenz, ketamine, propofol, and diazepam (16,17). Ritonavir, a protease inhibitor, is known to be a CYP2B6 inducer, which may explain the increased elimination of several drugs as demonstrated with bupropion (18). Genetic polymorphisms occur in approximately 3% of whites and up to 20% of blacks, being poor metabolizers, this seems to predict a more complicated clinical course and greater risk of toxicity-driven drug discontinuation (15).
Cytochrome 2C8
Cytochrome 2C8 (CYP2C8) has been studied to a lesser extent than its familial counterparts. Comprising approximately 7% of total microsomal CYP content in the liver, it caries out oxidative metabolism in nearly 5% of drugs cleared via phase I processes (19). Therapeutic agents metabolized by CYP2C8 include paclitaxel, amiodarone, rosiglitazone, cerivastatin, and fluvastatin. Known inhibitors of CYP2C8 consist of trimethoprim, gemfibrozil, montelukast, and ketoconazole, as well as several known CYP3A4 substrates—amitriptyline, terfenadine, and triazolam—causing up to 50% inhibition of CYP2C8 activity (19). Several genetic polymorphisms have been identified with CYP2C8, the most common of which is seen in roughly 18% of blacks and rarely in whites.
Cytochrome P2C9
Cytochrome P2C9 (CYP2C9) is responsible for the metabolism of several common medications including ibuprofen, carvedilol, celecoxib, losartan, phenytoin, glyburide, and warfarin (11). Rifampin and rifabutin are powerful inducers of CYP2C9 activity and will therefore decrease serum concentrations of the above substrates. Other inducers include carbamazepine, ethanol, and phenobarbital. Warfarin is produced as a racemic mixture of R-warfarin and S-warfarin, with the S-enantiomer being responsible for the predominance of pharmacologic activity. S-warfarin is principally metabolized via CYP2C9; inhibition of this enzyme pathway results in clinically significant drug–drug interactions, such that the Food and Drug Administration approved updated labeling for warfarin in 2007 remarking on this pharmacogenetic issue (20). Known inhibitors of CYP2C9 include fluconazole, ketoconazole, metronidazole, amiodarone, lovastatin, and even a number of flavonoids, secondary metabolites of many plants consumed by humans in a typical diet (21). Genetic polymorphisms occur in up to one-third of whites, contributing to abnormally decreased enzyme activity in these individuals (22).
Cytochrome P2C19
Like several other monoamine oxidases, cytochrome P2C19 (CYP2C19) has been shown to exhibit a genetic polymorphism; 3% to 6% of Caucasians, 15% to 20% of Japanese,
and 10% to 20% of African Americans are poor metabolizers (23,24). Medications metabolized by CYP2C19 include several benzodiazepines, citalopram, tricyclic antidepressants, omeprazole, and warfarin, as well as the antiepileptics phenytoin, phenobarbital, and valproic acid (25,26). The isoenzyme is of major relevance to anticancer drug and antiepileptic drug interactions. Induction or inhibition of CYP2C19 can cause a decrease in anticancer drug concentrations. Similarly, enzyme inhibition or induction by anticancer drugs may lead to toxicity or loss of seizure control. This complex relationship is important particularly in the treatment of patients with brain cancer (22). Known inducers of CYP2C19 include carbamazepine, phenytoin, rifampin, and phenobarbital, whereas inhibitors consist of fluoxetine, sertraline, fluvoxamine, omeprazole, ritonavir, and isoniazid.
and 10% to 20% of African Americans are poor metabolizers (23,24). Medications metabolized by CYP2C19 include several benzodiazepines, citalopram, tricyclic antidepressants, omeprazole, and warfarin, as well as the antiepileptics phenytoin, phenobarbital, and valproic acid (25,26). The isoenzyme is of major relevance to anticancer drug and antiepileptic drug interactions. Induction or inhibition of CYP2C19 can cause a decrease in anticancer drug concentrations. Similarly, enzyme inhibition or induction by anticancer drugs may lead to toxicity or loss of seizure control. This complex relationship is important particularly in the treatment of patients with brain cancer (22). Known inducers of CYP2C19 include carbamazepine, phenytoin, rifampin, and phenobarbital, whereas inhibitors consist of fluoxetine, sertraline, fluvoxamine, omeprazole, ritonavir, and isoniazid.
Table 64.1 Cytochrome P450 Substrates, Inhibitors, and Inducers | ||||||||||||||||||||||||||||||||||||||||||||||||||||||||||||||||||||||||||||||||||||||||||||||||||||||||||||||||||||||||||||||||||||||||||||||||||||||||||||||||||||||||||||||||||||||||||||||||||||||||||||||||||||||||||||||||||||||||||||||||||||||||||||||||||||||||||||||||||||||||||||||||||||||||||||||||||||||||||||||||||||||||||||||||||||||||||||||||||||||||||||||||||||||||||||||||||||||||||||||||||||||||||||||||||||||||||||||||||||||||||||||||||||||||||||||||||||||||||||||||||||||||||||||||||||||||||||||||||||||||||||||||||||||||||||||||||||||||||||||||||||||||||||||||||||||||||||||||||||||||||||||||||||||||||||||||||||||||||||||||||||||||||||||||||||||||||||||||||||||||||||||||||||||||||||||||||||||||||||||||||||||||||||||||||||||||||||||||||||||||||||||||||||||||||||||||||||||||||||||||||||||||||||||||||||||||||||
---|---|---|---|---|---|---|---|---|---|---|---|---|---|---|---|---|---|---|---|---|---|---|---|---|---|---|---|---|---|---|---|---|---|---|---|---|---|---|---|---|---|---|---|---|---|---|---|---|---|---|---|---|---|---|---|---|---|---|---|---|---|---|---|---|---|---|---|---|---|---|---|---|---|---|---|---|---|---|---|---|---|---|---|---|---|---|---|---|---|---|---|---|---|---|---|---|---|---|---|---|---|---|---|---|---|---|---|---|---|---|---|---|---|---|---|---|---|---|---|---|---|---|---|---|---|---|---|---|---|---|---|---|---|---|---|---|---|---|---|---|---|---|---|---|---|---|---|---|---|---|---|---|---|---|---|---|---|---|---|---|---|---|---|---|---|---|---|---|---|---|---|---|---|---|---|---|---|---|---|---|---|---|---|---|---|---|---|---|---|---|---|---|---|---|---|---|---|---|---|---|---|---|---|---|---|---|---|---|---|---|---|---|---|---|---|---|---|---|---|---|---|---|---|---|---|---|---|---|---|---|---|---|---|---|---|---|---|---|---|---|---|---|---|---|---|---|---|---|---|---|---|---|---|---|---|---|---|---|---|---|---|---|---|---|---|---|---|---|---|---|---|---|---|---|---|---|---|---|---|---|---|---|---|---|---|---|---|---|---|---|---|---|---|---|---|---|---|---|---|---|---|---|---|---|---|---|---|---|---|---|---|---|---|---|---|---|---|---|---|---|---|---|---|---|---|---|---|---|---|---|---|---|---|---|---|---|---|---|---|---|---|---|---|---|---|---|---|---|---|---|---|---|---|---|---|---|---|---|---|---|---|---|---|---|---|---|---|---|---|---|---|---|---|---|---|---|---|---|---|---|---|---|---|---|---|---|---|---|---|---|---|---|---|---|---|---|---|---|---|---|---|---|---|---|---|---|---|---|---|---|---|---|---|---|---|---|---|---|---|---|---|---|---|---|---|---|---|---|---|---|---|---|---|---|---|---|---|---|---|---|---|---|---|---|---|---|---|---|---|---|---|---|---|---|---|---|---|---|---|---|---|---|---|---|---|---|---|---|---|---|---|---|---|---|---|---|---|---|---|---|---|---|---|---|---|---|---|---|---|---|---|---|---|---|---|---|---|---|---|---|---|---|---|---|---|---|---|---|---|---|---|---|---|---|---|---|---|---|---|---|---|---|---|---|---|---|---|---|---|---|---|---|---|---|---|---|---|---|---|---|---|---|---|---|---|---|---|---|---|---|---|---|---|---|---|---|---|---|---|---|---|---|---|---|---|---|---|---|---|---|---|---|---|---|---|---|---|---|---|---|---|---|---|---|---|---|---|---|---|---|---|---|---|---|---|---|---|---|---|---|---|---|---|---|---|---|---|---|---|---|---|---|---|---|---|---|---|---|---|---|---|---|---|---|---|---|---|---|---|---|---|---|---|---|---|---|---|---|---|---|---|---|---|---|---|---|---|---|---|---|---|---|---|---|---|---|---|---|---|---|---|---|---|---|---|---|---|---|---|---|---|---|---|---|---|---|---|---|---|---|---|---|---|---|---|---|---|---|---|---|---|---|---|---|---|---|---|---|---|---|---|---|---|---|---|---|---|---|---|---|---|---|---|---|---|---|---|---|---|---|---|---|---|---|---|---|---|---|---|---|---|---|---|---|---|---|---|---|---|---|---|---|---|---|---|---|---|---|---|---|---|---|---|---|---|---|---|---|---|---|---|---|---|---|---|---|---|---|---|---|---|---|---|---|---|---|---|---|---|---|---|---|---|---|---|---|---|---|---|---|---|---|---|---|---|---|---|---|---|---|---|---|---|---|---|---|---|---|---|---|---|---|---|---|---|---|---|---|---|---|---|---|---|---|---|---|---|---|
|
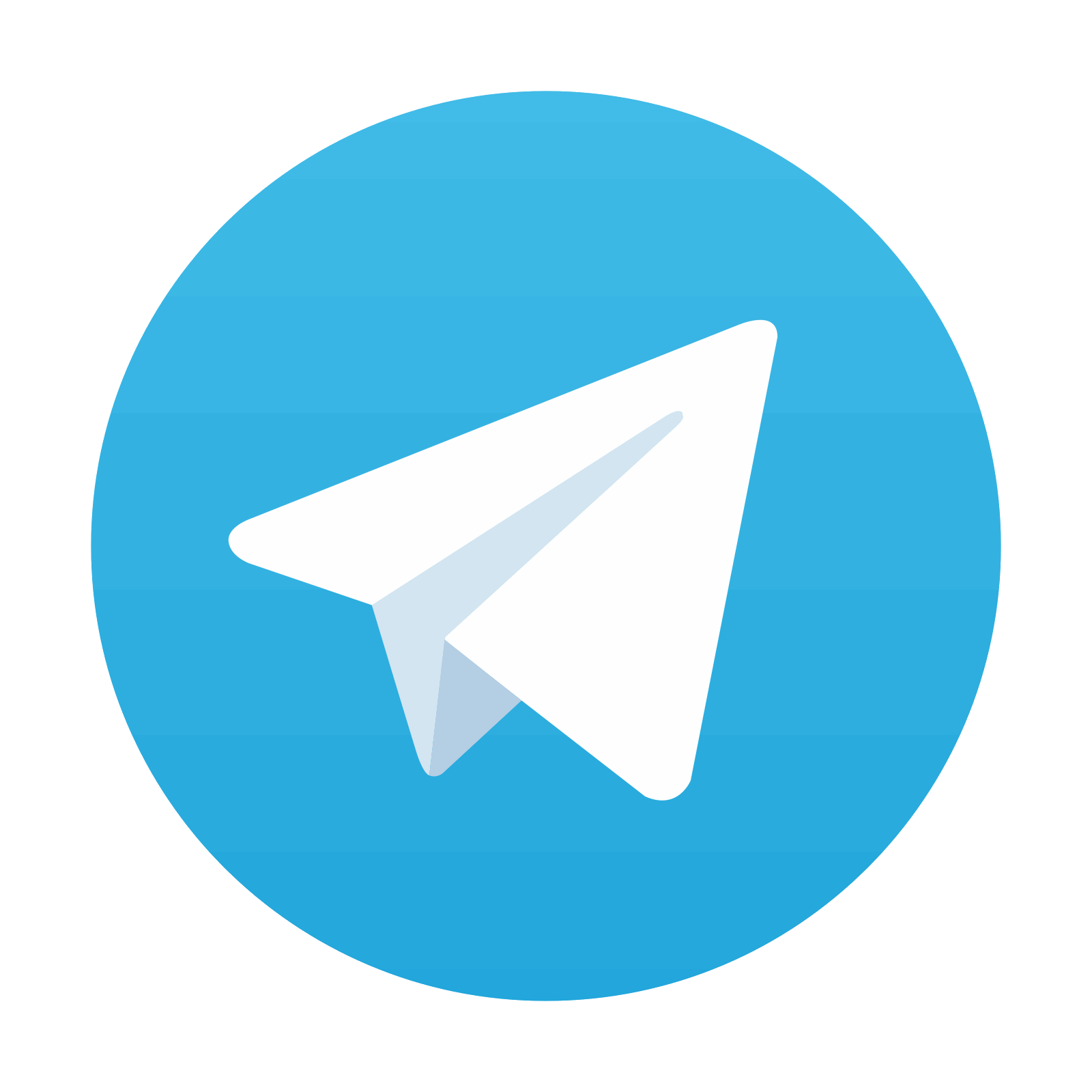
Stay updated, free articles. Join our Telegram channel

Full access? Get Clinical Tree
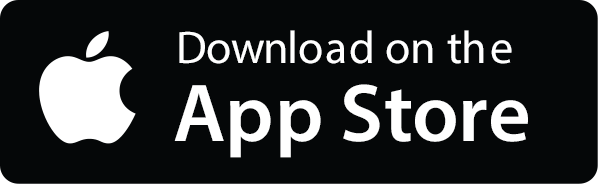
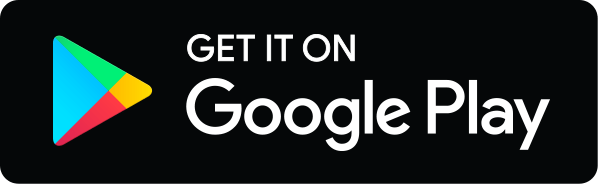