Children with Down syndrome (DS) and acute leukemias acute have unique biological, cytogenetic, and intrinsic factors that affect their treatment and outcome. Myeloid leukemia of Down syndrome (ML-DS) is associated with high event-free survival (EFS) rates and frequently preceded by a preleukemia condition, the transient abnormal hematopoiesis (TAM) present at birth. For acute lymphoblastic leukemia (ALL), their EFS and overall survival are poorer than non-DS ALL, it is important to enroll them on therapeutic trials, including relapse trials; investigate new agents that could potentially improve their leukemia-free survival; and strive to maximize the supportive care these patients need.
Key points
- •
Children with Down syndrome manifest multiple hematologic manifestations: (1) transient myeloproliferative disorder (TMD)/transient abnormal myelopoiesis (TAM) at birth, (2) acute myeloid leukemia (ML-DS), and (3) acute lymphoblastic leukemia (ALL).
- •
The underlying primary basis for the varied hematologic manifestations is linked to the gene dosage effect of chromosome 21–encoded genes.
- •
TMD/TAM and ML-DS are characterized by the presence of truncating mutations in exon 2 of the hematopoietic transcription factor GATA1 . Spontaneous resolution is common in TMD/TAM, and ML-DS is highly responsive to chemotherapy with resultant high cure rates compared with acute myeloid leukemia in non-DS children.
- •
DS-ALL is characterized by the presence of mutations in JAK2 tyrosine kinase and IKZF1 . In contrast to the high cure rates in ML-DS, the results in DS-ALL are same are inferior to non-DS ALL, in part, because of the lower frequency of good-response ALL subtypes and also the higher systemic toxicity of agents used in children with DS.
Introduction
For more than 150 years, Down syndrome (DS) has been linked to the English physician John Langdon Down. His essay published in 1866, “Observations on an Ethnic Classification of Idiots,” described a group of cognitively impaired individuals with common physical features. DS is now recognized as the most common chromosomal abnormality, occurring in 1 in every 800 to 1000 live births. Neonates, children, and adults with DS develop multiple medical disorders; hematologic disorders being one of the most well known. It has long been recognized that children with constitutional trisomy 21 (DS) have a markedly increased risk of acute leukemia. The first description of a child with DS who developed acute leukemia was published in 1930. Subsequently, a national survey in the United States provided support to the notion that children with DS had an increased risk of developing leukemia. Remarkably, children with DS are at an increased risk both of acute megakaryocyte-erythroid leukemia (known as myeloid leukemia of DS [ML-DS]) by 150-fold and of acute B-lineage lymphoblastic leukemia by 33-fold compared with children without DS.
In ML-DS, it is now clear that the initial event is perturbation of fetal hemopoiesis by trisomy 21 (T21) itself. This perturbation leads to complex defects in fetal hemopoiesis and newborn hematology. In up to 28% of fetuses/newborns with DS, hemopoietic cells acquire mutations in the gene encoding the key megakaryocyte-erythroid transcription factor GATA1. Acquisition of GATA1 mutations can either be clinically silent or result in a clinically important preleukemic fetal/neonatal disorder transient abnormal myelopoiesis (TAM). Most cases of TAM resolve without long-term clinical sequelae; but in a proportion of cases with TAM, neonates/young children acquire additional genetic mutations that immortalize the TAM clone and result in frank ML-DS. There are parallel defects in DS fetal B-cell lymphopoiesis caused by T21 that most likely result in acquisition of a series of cooperating and transforming mutations in genes encoding key regulators of B-lymphopoiesis (eg, JAK2 and CLRF2 ).
Thus, the unique features of DS-associated leukemias arise because of the crucial role played by T21 that then creates the right cellular and molecular environment for the acquisition of additional genetic mutations that together lead to acute leukemia. Thus, DS-associated leukemias represent potentially one of the most tractable human models to understand the biological basis of multistep leukemogenesis and the impact of aneuploidy on cancer. Next, the authors highlight some of the recent clinical and biological advances in these preleukemic and leukemic conditions.
Introduction
For more than 150 years, Down syndrome (DS) has been linked to the English physician John Langdon Down. His essay published in 1866, “Observations on an Ethnic Classification of Idiots,” described a group of cognitively impaired individuals with common physical features. DS is now recognized as the most common chromosomal abnormality, occurring in 1 in every 800 to 1000 live births. Neonates, children, and adults with DS develop multiple medical disorders; hematologic disorders being one of the most well known. It has long been recognized that children with constitutional trisomy 21 (DS) have a markedly increased risk of acute leukemia. The first description of a child with DS who developed acute leukemia was published in 1930. Subsequently, a national survey in the United States provided support to the notion that children with DS had an increased risk of developing leukemia. Remarkably, children with DS are at an increased risk both of acute megakaryocyte-erythroid leukemia (known as myeloid leukemia of DS [ML-DS]) by 150-fold and of acute B-lineage lymphoblastic leukemia by 33-fold compared with children without DS.
In ML-DS, it is now clear that the initial event is perturbation of fetal hemopoiesis by trisomy 21 (T21) itself. This perturbation leads to complex defects in fetal hemopoiesis and newborn hematology. In up to 28% of fetuses/newborns with DS, hemopoietic cells acquire mutations in the gene encoding the key megakaryocyte-erythroid transcription factor GATA1. Acquisition of GATA1 mutations can either be clinically silent or result in a clinically important preleukemic fetal/neonatal disorder transient abnormal myelopoiesis (TAM). Most cases of TAM resolve without long-term clinical sequelae; but in a proportion of cases with TAM, neonates/young children acquire additional genetic mutations that immortalize the TAM clone and result in frank ML-DS. There are parallel defects in DS fetal B-cell lymphopoiesis caused by T21 that most likely result in acquisition of a series of cooperating and transforming mutations in genes encoding key regulators of B-lymphopoiesis (eg, JAK2 and CLRF2 ).
Thus, the unique features of DS-associated leukemias arise because of the crucial role played by T21 that then creates the right cellular and molecular environment for the acquisition of additional genetic mutations that together lead to acute leukemia. Thus, DS-associated leukemias represent potentially one of the most tractable human models to understand the biological basis of multistep leukemogenesis and the impact of aneuploidy on cancer. Next, the authors highlight some of the recent clinical and biological advances in these preleukemic and leukemic conditions.
Trisomy 21 and human fetal hematopoiesis
Fetal Origin of Trisomy 21–Associated Leukemias
Human T21 itself perturbs second-trimester fetal liver hemopoietic stem/progenitor (HSPC) function. T21 increases the frequency of immunophenotypic hemopoietic stem cell (HSC) that has a biased erythroid-megakaryocyte primed gene expression profile compared with disomic HSC. Furthermore, multiple HSPC populations show increased megakaryocyte-erythroid output in colony assays. Coupled with this, there is an expansion of megakaryocyte-erythroid progenitors themselves. Consistent with this increased megakaryocyte output, immunohistochemical studies of T21 fetal liver sections show increased megakaryocyte numbers. However, megakaryocyte differentiation may be compromised, as fetal liver megakaryocytes are morphologically abnormal. Corroborating data from human T21 embryonic stem cells and induced pluripotent stem cells show increased erythroid and possible megakaryocyte production. In addition to increased, but likely perturbed megakaryocyte-erythroid differentiation, there is a severe impairment of B-lymphoid development in DS fetal liver; with an approximately 10-fold reduction in pre-pro B cells and B-cell potential of HSC, in tandem with reduced HSC lymphoid gene expression priming.
Abnormalities of Blood Counts in Newborns with Down Syndrome
Fetal liver hemopoietic defects most likely result in multi-lineage blood count changes in newborns with DS. These changes were initially noted in several small retrospective studies and, more recently, in a recent large prospective study. Neonates with DS had higher hemoglobin concentrations, increased circulating erythroblasts, and abnormal red cell morphology, including macrocytosis, target cells, and basophilic stippling. Median platelet counts were also lower than normal in neonates with DS. Thrombocytopenia was common; although the median mean platelet volume was similar to neonates without DS, platelet morphology was abnormal (giant platelets, circulating megakaryocytes, and/or megakaryocyte fragments) in greater than 95% of cases. Neonates with DS had higher numbers of granulocytes and monocytes despite the reduction in granulocyte-monocyte progenitors in fetal liver. This finding may reflect a greater reliance on bone marrow hematopoiesis in late gestation and after birth. The total lymphocyte count (and particularly B-lymphocyte count) was reduced in the neonates with DS.
Molecular Basis for Perturbed Fetal/Neonatal Hematology Caused by Trisomy 21
The molecular basis for trisomy 21-mediated abnormal hematopoiesis is still largely unclear. The complex HSPC and peripheral defects suggest multiple genes are likely to be involved at distinct fetal stages of hematopoiesis. These genes could include the approximately 300 protein- and RNA-encoding on chromosome 21 through gene dosage and/or more global impact on disomic gene regulation. At least 2 approaches have been used to identify genes linked to increased leukemia susceptibility. First, studies in rare patients and mouse models have tried to narrow down the anatomic region of chromosome 21 responsible for the hemopoietic phenotype. Occasional patients with partial trisomy suggest that the leukemia risk is confined to an 8.5-Mb region on chromosome 21, although conclusions here are limited by the very small number of cases of leukemia identified in these patients. The study of murine models trisomic for a variable number of human chromosome 21 genes has provided some insight but are somewhat limited, as none fully recapitulates the human hemopoietic phenotype in fetal and neonatal human DS. In summary, overexpression of the genes ERG , DYRK1A , CHAF1B , and HLCS have all been implicated in a late-onset adult myeloproliferative disorder and in facilitating the transformation to acute myeloid leukemia (AML). Second, analysis of gene expression in primary T21 fetal liver samples and hemopoietic cells derived from human T21 embryonic stem cells or induced pluripotent cells suggest that increased expression of several genes may contribute to deregulated hemopoiesis. These genes include RUNX1 , BACH1 , ETS2 , ERG , DYRK1A, GABPA , and SON . However, careful functional experiments will be central to understanding if altered expression of any gene is causal to the T21 hemopoietic phenotype.
In addition, it is unclear if the T21 phenotype is hemopoietic cell autonomous or is, in part, mediated by cells constituting the hemopoietic microenvironment. Several lines of indirect evidence suggest that fetal liver may provide the specialized microenvironment necessary for instigating and/or maintaining abnormal hematopoiesis in DS. First, as mentioned earlier, the observation that abnormal hemopoiesis occurs in fetal life suggests a yolk sac/fetal microenvironment is important. Second, differential production and responsiveness of fetal tissues, including hematopoietic cells, to insulin-like growth factors is one of the few consistently reported differences between adult and fetal hematopoiesis.
Transient abnormal myelopoiesis: myeloid preleukemia of Down syndrome
Clinical Findings
Although TAM is usually seen in newborns with DS, 7% to 16% of TAM cases have mosaic T21. TAM can also present as hydrops fetalis with hepatosplenomegaly. The current definition of TAM is imprecise. It is defined as the presence of circulating blood blasts in a baby with typical clinical features of TAM who may or may not have hematologic abnormalities. Previous studies have used differing clinical and/or hematologic criteria to define TAM. At one end of the spectrum, TAM is detected as an incidental finding on review of a blood smear in an otherwise well baby. Often there will be an associated mild leucocytosis and thrombocytopenia but these findings are also common in neonates with DS who do not have TAM. At the other of the spectrum, babies can be extremely sick with bruising, ascites, jaundice, hepatosplenomegaly, respiratory distress associated with pleural effusions, and a low cardiac output state associated with a pericardial effusion. In one series, 25% of the babies with TAM were referred to an intensive care unit.
Transient Abnormal Myelopoiesis: Laboratory Findings
Laboratory tests (reviewed in ) can show leucocytosis (20%–30% of cases with a mean white cell count of 20–40 × 10 9 /L, with occasional cases having a white count of >100 × 10 9 /L), though. There is variable thrombocytopenia in approximately 40% of cases (mean 80–100 × 10 9 /L) but thrombocytopenia is not more common in TAM than in neonates with DS who do not have TAM and occasionally severe anemia (hemoglobin <8 g/dL) is seen. Basophilia and an eosinophilia are often seen. Blasts often, but not invariably, have the appearance of megakaryoblasts (with cytoplasmic blebbing). Flow cytometry of blasts may be helpful, as they often express CD34, CD38, CD117, CD7, CD45, the erythroid antigen CD235, the megakaryocyte antigens CD41, CD42b, and CD61 and CD56 and almost always express CD33. The bone marrow aspirate is not often helpful. The cellularity can be increased, normal, or decreased. There is a deranged coagulation profile in 20% to 25% of cases, with disseminated intravascular coagulation (DIC) in approximately 7% to 10% of cases. Hepatic dysfunction is usually associated with hyperbilirubinemia, ascites, elevated transaminases, and hepatic infiltration with blasts. In more severe cases, there is hepatic fibrosis.
GATA1 Mutation, Definition of Transient Abnormal Myelopoiesis and Silent Transient Abnormal Myelopoiesis, and Identification of Children at Risk of Myeloid Leukemia of Down Syndrome
A diagnostic test in nearly many cases have a normal white cell count babies with TAM is the presence of acquired N-terminal truncating mutations and, more rarely internal in frame deletions, within exon 2 and, occasionally, exon 3 in the gene encoding the megakaryocyte-erythroid transcription factor GATA1. GATA1 mutations are required for TAM. The detection of N-terminal truncating GATA1 mutations also extends to cases of TAM in neonates with DS mosaicism. There is a unique association with these types of GATA1 mutation and leukemogenesis in hemopoietic cells with T21. Although rare, families and individuals have been described with N-terminal truncating GATA1 mutations in non-DS individuals; there is no documented increased risk of AML in these cases. Non-DS patients with N-terminal truncating GATA1 mutations present with a variety of hematologic phenotypes, including multiple cytopenias and Diamond-Blackfan anemia phenotype.
The incidence of TAM has been the subject of debate. Retrospective studies suggest TAM affects approximately 10% of neonates with DS; but these studies have used differing clinical and/or hematologic criteria to define TAM, none of which is specific for TAM. Importantly, no retrospective studies have systematically screened neonates for GATA1 mutations, a genetic marker specific for TAM and ML-DS. Thus, current definitions of TAM neither specify the percentage of blasts considered abnormal in DS neonates nor the role of GATA1 mutation analysis in the diagnosis. As a result, asymptomatic TAM may be missed in some neonates, as blood counts and smears are often not performed. However, in others babies, TAM may be overdiagnosed by relying on nonspecific clinical and hematologic features. This possibility is borne out by the only large systematic GATA1 mutation screen, performed by Sanger sequencing of genomic DNA extracted from dried blood spots, which found a prevalence of GATA1 mutations in neonates with DS of only 3.8%, in contrast to the 5% to 10% prevalence of TAM diagnosed by clinical and hematologic criteria (see review by ).
The perception of the incidence and definition of TAM has been changed by a recent prospective study that systematically documented clinical features, blood counts, blood smears, and GATA1 mutation in a large population-based cohort of newborns with DS. Surprisingly, 195 out of 200 (97.5%) neonates with DS had circulating blasts (range: 1%–77%). In approximately 8.5% of cases, the blast count was greater than 10%; a GATA1 mutation was detected by conventional Sanger sequencing and mutation detection techniques (direct high-pressure liquid chromatography [DHPLC]). In a further approximately 20% of babies with blast counts less than 10%, GATA1 mutations were detected by the more sensitive next-generation sequencing mutation detection. No clinical or hematologic features distinguished these neonates from GATA1 mutation-negative neonates with DS or cases of TAM. Thus, GATA1 mutations are common, occur in approximately 28% of all neonates with DS, but are often unsuspected and detectable only with sensitive methods. The authors suggest TAM is defined as blasts greater than 10% and a GATA1 mutation detected by conventional sequencing and/or DHPLC. The authors suggest the term silent TAM for those with a GATA1 mutation detectable only by more sensitive sequencing techniques. In the authors’ series, both TAM and silent TAM can transform to ML-DS. Longer-term follow-up is required to determine rates of transformation. To diagnose silent TAM and TAM, an initial evaluation of blood smears as well as full blood counts, as recommended by the American Academy of Pediatrics, is a useful, immediate screening step to identify neonates with DS with classic TAM who may require early treatment (especially where GATA1 analysis is unavailable or delayed). If possible, all neonates with DS should also have GATA1 mutation analysis by Sanger sequencing and DHPLC to quickly identify those with large mutant GATA1 clones. For neonates with DS without mutations by Sanger sequencing or DHPLC, next-generation resequencing can be used to identify those with small mutant GATA1 clones. By comprehensively detecting GATA1 mutations, pediatric hematology follow-up can be limited to those at risk of transformation rather than all babies with blasts (ie, almost all babies with DS).
Outcome and Management of Transient Abnormal Myelopoiesis
In most babies (80%–90%), the condition only requires observation and blood count monitoring, as it resolves spontaneously over a 1- to 3-month period (median time to resolution 47 days ). However, a proportion of babies require therapy for clinical symptoms (eg, cardiac failure or DIC). However, it is unclear which infants will benefit from treatment and what the most effective treatment is. Accordingly, treatment policies vary considerably between centers. Both the US Pediatric/Children’s Oncology Group (POG/COG) and the German International Berlin-Frankfurt-Munster (BFM) group have developed treatment guidelines for TAM that can be instituted at the physician’s discretion.
As TAM blasts are highly sensitive to cytarabine, it is the basis of cytoreductive therapy. The response is generally rapid with disappearance of peripheral blasts within 7 days of treatment. However, in babies with severe liver disease associated with fibrosis, the response to chemotherapy is poor. In the POG study 9481, cytosine arabinoside (araC) was given either as 10 mg/m 2 per dose or 1.2 to 1.5 mg/kg per dose subcutaneously or intravenously by slow injection twice a day for 7 days. In the AML-BFM study, 0.5 to 1.5 mg/kg was administered for 3 to 12 days. The higher dose of cytarabine (3.33 mg/kg/24 h given by continuous infusion for 5 days) used in the COG A2971 study is probably not required and was associated with significant toxicity. Despite therapy, 10% to 20% of babies die of TAM within 3 months with hepatic and renal failure and DIC. These babies often present with a higher white count (>100 × 10 9 /L), ascites, effusions, and coagulopathy and develop hepatic fibrosis.
Transformation to Myeloid Leukemia of Down Syndrome
Following the clinical resolution of TAM with normalization of blood counts either spontaneously or after treatment with very low-dose cytarabine, up to 30% of these neonates with clinical TAM will subsequently develop ML-DS. In a baby with a history of TAM, often the same GATA1 mutation is detected when the child presents with ML-DS proving that the two disorders are clonally linked. In approximately 15% to 25% of cases, multiple GATA1 mutations are detected in both TAM and ML-DS suggesting that multiple GATA1 mutant clones exist.
Clinical Features of Myeloid Leukemia of Down Syndrome
Children with ML-DS with DS represent approximately 15% of the pediatric AML cases. Reports from both the United Kingdom Childhood Cancer Study and the Children’s Cancer Group (CCG) identified that the mean age at diagnosis of ML-DS was lower than the age for children without DS (2.2 years versus 6.7 years and 1.8 versus 7.5 years, respectively). Overall, 95% of ML-DS cases are diagnosed before 4 years of age. Progression to ML-DS does not occur in children beyond 5 years of age.
Progression to ML-DS occurs at a variable tempo. In some children, progression is heralded by a period of falling blood counts, especially thrombocytopenia and leukopenia. Cytopenia may be short or prolonged over months before a formal diagnosis of ML-DS. It is often associated with dysplastic changes in peripheral blood cells. At presentation of ML-DS, children with DS may not seem acutely ill compared with children without DS. This circumstance is caused, in part, by closer clinical monitoring of these children based on their known increased risk to develop leukemia and early evidence of abnormalities in blood count parameters indicating evidence of potential progression to leukemia in the absence of clinical symptoms. These early signs of myelodysplasia are characterized by progressive anemia and thrombocytopenia in association with dysplastic erythroid cells and megakaryocytes in the bone marrow. This myelodysplastic phase frequently precedes the development of AML, and the spectrum of both myelodysplastic syndrome (MDS) and AML have been known collectively as ML-DS. Rarely ML-DS can progress directly from aggressive TAM. In these cases, the diagnosis is problematic, as it is difficult to distinguish between TAM that is not resolving and the development of ML-DS. A bone marrow aspirate and trephine can be helpful to confirm greater than 30% blasts. Often the blast count is lower as the marrow sample on aspirate is hemodilute. In these cases, the trephine shows marrow fibrosis echoing the liver fibrosis seen in some cases of TAM. If the marrow is aspirable or if there are circulating blasts, flow cytometry is valuable, as blasts express the same cell surface markers as TAM blasts.
Laboratory Features of Myeloid Leukemia of Down Syndrome
Several other distinctive differences exist in the biological features of ML-DS compared with AML in children without DS. Among the 8 different French-American-British subtypes of AML, children with DS have a disproportionately high proportion with the acute megakaryocytic/megakaryoblastic (AMKL, M7) phenotype. Zipursky estimated that children with DS have a 500-fold increased risk of developing ML-DS compared with children without DS, with some clinical studies identifying the ML-DS phenotype in more than 90% of ML-DS cases. Besides the morphologic identification of the ML-DS phenotype, megakaryoblasts are identified by their immunophenotype expression of the platelet-associated membrane antigens (glycoprotein IIb/IIIa) using CD41/61 antibodies and frequently the aberrant expression of the T-cell antigen, CD7, and the thrombospondin receptor, CD36. High expression of CD36 marker distinguishes ML-DS from most cases of de novo AMKL and may be a surrogate for the developmental stage of the megakaryoblasts identified by molecular studies.
The proportion of patients with DS who fit the criteria of MDS based on the bone marrow involvement containing less that 30% blasts was approximately 30% for both the COG A2971 and CCG-2891 studies. Structural chromosomal abnormalities detected in ML-DS include trisomy 8, trisomy 11, and T21 (besides the constitutional T21), dup (1p), del (6q), del (7p), dup (7q), and del (16q). In contrast, the classic cytogenetic abnormalities seen in AML cases in children without DS, including AML-ETO t (8;21), PML-RARA t (15;17), CBFB-MYH11 inv (16), and MLL 11q23 rearrangements, are not seen in ML-DS. Furthermore, neonates and children with DS developing ML-DS usually do not acquire the specific non-DS childhood AMKL cytogenetic abnormality RBM15-MKL1 t (1;22) or the cryptic inversion of chromosome 16 that produces the CBFA2T3-GLIS2 fusion gene.
Molecular Abnormalities in Myeloid Leukemia of Down Syndrome
Whole genome/exome sequencing identified that the mean number of somatic mutations in TAM cases (with GATA1 mutations in all cases) was 1.5, whereas the number of mutations was significantly higher in ML-DS cases than in TAM. Acquired mutations were identified in genes encoding components of the cohesin complex ( RAD21 , STAG2 , NIPBL , SMC3 , SMC1A ); chromatin regulators ( EZH2 , SUZ12 , ASXL1 , DNMT3A ), cytokine signaling pathway regulators ( JAK1 , JAK3 , MPL , KRAS , NRAS , PTPN11 , SH2B3 ), and other genes that have also been previously shown to be been mutated in leukemia ( TP53 , ETV6 , SRSF2 ). These observations indicate that TAM is caused by a GATA1 mutation together with T21 and progresses to ML-DS because of the acquisition of additional mutations.
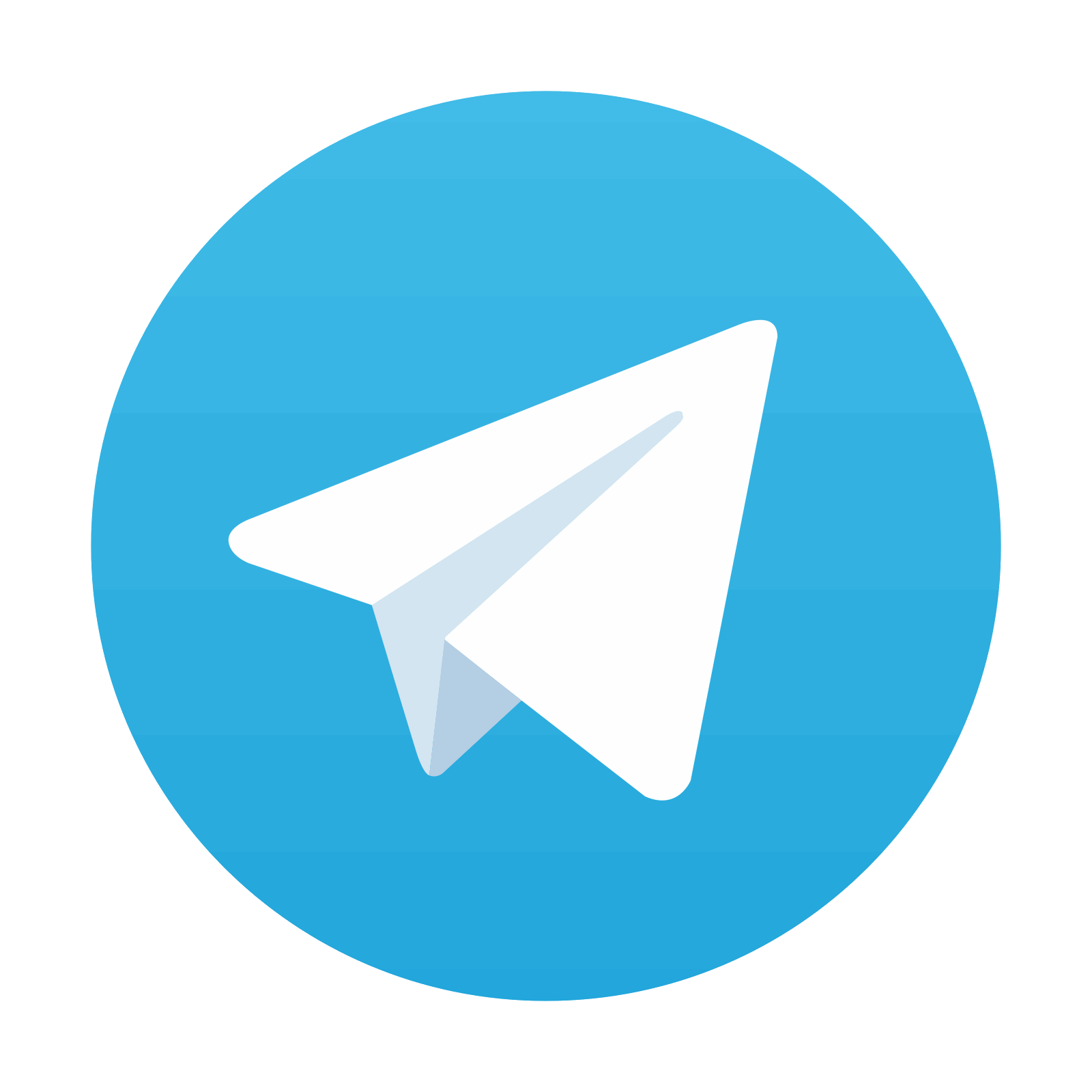
Stay updated, free articles. Join our Telegram channel

Full access? Get Clinical Tree
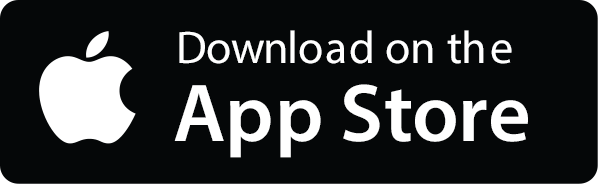
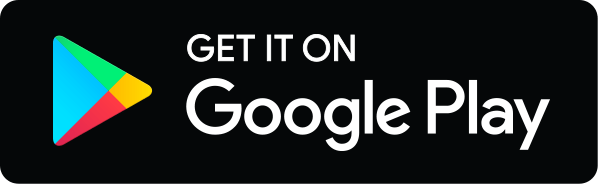