Objective
Although premature cervical remodeling is involved in preterm birth (PTB), the molecular pathways that are involved have not been elucidated fully. MicroRNAs (miRNAs) that are highly conserved single-stranded noncoding RNAs that play a crucial role in gene regulation have now been identified as important players in disease states. The objective of this study was to determine whether miRNA profiles in cervical cells are different in women who are destined to have a PTB compared with a term birth.
Study Design
A nested case-control study was performed. With the use of a noninvasive method, cervical cells were obtained at 2 time points in pregnancy. The cervical cell miRNA expression profiles were compared between women who ultimately had a PTB (n = 10) compared with a term birth (n = 10). MiRNA expression profiles were created with the Affymetrix GeneChip miRNA Array. The data were analyzed with the Significance of Analysis of Microarrays and Principle Components Analyses. A false-discovery rate of 20% was used to determine the most differentially expressed miRNAs. Validation was performed with quantitative polymerase chain reaction. In vitro studies were performed to confirm expression and regulation of select miRNAs.
Results
With a false-discovery rate of 20% of the 5640 miRNAs that were analyzed on the array, 99 miRNAs differed between those with a PTB vs a term birth. Qualitative polymerase chain reaction validated the array findings. In vitro studies confirmed expression of select miRNAs in cervical cells.
Conclusion
MiRNA profiles in cervical cells may distinguish women who are at risk for PTB months before the outcome. With the large downstream effects of miRNAs on gene expression, these studies provide a new understanding of the processes that are involved in premature cervical remodeling and allow for the discovery of new therapeutic targets.
For Editors’ Commentary, see Contents
Preterm birth remains the leading cause of childhood morbidity and death. Although there have been some clinical trials that have demonstrated a reduction in the preterm birth rate by targeting women who are at high risk, preventative or interventional strategies that significantly reduce the national and international preterm birth rate have not yet been realized. The major impediment to more impactful interventional strategies has been our lack of understanding of the essential mechanisms that are involved in the pathogenesis of preterm birth.
Clinical data demonstrate that a short cervix is one of the most consistent predictors of preterm birth. However, what the molecular equivalent to a sonographic short cervix is remains unknown. Indeed, although a sonographic short cervix is believed to be a surrogate for “premature cervical remodeling,” there is a lack of evidence revealing what actually is occurring (ie, molecularly, biochemically) in cervical tissue at the time when a sonographic short cervix becomes evident. This information is critical if we are to discover more effective prevention strategies for preterm birth. It is known that remodeling of the cervix must occur at any gestational age to allow the passage of the fetus. Hence, cervical remodeling, at term or preterm, is an obligatory molecular event in the process of parturition. Animal studies have revealed some key insights regarding the pathways that are involved in the process of cervical remodeling. However, whether these revealed processes in animal models correlate to what occurs in human pregnancy is assumed but not proved. Human studies are very limited in regards to assessing the molecular events in the pregnant cervix. Two studies have been performed that involved the biopsy of the cervix in women at term. These studies demonstrated how gene expression patterns differ in the cervix in laboring vs nonlaboring women as well as between those women with and without a ripe cervix. Although these studies provide some insight to the process of cervical ripening at term, they involve a more invasive method to assess the molecular phenotype of the pregnant cervix. Notably, there are no studies to date that provide information regarding the molecular events that occur in the cervix that might be associated with premature cervical remodeling and preterm parturition.
Although animal studies and 2 human studies suggest some changes in gene expression in the cervix in preparation of parturition, most of these studies have been limited to investigations of messenger RNA (mRNA) expression. Although these studies provide molecular insight, recent emergence of the role of microRNAs (miRNAs) in gene regulation support the concept that investigation of miRNAs in normal and pathologic processes of cervical remodeling might provide greater information.
In the last decade, miRNA biology has emerged as an important player in both physiologic and pathophysiologic responses. MiRNAs are small (approximately 22 nucleotides in length) highly conserved single-stranded RNA molecules that play a critical role in posttranscriptional gene regulation by interacting with the 3′ untranslated regions of specific mRNA targets to affect mRNA stability and translation negatively. In most organisms, there are a limited number of miRNAs (estimated at approximately 1000 in human genome) compared with the number of mRNAs (approximately 30,000). However, 1 miRNA may regulate hundreds of mRNAs and consequently have significant and wide-ranging effects on gene expression networks. A primary role of miRNAs appears to be specific regulation or “fine-tuning” of gene expression to control development and tissue homeostasis. However, under certain conditions (eg, stress, inflammation, hypoxia), the functions of miRNAs become pronounced, which suggests an essential role of miRNAs in disease states. MiRNAs are now implicated in varied disease states that include cancer and cardiovascular disease and are considered as important therapeutic targets. Despite the growing importance of miRNAs to pathogenic states, there is no existing study that has explored the role of miRNAs in cervical remodeling and their possible involvement in preterm birth.
Our objective for this study was to determine whether there was a distinct miRNA profile in cervical cells that were collected from women who were destined to have a preterm birth compared with a term birth. For this study, we performed a nested case-control study within a prospective cohort of women who were at high risk for preterm birth. MiRNA array analysis was performed and followed by validation by quantitative polymerase chain reaction (QPCR). Exploration of the regulation of discovered miRNAs by inflammation was performed with in vitro model systems.
Methods
Nested case-control within a prospective cohort
This study used biospecimens that were collected as part of a prospective cohort study that was called “PREDICT.” This study was approved by the Institutional Review Board at the University of Pennsylvania and was performed at a single, urban tertiary care center between August 2011 and November 2012. The cohort consisted of women who were at high risk for preterm birth because of history of previous spontaneous preterm birth or second-trimester loss, previous cervical surgery without a subsequent full-term birth, and/or uterine anomaly with a singleton pregnancy. Women who used systemic steroids or immunosuppressive therapy, who had HIV, lupus, pregestational diabetes mellitus, rheumatoid arthritis, Crohn’s disease, ulcerative colitis, or who had cancer or an organ transplant were excluded. Additionally, women who had anything in the vagina within the last 24 hours (including sexual intercourse, vaginal probe, digital cervical examination, lubricants), had active vaginal bleeding, had evidence of rupture of membranes, and/or had cervical dilation ≥3 cm at the time of enrollment were excluded. In this study, clinical and biospecimen data were collected at 2 time points: (1) visit 1 occurred at 20 weeks to 23 weeks 6 day gestation (V1) and (2) visit 2 occurred at 24 weeks to 27 weeks 6 days gestation (V2). For the study presented here, 10 women with a spontaneous preterm birth at <37 weeks of gestation and 10 women with a term delivery (>37 weeks of gestation) were identified. No matching by race or parity was performed. All cases of preterm birth were reviewed by 1 of the investigators (J.B.) to confirm that the preterm delivery was a spontaneous delivery.
Biospecimen collection
To ascertain what might be occurring molecularly in the cervix during pregnancy, we created a “RNA PAP” technique. This technique involves the collection of cervical cells in a method similar to the performance of a Papanicolaou (PAP) smear. A cytobrush is used to collect cells from the ectocervix. The collected cells are placed in QIAzol lysis reagent (Qiagen, Valencia, CA), and the specimen is immediately placed in liquid nitrogen. The frozen sample is stored at –80°C until future use. The method for RNA extraction from these samples is noted later. We have confirmed that using this technique, in any trimester of pregnancy, provides adequate RNA to perform expression analyses (unpublished data). Based on existing data from studies that have assessed cell populations from PAP smears, we assumed that the RNA PAP contains mostly epithelial cells (ectocervical and endocervical cells) and some leukocytes. Our ability to harvest adequate RNA for gene expression studies is consistent with what has been reported with the use of nonpregnant PAP specimens. For this study, an RNA PAP was performed at each study visit.
Clinical data collection
Transvaginal cervical length (CL) measurement was performed on V1 and 2. CL measurement was performed per the protocol established by Iams et al ; the shortest measurement was used for each patient. Clinical data were collected from the patient’s medical records and verified with the patient; delivery information was obtained at the conclusion of the pregnancy.
Statistical analyses for clinical data
χ 2 analyses were performed to determine associations between categoric demographic data. CL values at V2 and selected targets (miR-143, miR-145, and JAM-A) were distributed normally. Therefore, parametric tests were performed to determine the association between median miRNA levels and dichotomous CL. Nonparametric correlation coefficients were calculated between select miRNAs and continuous CL.
In vitro modeling: cell culture and cell treatments
Immortalized human papillomavirus 16/E6E7 ectocervical (American Type Culture Collection CRL-2614) cells (American Type Culture Collection, Manassas, VA) were maintained in keratinocyte serum-free medium (GIBCO BRL Life Technologies, Gaithersburg, MD) supplemented with 50 μg/mL bovine pituitary extract, 0.1 ng/mL epidermal growth factor, 100 U/mL penicillin, and 100 μg/mL of streptomycin at 37°C in a 5% CO 2 humidified incubator. Ectocervical cells were plated at 1.5 × 10 5 cells/well in 6-well plates for 24 hours in normal growth media as previously described. The cells were then grown in keratinocyte media without growth factors to mimic “serum starved” growth conditions for 24 hours before treatment with 10 μg/mL lipopolysaccharide strain Escherichia coli 055:B5 (Sigma, St Louis, MO; n = 6 per treatment group). At 6 and 24 hours of treatment, the media were removed, and the cells were washed with sterile phosphate-buffered saline solution. The cells were collected in Trizol (Life Technologies, Carlsbad, CA) for total RNA extraction.
Total RNA isolation
Total RNA that included miRNAs and other small RNAs were extracted from the human biospecimens (RNA PAP) and in vitro studies in the same manner. RNA was extracted by phenol/chloroform extraction followed by column-based purification, as described in the manufacturer’s protocol for Qiagen’s miRNeasy Mini kit. The RNA was eluted in 30 μL of RNase-free water. RNA concentration was determined with a spectrophotometer (NanoDrop 2000 Spectrophotometer; Nanodrop, Rockland, DE), and RNA integrity was verified with a bioanalyzer (Agilent 2100 Bioanalyzer; Agilent Technologies, Palo Alto CA). The isolated RNA from cervical cells was used for miRNA profiling and array validation. RNA from ectocervical and endocervical cells was used for miRNA measurement by QPCR.
MiRNA array methods and analyses
MiRNA profiling was performed with the RNA PAP specimen (V2 specimen) from women who delivered at term (n = 10) and preterm (n = 10). Total RNA (600 ng) was 3′ labeled with the FlashTag Biotin HSR RNA Labeling Kit (Affymetrix, Santa Clara, CA), according to the manufacturer’s protocol. Samples were then hybridized onto Genechip miRNA 3.0 arrays for 18 hours (Affymetrix). Hybridization images were then scanned and digitized with the Genechip Scanner 3000 (Affymetrix). The normalized signal intensity was log 2 transformed, and data analysis was performed with The Partek 6.6 Genomic Suite software (Copywrite; Partek Inc, St. Louis, MO). Statistical Analysis of Microarrays ( http://www-stat.stanford.edu/∼tibs/SAM/ ) was used to analyze the data and generate false-discovery rates for individual miRNAs. After we used the Statistical Analysis of Microarrays, a false-discovery rate of <20% was selected. MiRNAs with >2-fold expression change and a false-discovery rate <20% were considered significantly altered. Global sample variation was assessed by principal components analysis. MiRNAs that were identified as being significantly different were than queried in miRDB ( mirdb.org is an online database for miRNA target prediction and functional annotations) to identify potential gene targets.
MiRNA array validation and miRNA quantification by QPCR
To confirm the miRNA array results, we used the same samples (V2) that were used for the array and performed QPCR. Validation of the miRNA array was performed in V2 samples (term birth, 10; preterm birth, 10 preterm) with SYBR Green real-time polymerase chain reaction (Life Technologies), as previously described. This same method was used to assess alterations in miRNA expression in the RNA PAP samples that had been collected at an earlier time point (V1). In addition, the same method for QPCR was used to investigate miRNA expression in the in vitro studies. Briefly, complementary DNA (cDNA; 800 ng to 1 μg) was synthesized with the miScript II Reverse Transcription Kit (Qiagen, Valencia, CA), per the manufacturer’s instructions. SYBR Green-based miScript Primer assays were performed using microRNA specific primers (Qiagen). QPCR reactions were completed, in triplicate wells (n = 3) on the Applied Biosystems 7900HT Sequence Detection System (Life Technologies) according to the maufacturer’s protocol and as previously published by our group for miRNA investigations. The ΔΔCT method was used for relative expression quantification with the use of RQ manager software (version 2.4; Life Technologies). The endogenous reference gene RNU6B (MS00014000) was used for miRNA quantification for both the human samples and the in vitro studies, as previously reported. All primer sets were purchased from Qiagen: miR-145 (MS00003528), miR143 (MS00003514), miR-199a-3p//miR-199b-3P (MS00007602), miR-199b-5P (MS00003731), miR-140-5P (MS00003500), miR-338-5P (MS00009478), miR-148b (MS00031458), miR-106b* (MS00008400), miR-181a-1//miR-181a-2 (MS00008827), miR-19a (MS00003192), miR-128-1//miR-128-2 (MS00008582), miR-3176 (MS00020860).
JAM-A quantification in v2 samples
RNA was extracted from the V2 samples (preterm birth, 8; term birth, 10) with TRIzol reagent (Invitrogen, Carlsbad, CA), and the concentration was determined with a NanoDrop 2000 Spectrophotometer (Nanodrop TM Rockland, DE). CDNA was synthesized with random hexamers and the High Capacity cDNA Reverse Transcription Kit (Applied Biosystems). Specific primers JAM-A were purchased from Applied Biosystems (Foster City, CA). To account for potential difference in starting material, 18S recombinant RNA was also quantified (Applied Biosystems). The target and 18S QPCRs were performed on the sequence detector polymerase chain reaction machine (Applied Model 7900, PA; Applied Biosystems), as previously described. Each reaction occurred in separate tubes and was performed in triplicate. Relative quantification of 18S recombinant RNA and target mRNA was calculated with the comparative cycle fitted to a 5-point standard curve. The standard curve was generated from cDNA aliquots from each assayed sample. JAM-A expression levels were normalized with respect to 18S RNA levels.
Statistical analysis for changes in miRNA and mRNA expression
Statistical analyses of all miRNAs that were analyzed from V1 and 2 samples and the in vitro studies (ectocervical cells) were performed in a similar manner. For data that were normally distributed, t tests were used. If the data were not distributed normally the Mann-Whitney test was used. Statistical significance was defined as a probability value of < .05. For all QPCR data, the fold change for miRNA expression is noted and represents a ratio between the experimental mean and the control mean. For the human samples, this fold represents the change in women with preterm birth compared with term. For the in vitro studies, the fold change is the ratio from lipopolysaccharide that was exposed to saline solution.
Results
Clinical demographics
The outcomes for the parent study are described elsewhere. For this investigation, we performed a nested case-control study by identifying women who had a spontaneous preterm birth (<37 weeks of gestation) and who had term deliveries for a total of 20 women. CL was significantly different at V2 in those women who had a preterm birth compared with control subjects. Similarly, the use of 17-alpha hydroxyprogesterone caproate was higher in women who ultimately had a preterm birth. There were no other significant differences between the 2 groups ( Table 1 ).
Demographic variable | Term birth (n = 10) | Preterm birth (n = 10) | P value a |
---|---|---|---|
African American race, n (%) | 7 (70.0) | 8 (80.0) | .61 |
Maternal age, y b | 31.5 (29–33) | 23.5 (23–37) | .23 |
Use of 17-alpha hydroxyprogesterone caproate, n (%) | 2 (20.0) | 7 (70.0) | .025 |
Previous preterm birth as indication for enrollment into parent study, n (%) c | 4 (40.0) | 9 (90.0) | .057 |
Gestational age at delivery, wk b | 40 (39–41) | 33.5 (28–36) | < .0001 |
Clinical chorioamnionitis, n (%) | 1 (1.0) | 0 | .31 |
Cervical length b | |||
Visit 1 | 3.67 (3.13–4.01) | 3.25 (2.58–3.39) | .07 |
Visit 2 | 3.83 (3.40–4.14) | 2.89 (2.46–3.21) | .03 |
a Categoric variables compared by χ 2 analyses
b Continuous variables compared by nonparametric comparisons of medians and reported as median (interquartile range)
c Patients were enrolled into the parent study for a history of a previous spontaneous preterm birth or second-trimester loss.
miRNA array of results (V2 samples)
Of the 5640 miRNAs that were analyzed on the array, 99 miRNAs in the cervical cells differed between the women who eventually had a preterm birth compared with their term counterparts. These miRNAs had probability values < .008 and a <20% false-discovery rate. Of these miRNAs, 24 had a >2-fold change in expression between spontaneous preterm birth and term birth ( Table 2 ). Of the 24 targets, all but 2 miRNAs were increased in those women who eventually had a preterm birth. The results of principal components analysis can be seen on Figure 1 . Although there is notable variability, there appear to be 2 large clusters that correspond to women who delivered preterm compared with term ( Figure 1 ). With the use of miRDB, when the top 18 differentially regulated miRNAs are investigated ( Table 2 ), there are 9864 possible gene targets. The targets likely to be involved in cervical remodeling are listed in Table 2 .
MicroRNA | Fold change a | P value | Predicted targets, n | Predicted targets of interest for cervical remodeling, inflammation, and/or preterm birth |
---|---|---|---|---|
miR-143 | 12.34 | .0012 | 280 | Claudin 22, TLR-2, Adducin 3, Versican |
miR-145 | 11.50 | .0009 | 542 | JAM-A , MMP16, Cadherin Type 2, Fibronectin |
miR-199a-3P/ miR-199b-3P | 7.20 | .0048 | 296 | Adducin 3, Syndecan 2, Integrins, Endomucin, Fibronectin 1 COX-2 |
miR-199b-5P | 2.40 | .0041 | 294 | Podocallyxin, HIF-1α, Gap Junction Protein α5,Collagen type 19α1, Prostacyclin Synthase |
miR-140-5P | 5.33 | .0035 | 319 | Claudin 22, PR, Mucin 7, MMP13, Collagen Type 4α2, Laminin γ1, |
miR-338-5P | 4.98 | .0047 | 1178 | TLR-4, PR, IL-6, Mucin 7, CCR6 and 7, Integrin β2, MMP16 |
miR-19a | 4.17 | .0007 | 638 | GAP Junction Protein α1, Integrin β8, IL-15, Syndecan 1, TLR-5, Protocadherins |
miR-148b | 4.02 | .0044 | 369 | MMP10, 13 and 15, IL-15, Collagen Type 4α1 and Type 2α1, Integrins α5 and α11 |
miR-106b a | 3.90 | .0030 | 14 | SOCS7, Protocadherin, IL-R associated kinase, |
miR-181a-1/ miR-181a-2 | 3.81 | .0048 | 1065 | IL-1α, IL-2, Claudin 8, TLR-4, Integrin β8, Protocadherins, cAMP Responsive Element Binding Protein |
miR-128-1/ miR-128-2 | 3.69 | .0044 | 498 | Colony Stimulating Factor, Interferon α1, Versican, TLR-8, CCR9, Zona Occuldins 2 , C/EBP, Collagen type 3α1 |
miR-3176 | –3.24 | .0079 | 228 | Collagen Type 4α4, MMP24, Adducin 2, protocadherins, Catenin delta 1 |
miR-374b | 3.06 | .0021 | 1122 | C/EBP-beta, SMAD2 and 6, Adducin 3, MMP14, Collagen 24α1, Mucin 17, IL-10, Claudin 11 |
miR-24-2 a | 3.05 | .0046 | 101 | Hyaluronan Synthase 2, IL-21R, protocadherins |
miR-15a | 3.03 | .0040 | 690 | bFBGF-2, Collagen Type 24 α1 and 12 α1, Claudin 2 and 12, Laminin γ1 |
miR-25 | 2.99 | .0012 | 449 | Desmocollin-2, Collagen (various types) Integrin α5 and α6, Hyaluronan Synthase 2, Claudin 11 |
miR-1260 | –2.99 | .0071 | 263 | MMP16, IL-11, Integrin α5, Keratin 73 &80 |
miR-30e | 2.90 | .0047 | 838 | Il-1α, Collagen Type 13α1, Endomucin, IL-7, Integrin 1α6 |
a Fold change in microRNA expression in cervical cells between patients who did and did not have a preterm birth.

Validation of miRNA array (V2 samples)
Findings on expression arrays require validation with a more specific technique such as QPCR. Therefore, with the same samples, we investigated miRNA expression in the V2 samples using QPCR. We did this validation for 9 of the top miRNAs that were identified by the array ( Table 3 ). QPCR confirmed the significant up-regulation of MiR 143, 145, and 199b-5P in the V2 samples. Several other miRNAs were increased in the same magnitude as found on the array; however, these changes did reach statistical significance with notable variability across samples.
MicroRNA | MicroRNA array | Quantitative polymerase chain reaction validation | ||
---|---|---|---|---|
Fold change a | P value | Fold change a | P value | |
miR-143 | 12.34 | .0012 | 5.20 | .0076 |
miR-145 | 11.50 | .0009 | 5.00 | .0082 |
miR-199a-3P/miR-199b-3P | 7.20 | .0048 | 6.13 | .0653 |
miR-199b-5P | 2.40 | .0041 | 7.90 | .0030 |
miR-140-5P | 5.33 | .0035 | 3.00 | .1220 |
miR-338-5P | 4.98 | .0047 | -1.03 | .8900 |
miR-148b | 4.02 | .0044 | 2.80 | .7304 |
miR-106b a | 3.90 | .0030 | 1.20 | .3712 |
miR-181a-1/miR-181a-2 | 3.81 | .0048 | 1.90 | .2370 |
a Fold change in microRNA expression in cervical cells between patients who did and did not have a preterm birth.
Investigation of miRNA expression earlier in gestation (V1)
We sought to assess whether the miRNAs that were found to be altered at V2 would also be expressed differentially earlier in pregnancy (20-24 weeks of gestation). Therefore, in the cervical cell samples that were collected at the earlier time-point (V1), we used QPCR to assess several of the miRNAs that were altered in V2 by the array ( Table 4 ). MiR-143 and miR-145 were increased 3-fold in the V1 samples but this did not reach statistical significance. Only miR-106b* was increased significantly in the V1 samples in women who ultimately had a preterm birth compared with term. It is important to note that, although several miRNAs were not significantly increased at V1, there was increased abundance of all miRNAs that were tested except miR-3176, which was decreased in samples from women who had a preterm compared with a term birth.
MicroRNA | Quantitative polymerase chain reaction validation | |
---|---|---|
Fold change a | P value | |
miR-143 | 3.26 | .1520 |
miR-145 | 3.06 | .0831 |
miR-199a-3P/miR-199b-3P | 2.14 | .2390 |
miR-199b-5P | 3.20 | .0592 |
miR-140-5P | 2.16 | .1615 |
miR-338-5P | 2.30 | .1672 |
miR-148b | 5.90 | .0927 |
miR-106b a | 1.91 | .0037 |
miR-181a-1/miR-181a-2 | 1.80 | .4894 |
miR-19a | 1.90 | .2991 |
miR-128-1/miR-128-2 | 1.80 | .1516 |
miR-3176 | –1.40 | .7618 |
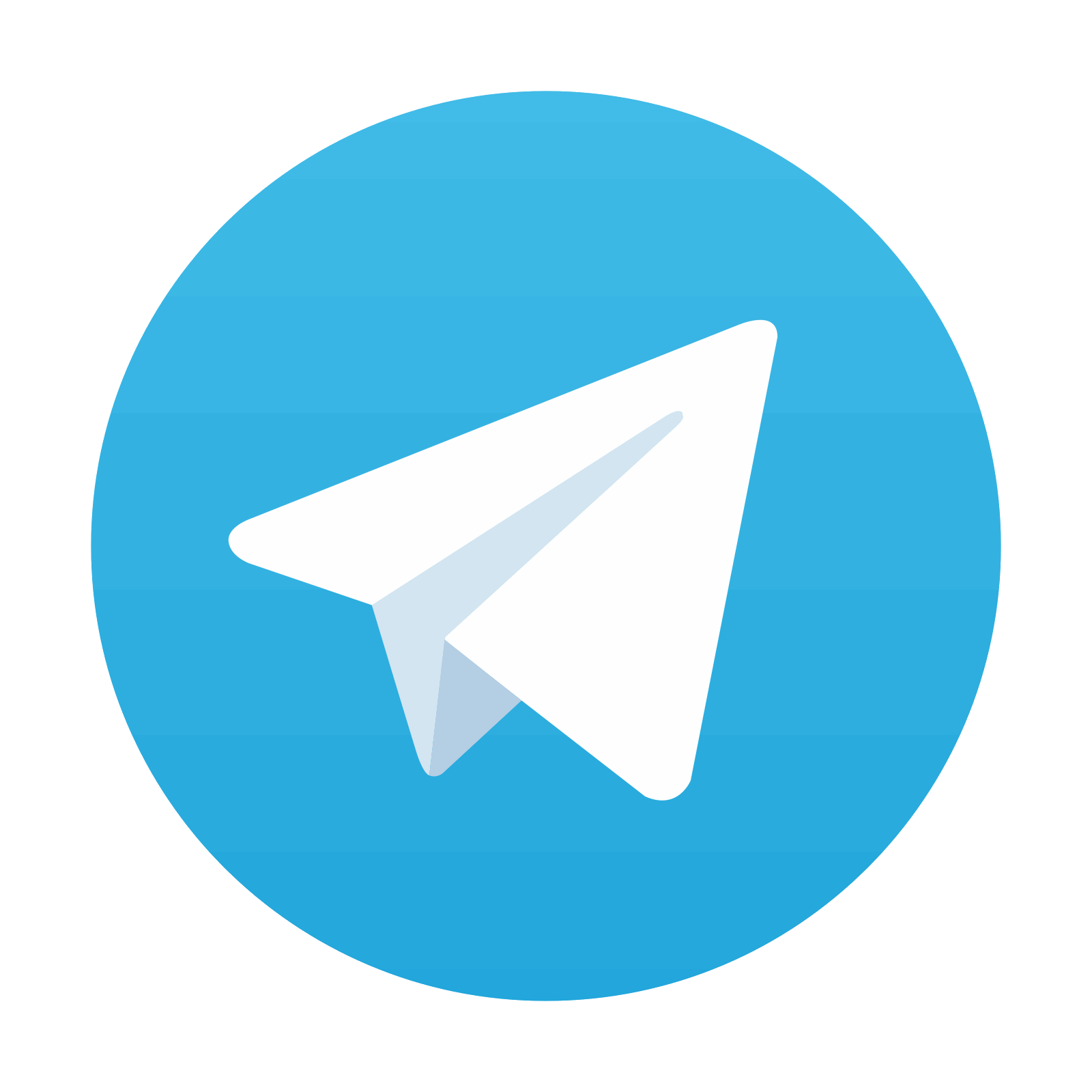
Stay updated, free articles. Join our Telegram channel

Full access? Get Clinical Tree
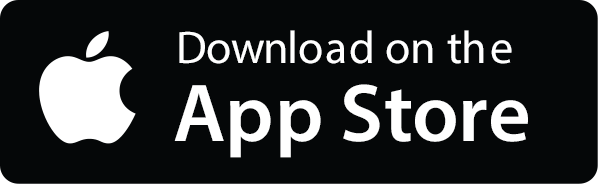
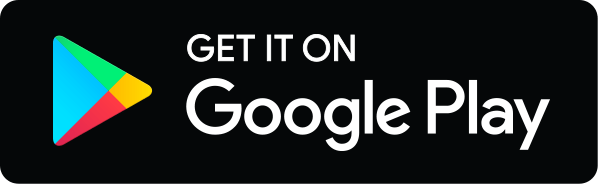
