Diuretics
Jean-Pierre Guignard
Introduction
Diuretics promote the excretion of water and electrolytes. They are primarily used in states of inappropriate salt and water retention. Such states can be secondary to renal diseases (nephrotic syndrome, glomerulonephritis, chronic renal failure), congestive heart failure (CHF), and liver disease (cirrhosis). Diuretics are also used in a variety of clinical situations in which an increase in sodium excretion is not the primary goal of treatment. Such conditions include acute renal failure (ARF), electrolyte disturbances (hypo- or hyperkalemia, hypercalcemia, hypercalciuria) and nephrogenic diabetes insipidus. Effective therapeutic goals when using diuretics require thorough knowledge of the renal regulation of water and electrolytes.
Renal Function
Urine formation starts by the ultrafiltration of plasma through the glomerular capillary wall. The rate of glomerular filtration (GFR) is determined by the net filtration pressure across the glomerular capillaries and the glomerular ultrafiltration coefficient (Kf), which is the product of the surface area and the permeability of the glomerular capillaries:
GFR = Kf × net filtration pressure
Changes in systemic arterial pressure, in intrarenal arteriolar resistance, and in the plasma oncotic pressure modulate GFR (1). Both renal perfusion and GFR are controlled by hormones and autacoids such as angiotensin II, the prostaglandins, endothelin, bradykinin, and nitric oxide, and by the sympathetic nervous system. The filtration process does not produce significant changes in the concentration of small solutes. Modifications of the filtrate occur by the reabsorption and secretion of solutes across the renal tubular cells and by the reabsorption of water.
Transport of Solutes
Reabsorption of solutes is achieved by active or passive transport across the tubular cell membranes, using a transcellular or a paracellular route. Primary active transport requires a source of metabolic energy, provided by adenosine triphosphate (ATP) hydrolysis. The most important active process in the nephron is the Na+, K+-ATPase located on the basolateral side of the tubular cells. Other primary active transport mechanisms include various ATPases: Ca2+-ATPase, H+-ATPase, and a H+, K+-ATPase. Secondary active transport of solutes along (symport) or against (antiport) the Na+ gradient created by its primary active transport occurs via specific protein carrier molecules (transporters). Some proteins in the cell membranes, termed uniporters, transport only a single substance down the concentration gradient. Such is the case for glucose moving across the basolateral membrane of proximal tubular cells. Finally, cell membranes contain channels allowing the rapid passage of specific ions (Na+, K+, Cl–) across cellular membranes (2). Transport via channels or uniporters is sometimes referred to as facilitated diffusion.
Water flows passively down the osmotic gradient created by the active transport of solutes. The water reabsorbed along the nephron carries solutes, a process termed solvent drag. Macromolecules are transported by endocytosis, a process also using the energy provided by the ATPases.
Reabsorption of Sodium
The renal tubule can reabsorb up to 99% of the filtered load of sodium. Two-thirds are reabsorbed in the proximal tubule, 25% in the ascending limb of loop of Henle, and 10% in the distal tubule and collecting duct. The driving force for Na+ reabsorption is the Na+, K+-ATPase in the basolateral membrane of the tubular cell. The gradient created by the active pumping of Na+ out of the cell allows the passive entry of Na+ at the luminal membrane, along its electrochemical gradient. The basolateral pump also provides energy for the secondary antiport or symport transport of such solutes as Cl–, HCO3–, Ca2+, phosphate, glucose, urea, and amino acids (1).
In the early proximal tubule the reabsorption of Na+ is coupled to that of HCO3– and a number of organic molecules. Many of these solutes are almost completely removed from the tubular fluid in the first part of the
proximal tubule. In the second half of the proximal tubule, Na+ is reabsorbed with Cl–.
proximal tubule. In the second half of the proximal tubule, Na+ is reabsorbed with Cl–.
The thick ascending limb of loop of Henle reabsorbs approximately 25% of the filtered load of Na+. It is impermeable to water. The movement of Na+ across the luminal membrane is mediated by the Na+, 2 Cl–, K+ symporter. The Na+ that is reabsorbed is deposited in the medullary interstitium, where it is trapped by the countercurrent multiplier mechanism, together with urea. The integrity of the Na+, K+-ATPase and of the Na+, 2 Cl–, K+ cotransport is necessary for the generation of a hypertonic medulla required to concentrate the urine.
The early distal tubule forms part of the juxtaglomerular apparatus that provides the feedback control of single nephron GFR. In this part of the nephron, which is also impermeable to water, the continuous avid reabsorption of Na+ results in the dilution of urine. This segment is thus referred to as the diluting segment.
The late distal tubule and collecting duct are composed of two distinct cell types, the principal cells and the intercalated cells. The principal cells reabsorb Na+ and water and secrete K+, a process controlled by aldosterone. The intercalated cells reabsorb K+ and secrete H+ in the tubular lumen. These cells play an important role in regulating acid–base balance. In the distal tubule and collecting duct, part of sodium enters the luminal membrane via an epithelial Na+ channel (ENA C).
The medullary collecting duct reabsorbs ∼3% of the filtered load of Na+. Active H+ secretion against steep concentration gradients occurs in this segment, which is essential for the excretion of fixed acids. The permeability of the collecting duct cells to water is under the influence of arginine vasopressin (AVP), also known as the antidiuretic hormone (ADH) (1).
Transport of Water
The proximal tubule is highly permeable to water, much of which passes through the cells via aquaporin-1 water channels present in both the apical and the basolateral cell membranes (3). While the descending limb of loop of the Henle is highly permeable to water, the thin and thick ascending segments of the loop are almost totally impermeable. The permeability of the collecting duct to water is under the control of AVP. This hormone increases the permeability of the cortical tubular cells by incorporating aquaporin-2 water channels in the apical membrane (3). In the presence of AVP, the permeability of this segment to water is thus increased, allowing the diffusion of free water out of the tubular lumen into the highly hypertonic interstitium (1).
Transport of Other Cations and Anions (K+, CA2+, Pi)
Potassium
The kidneys play a major role in maintaining K+ balance. Two-thirds of the filtered K+ are reabsorbed in the proximal tubule and 20% in the ascending limb of loop of Henle. The regulation of K+ excretion occurs via the secretion of K+ in the distal tubule and collecting duct. When the dietary intake of K+ is too large, K+ excretion can exceed the K+ filtered load. Three major factors control the rate of distal K+ secretion: (a) the activity of the Na+, K+-ATPase; (b) the electrochemical gradient for K+ across the apical membrane; and (c) the permeability of the apical membrane to K+. The excretion of K+ is regulated by the plasma K+ concentration, the acid–base balance, aldosterone, and AVP.
Calcium
Seventy percent of the filtered Ca2+ is reabsorbed in the proximal tubule, 20% in the thick ascending limb of loop of Henle, 9% in the distal tubule, and 1% in the collecting duct. In the proximal tubule, Ca2+ enters the apical membrane by diffusing through a paracellular route or Ca2+ channels, down the electrochemical gradient created by the primary and secondary active transport of Ca2+ out of the basolateral membrane (Ca2+-ATPase; Na+-Ca2+ antiport). Reabsorption of Ca2+ in the ascending limb is largely passive down the electrochemical gradient created by the active reabsorption of Na+. The reabsorption of Na+ and Ca2+ changes in parallel in both the proximal and the thick ascending limb of loop of Henle. This accounts for the occurrence of parallel changes in Ca2+ and Na+ excretion. In the distal tubule the reabsorption of Ca2+ is entirely active and independent from that of Na+. The renal excretion of Ca2+ is regulated by parathyroid hormone, calcitriol, and calcitonin (1).
Phosphate
The proximal tubule reabsorbs 80% of the filtered load of Pi and the distal tubule 10%. The remaining 10% is excreted in the urine. Pi enters the apical membrane by a 2Na+–Pi symporter and exits the cell across the basolateral membrane by a Pi–anion antiporter. The excretion of Pi is mainly regulated by parathyroid hormone. Extracellular fluid (ECF) volume expansion decreases the reabsorption of Pi. Other factors depressing the reabsorption of Pi include metabolic acidosis and the glucocorticoids (1).
Secretion of Organic Cations and Anions
The proximal tubule reabsorbs solutes and water and also secretes various organic cations and anions, many of which are end products of metabolism. The transport mechanisms for both organic cations and anions are nonspecific, so that several cations and several anions can compete for the cationic or the anionic secretory pathways. In addition to endogenous organic cations and anions, various exogenous drugs are also eliminated by secretion. Such is the case for the anionic diuretics (acetazolamide, thiazides, furosemide, bumetanide) or cationic diuretics (amiloride) (1).
Regulation of Na+ and Water Transport
In addition to the glomerular tubular balance that via Starling forces adapts the reabsorption of Na+ to its filtered load, Na+ transport is regulated by various hormones and paracrine factors. Angiotensin II, aldosterone, the atrial natriuretic peptide (ANP), the prostaglandins, the catecholamines, and dopamine play major roles (1).
Angiotensin II
Angiotensin II stimulates proximal Na+ and water reabsorption by increasing the apical Na+/H+ exchange. It is an important secretagogue for aldosterone. By acting on the zona glomerulosa of the adrenal cortex to release aldosterone, it indirectly favors distal Na+ reabsorption. Angiotensin II release is activated by a decrease in extracellular fluid volume and renal perfusion.
Aldosterone
Aldosterone stimulates the distal reabsorption of Na+ by increasing the permeability of the collecting tubule luminal membrane to Na+. Aldosterone also favors the secretion of K+ and H+ and increases the reabsorption of Cl–. Aldosterone stimulates the Na+, K+-ATPase and the production of ATP. The secretion of aldosterone by the adrenal cortex is stimulated by angiotensin II, hyperkalemia, and severe hyponatremia.
Atrial Natriuretic Peptide
The heart produces two natriuretic peptides: the ANP stored in the atrial myocytes and the brain natriuretic peptide (BNP) stored in the ventricular myocytes. Both peptides are released when the heart dilates. The kidneys produce a related natriuretic peptide termed urodilatin. In general, the natriuretic peptides antagonize the actions of the renin–angiotensin–aldosterone system. The 28-amino acids peptide is released when atrial stretch receptors sense an increase in the effective circulating volume. ANP stimulates the excretion of Na+ by (a) increasing GFR via vasodilatation of the afferent arterioles; (b) inhibiting renin release and the secretion of aldosterone; (c) inhibiting the Na+, K+-ATPase in the inner medullary collecting duct, and (d) closing the epithelial Na+ channels.
Prostaglandins
The prostaglandins, synthesized by the cyclooxygenases in the renal cortex, the medullary interstitium, and the collecting duct epithelial cells, increase Na+ excretion. They do it by increasing GFR and by inhibiting Na+ reabsorption in the collecting duct.
Norepinephrine and Epinephrine
Norepinephrine and epinephrine released from sympathetic nerves and the adrenal medulla, respectively, stimulate Na+ reabsorption in the proximal tubule, the thick ascending limb of the loop of Henle, the distal tubule, and the collecting duct.
Dopamine
Dopamine, synthesized by proximal tubular cells and dopaminergic nerves, increases the excretion of Na+ by inhibiting Na+ proximal reabsorption.
Antidiuretic Hormone (Arginine Vasopressin)
Water following Na+ passively, its reabsorption is increased by all the factors that stimulate Na+ reabsorption. The hormone that really regulates water balance is AVP, a nonapeptide with a molecular weight of 1,099 daltons.
Three types of receptors mediate the actions of AVP. V1A receptors are located on vascular smooth muscle and mediate vasoconstriction. V1B receptors are present in the anterior pituitary gland and mediate the release of adrenocorticotropic hormone. The V2 receptors are located in the renal distal tubule and collecting duct. AVP binding to the V2 receptors leads to activation of an adenylyl cyclase with subsequent rise in intracellular cyclic AMP. This second messenger mediates the translocation of intracellular vesicles containing the water channel aquaporin-2 into the apical plasma membrane (3) and increases the transcription of aquaporin 2 (AQP-2). AVP-regulated AQP-2 shuttling is the primary determinant for water permeability of the collecting duct and, consequently, for the antidiuresis. In addition, AVP stimulates Na+–K+–2Cl– cotransport in the ascending limb of loop of Henle via V2 receptors.
The release of AVP is rapid, its plasma half-life is short (10 to 15 minutes), thus allowing a fine regulation of the plasma osmolality. Small changes in Posm (±3 mOsm per kg H2 O) activate or inhibit AVP release. Osmoreceptors, located in the supraoptic nucleus of the hypothalamus, regulate the release of AVP. Volo- and baroreceptors also affect AVP release. Because of the greater sensitivity of the osmoreceptors, their influence predominates when changes in osmolality and plasma volume are moderate. When large changes in plasma volume occur, however, the influence of the volo- and baroreceptors predominates over that of the osmoreceptors, leading to “inappropriate” secretion of AVP, with consequent water retention and hemodilution. Nonosmotic stimulation or inhibition of AVP release occurs as a consequence of drugs (nicotine, morphine, barbiturates), pain, and respiratory or cerebral distress (1).
Body Fluid Homeostasis
The kidney is responsible for maintaining the ECF fluid volume and osmolality constant in spite of large changes in salt and water intake.
Body Fluid Volume
NaCl, the major osmotically active solute in ECF, determines its volume. The balance between the intake and the
renal excretion of Na+ thus regulates ECF volume, and as a consequence cardiac output and blood pressure. Long-term changes in Na+ excretion are regulated by aldosterone. More rapid changes in Na+ excretion are achieved by changes in GFR and/or by various intrarenal hormones that regulate Na+ reabsorption.
renal excretion of Na+ thus regulates ECF volume, and as a consequence cardiac output and blood pressure. Long-term changes in Na+ excretion are regulated by aldosterone. More rapid changes in Na+ excretion are achieved by changes in GFR and/or by various intrarenal hormones that regulate Na+ reabsorption.
ECF volume is closely related to plasma volume. Alterations in plasma volume are sensed both on the venous and on the arterial side of the circulation. Arterial sensors perceive the adequacy of blood flow in the arterial circuit, a parameter coined as effective circulating volume. This volume is also sensed by baroreceptors located in the juxtaglomerular apparatus of the kidney. A decrease in renal perfusion pressure activates the renin–angiotensin–aldosterone system. Changes in the effective circulating volume activate other intrarenal hormones that modulate Na+ reabsorption either directly by an action on tubular transport or indirectly by changes in GFR (1).
Body Fluid Osmolality
The plasma osmolality is maintained within narrow limits. A 2% to 3% increase in Posm stimulates the osmoreceptors with the consequent release of AVP, leading to the reabsorption of free water in the collecting duct. Urine flow rate decreases and the osmolality of the urine increases. The opposite happens when Posm decreases by 2% to 3%. The concentration of urine can vary from 40 to 1,400 mOsm per kg H2 O, depending on whether AVP is completely absent or maximally stimulated. Concentration of the urine requires the presence of a hypertonic medullary interstitium. Impaired NaCl transport in the thick ascending limb of loop of Henle or defective countercurrent multiplier mechanisms results in a decrease in maximal urine osmolality (1).
Developmental Aspects of Renal Function and Body Fluid Homeostasis
In the human fetus, urine formation starts around the 10th to 12th week of gestation. The urine is normally hypotonic, indicating that the kidney actively reabsorbs electrolytes and solutes from the glomerular ultrafiltrate. The fetal kidney responds to diuretics administered to the mother by increasing its urine flow rate. The full complement of nephrons is achieved around the 35th week of gestation. GFR and renal plasma flow increase progressively throughout gestation but remain relatively low. GFR at birth is equal to 20 mL per minute per 1.73 m2 in term infants and doubles in the first 2 weeks of life. It reaches mature levels of 100 mL per minute per 1.73 m2 by the 6th to 12th month of postnatal age. GFR is lower at birth in premature neonates and develops at a somewhat lower velocity (4,5).
The reabsorption of filtered solute also undergoes maturational changes. Proximal Na+ reabsorption increases in parallel with an increase in the activity of the Na+, K+-ATPase and in the number of the glucose- and bicarbonate-Na+ cotransporters. Reabsorption in the loop of Henle also increases along with the activity of the Na+, K+-ATPase in this segment. Resistance of the distal tubule to aldosterone has been claimed to be partly responsible for Na+ wasting in the first weeks of premature infants (6).
In early postnatal life, several transporters and enzymes involved in the reabsorption of bicarbonate are weakly expressed, resulting in a relatively low HCO3– excretion threshold. The overall reabsorption of various electrolytes and solutes also undergoes maturational changes linked to the maturation of the transporters, symporters, and antiporters.
The distribution of water in the various compartments of the body varies with age. The ECF volume decreases from 60% body weight at 28 weeks of gestation to 42% at term and 20% at 3 months of age. These changes reflect the excretion of excessive ECF and a gradual increase in the amount of fat tissues. Although the volume of body fluid varies during growth, the tonicity of ECF is kept constant by the kidney, which excretes or retains appropriate amounts of water (7).
The newborn, premature or at term, is able to decrease urine osmolality to values as low as 40 mOsm per kg H2 O. Free-water excretion depends on the distal reabsorption of Na+ and hence on the amount of Na+ delivered to the distal tubule. Because of the low GFR present in the newborn infant, the ability to excrete large amounts of free water is limited (7).
The concentrating ability is limited in newborn infants, the more so in the preterm neonates. The relative ineffectiveness of the concentrating mechanism in the neonate has been ascribed to (a) a low corticomedullary concentration gradient; (b) shortness of the loops of Henle; (c) decreased formation of cAMP in response to ADH; and (d) interference of the elevated levels of prostaglandins with the vasopressin-stimulated cAMP synthesis.
In spite of elevated aldosterone levels, premature neonates frequently have difficulties in maintaining a positive Na+ balance on a Na+ intake of 1 to 2 mmol per kg per day on the 2nd to 4th week of life. Deficient proximal NaCl reabsorption and relative resistance to aldosterone have been claimed to be responsible for Na+ wasting. Both the renin–angiotensin system and the osmo-voloreceptors control system of ADH are efficient in the early newborn period. Hyponatremia is frequently seen, however, as the consequence of inappropriate secretion of ADH secondary to respiratory or cerebral distress (7,8).
Clinical Use of Diuretics
Diuretics are used in sodium-retaining states with or without the formation of edema, in situations of arterial hypertension or electrolyte imbalances, in oliguric renal failure, and in nephrogenic diabetes insipidus (9,10,11,12). Diuretics can be used to test the integrity of distal tubular function.
Edematous States
Salt and water retention with edema formation can occur as a primary event or as a consequence of reduced effective circulating volume with secondary hyperaldosteronism. The use of diuretics can be lifesaving when Na retention is associated with an expansion of the ECF volume. It may on the contrary further compromise the situation when sodium
retention occurs in response to homeostatic mechanisms mobilized to defend the circulating volume. The use of diuretics therefore requires careful monitoring of the patient’s hemodynamic states and an understanding of his or her underlying condition (6,13).
retention occurs in response to homeostatic mechanisms mobilized to defend the circulating volume. The use of diuretics therefore requires careful monitoring of the patient’s hemodynamic states and an understanding of his or her underlying condition (6,13).
Congestive Heart Failure
CHF is associated with an increase in pressure in the venous circulation. The increased capillary pressure favors the movement of fluid into the interstitium, resulting in the formation of edema. Failure of the heart to provide normal tissue perfusion is sensed as a decrease in effective circulating volume by the kidney, which is stimulated to retain NaCl and water. The presence of edema thus represents a side effect of the compensatory renal response to defend the effective circulating volume. The treatment of this condition consists in restoring normal cardiac output. By mobilizing the edematous fluid, diuretics improve the symptoms of CHF. The pulmonary edema secondary to left-sided heart failure requires the urgent use of diuretics to reduce the life-threatening pulmonary congestion (11).
Nephrotic Syndrome
The nephrotic syndrome is characterized by an increase in the permeability of the glomerular barrier to proteins, heavy proteinuria, hypoproteinemia, and the formation of edema. Two pathogenic mechanisms have been proposed to explain the formation of edema. In the underfill hypothesis, decreased plasma oncotic pressure results in decreased effective circulating volume with consequent secondary hyperaldosteronism and hypervasopressinism, leading to progressive salt and water retention. In this situation, usually seen at the onset of the minimal changes in nephrotic syndrome, the hypovolemia is manifested by clinical signs such as tachycardia, abdominal pain, and poorly perfused limbs. Elevated hematocrit and very low urinary sodium (<10 mmol per L) confirm the state of hypovolemia. Diuretics should only be used with great caution in this condition and only after correction of the hypovolemia by albumin infusion.
In the overfill theory (14), the edema is caused by the primary retention of Na+ and water, with increased plasma volume, increased hydrostatic capillary pressure, and leak of fluid into the interstitium. The pathogenesis of saline retention in the overfill hypothesis is not yet clear. Decrease in the activity of the ANP has been claimed to be responsible for the increased Na+ retention. These patients benefit from diuretic administration.
Liver Diseases
The occurrence of edema in patients with liver disease is secondary to decreased albumin synthesis, decreased oncotic pressure, and decreased effective circulating volume. The kidney responds by increasing salt and water retention. As ascitis develops, there is a rise in intra-abdominal pressure, a phenomenon that impairs venous drainage from the lower limbs. Although diuretic therapy for ascitis fails to improve survival in children, it benefits quality of life and limits complications such as bacterial peritonitis (16). Defective liver function also results in impaired inactivation of salt- and water-retaining hormones, such as aldosterone or vasopressin.
Arterial Hypertension
Arterial hypertension may be secondary to salt retention and expansion of the ECF volume. Na+ retention may be directly related to excessive synthesis of aldosterone in primary hyperaldosteronism. In the renal vascular form of hypertension, increased formation of angiotensin II elevates the blood pressure by inducing vasoconstriction and by stimulating sodium reabsorption. In essential hypertension, the retention of Na+ is not obvious. It has been recently hypothesized that impaired extrusion of Na+ out of the cells, secondary to defective Na+/Ca2+ counter transport could lead to an increase in intracellular Ca2+ with consequent increased vascular contractility (17). By leading to ECF volume contraction, diuretics decrease the blood pressure. They are often used as first-line drugs in the treatment of arterial hypertension (18,19). In adults, low-dose diuretics (hydrochlorothiazide 12.5 mg; torasemide 2.5 mg) constitute effective one-daily monopharmacotherapies for mild-to-moderate uncomplicated essential hypertension (17).
Electrolytes Imbalance
Diuretics can be used in various situations associated with dyselectrolytemia. They can increase K+ excretion in hyperkalemic states (loop diuretics, thiazides), increase calcium excretion in hypercalcemia (loop diuretics), or decrease the rate of calcium excretion in hypercalciuric states (thiazides). Increased HCO3– excretion can be achieved by the acetazolamide, whereas increased H+ excretion can be stimulated by loop diuretics.
Nephrogenic Diabetes Insipidus
This condition is characterized by the resistance of the collecting duct to the action of ADH. It can be due either to a X-linked congenital defect in the V2 receptors or to an autosomal recessive mutation in the aquaporin-2 molecule. The patient with diabetes insipidus excretes large amounts of dilute urine. The thiazides can reduce the extracellular space volume by impairing sodium distal reabsorption and increasing NaCl excretion. This decrease in ECF volume stimulates proximal salt and water retention, thus eventually leading to reduced urine flow rate. Prolonged treatment with hydrochlorothiazide–amiloride appears to be more effective and better tolerated than with just hydrochlorothiazide. Such treatment is as efficacious as the association hydrochlorothiazide–indomethacin, with fewer severe side effects (20).
Acute Renal Failure
Because they have been shown to variably increase total renal blood flow, loop diuretics are often administered to patients with oliguric renal insufficiency, in the hope of
promoting diuresis and improving GFR and renal perfusion (31). Although diuretic administration may convert oliguric ARF to nonoliguric ARF, there is no evidence that this treatment can ameliorate renal function or improve the outcome of patients with ARF (21).
promoting diuresis and improving GFR and renal perfusion (31). Although diuretic administration may convert oliguric ARF to nonoliguric ARF, there is no evidence that this treatment can ameliorate renal function or improve the outcome of patients with ARF (21).
Differential Diagnosis of Congenital Tubulopathies
Diuretics such as acetazolamide, furosemide, or hydro-chlorothiazide can be used to test distal tubular acidification or distal sodium reabsorption defects.
Classification of Diuretics
Diuretics can be classified according to their site and mode of action (Fig. 43.1, Table 43.1). They all increase sodium and water excretion and variably modify electrolyte excretion (Table 43.2). Filtration diuretics increase salt and water excretion by primarily increasing GFR. Osmotic diuretics depress salt and electrolyte reabsorption in the proximal tubule and in loop of Henle. Inhibitors of carbonic anhydrase act primarily on the proximal tubule. The most commonly used diuretics inhibit Na+ reabsorption either in the ascending limb of loop of Henle (loop diuretics), in the distal convoluted tubule (thiazide and thiazide-like diuretics), or in the late distal tubule and collecting duct (K+-sparing diuretics) (12,10). New diuretics are being developed with different modes of action (natriuretic peptides, adenosine antagonists, vasopressin antagonists). All diuretics share adverse effects, which are actually extension of their primary effects on electrolyte excretion (Table 43.3), as well as nonelectrolyte adverse effects (Table 43.4).
Filtration Diuretics
Agents that increase diuresis by increasing GFR are sometimes called filtration diuretics. These agents include the glucocorticoids and theophylline, as well as inotropic agents such as isoproterenol, dopamine and dobutamine. By increasing GFR, these drugs only moderately increase Na+ excretion. Part of the natriuresis achieved by filtration diuretics reflects inhibition of tubular Na+ reabsorption rather than an increase in the Na+ filtered load only.
Table 43.1 Clinical Use of Diuretics | ||||||||||||||||||||||||||||||||
---|---|---|---|---|---|---|---|---|---|---|---|---|---|---|---|---|---|---|---|---|---|---|---|---|---|---|---|---|---|---|---|---|
|
Table 43.2 Acute Effects of Diuretics on Electrolyte Excretiona | |||||||||||||||||||||||||||||||||||||||||||||||||||||||||||||||||||||||||||||||||||||||||||||||||||
---|---|---|---|---|---|---|---|---|---|---|---|---|---|---|---|---|---|---|---|---|---|---|---|---|---|---|---|---|---|---|---|---|---|---|---|---|---|---|---|---|---|---|---|---|---|---|---|---|---|---|---|---|---|---|---|---|---|---|---|---|---|---|---|---|---|---|---|---|---|---|---|---|---|---|---|---|---|---|---|---|---|---|---|---|---|---|---|---|---|---|---|---|---|---|---|---|---|---|---|
|
Dopaminergic Agents
Dopamine, a naturally occurring catecholamine, acts on the specific dopaminergic receptors DA1 and DA2, and also activates the α- and the β-adrenoreceptors. Dopamine may improve renal function by (a) increasing cardiac output through stimulation of the β-adrenergic receptors, (b) increasing blood pressure by inotropic and vasoactive mechanisms, (c) increasing renal perfusion via stimulation of the dopamine receptors in the renal vessels, and (d) directly inhibiting sodium reabsorption (22). The beneficial effects of low-dose dopamine in oliguric states are variable and, when present, modest. Because of a great overlap in the response to dopamine and significant individual variation, no dose is clearly only a beneficial renal dose (23). Even at low doses, dopamine may increase cardiac contractility and systemic vascular resistance and produce tachycardia, arrhythmia, tissue necrosis, and digital gangrene (24). Dopamine also blunts hypoxic ventilatory drive and may increase pulmonary shunt fraction in critically ill patients. Until efficacy and safety are demonstrated in control prospective studies, the use of dopamine as a renal protective agent remains questionable (25). The price of the modest improvement in urine output and sodium excretion with dopamine may be too high.
Table 43.3 Electrolytes Disturbances Induced by Diuretics | ||||||||||||||||||||||||||||||||||||||||||||||||||||||||||||||||||||||||||||||
---|---|---|---|---|---|---|---|---|---|---|---|---|---|---|---|---|---|---|---|---|---|---|---|---|---|---|---|---|---|---|---|---|---|---|---|---|---|---|---|---|---|---|---|---|---|---|---|---|---|---|---|---|---|---|---|---|---|---|---|---|---|---|---|---|---|---|---|---|---|---|---|---|---|---|---|---|---|---|
|
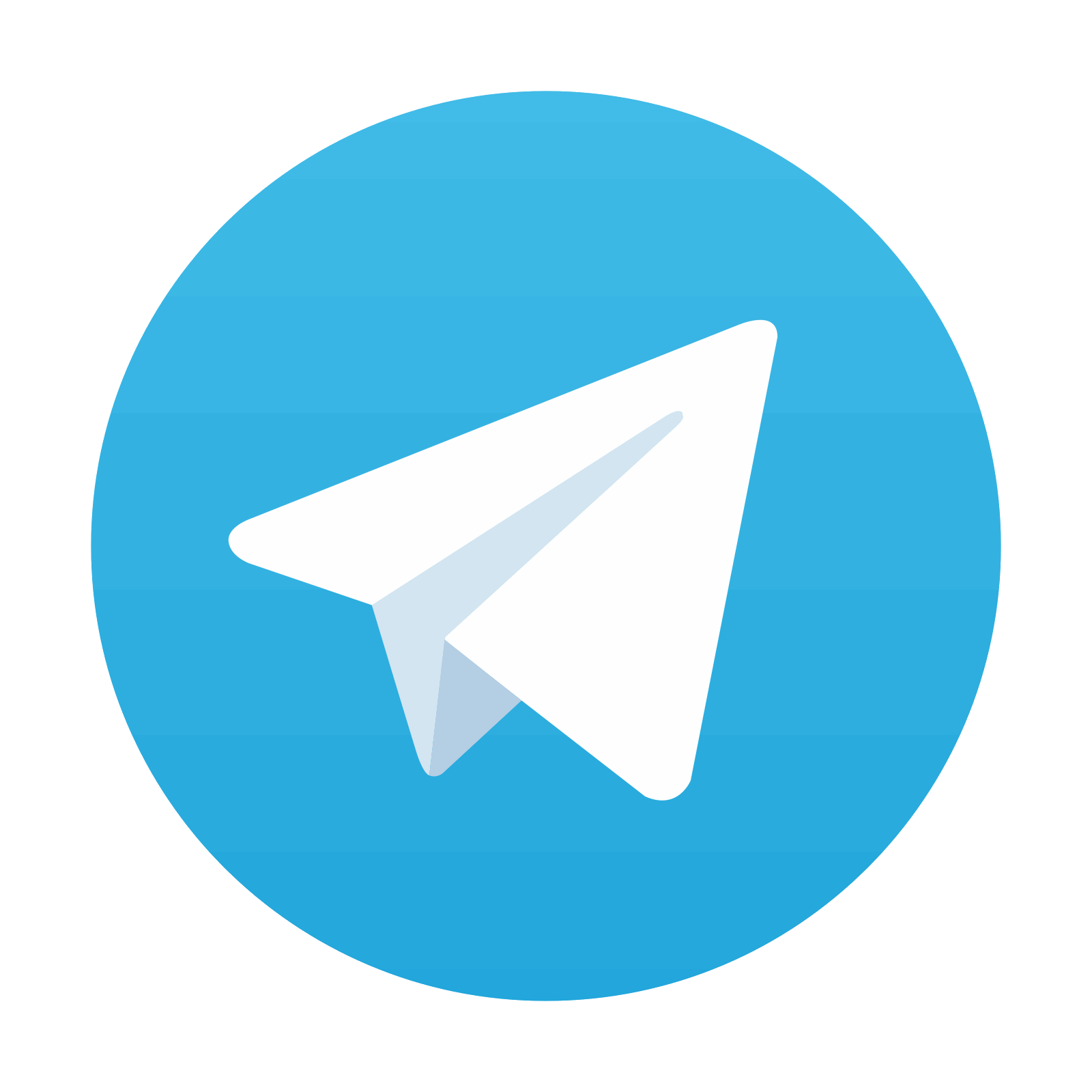
Stay updated, free articles. Join our Telegram channel

Full access? Get Clinical Tree
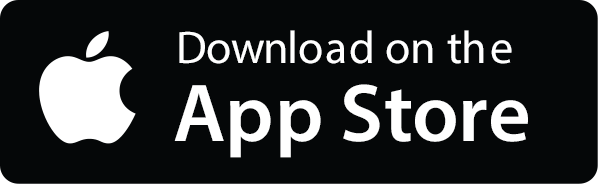
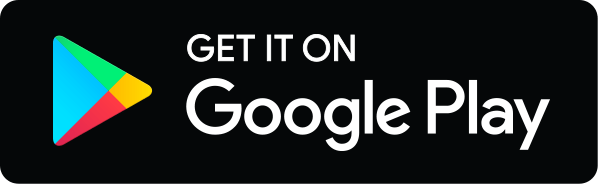