Fig. 1
(a) PA radiograph measuring radial inclination and (b) Lateral radiograph measuring palmar tilt
Fractures of the Distal Radius Outcome Tools
Distal radius fracture outcomes may be assessed clinically by measuring range of motion and grip strength and by tracking the incidence of complications and the need for repeat manipulation. Radiographic parameters followed include degree of angulation and cast index. The cast index is the ratio of the inner diameter of the cast in the sagittal plane to that in the coronal plane and is associated with the need for repeat manipulation (Fig. 3) (Chess et al. 1994). The Activities Scale for Kids performance (ASKp) version contains 30 items and is validated for self-reporting of physical activity by children ages 5–15 years (Young et al. 1995). The visual analog scale (VAS) is a validated instrument for the assessment of pain (Bijur et al. 2001). Both of these scales have been utilized in studies of pediatric distal radius fractures (Plint et al. 2006).
Fractures of the Distal Radius Treatment Options
The treatment options for distal radius fractures include splint immobilization, cast immobilization, closed reduction and cast immobilization, closed reduction and percutaneous pinning, and open reduction. The indications and contraindications for, and the techniques, outcomes, and complications of nonoperative and operative management are described below.
Nonoperative Management of Fractures of the Distal Radius
Most distal radius fractures are managed nonoperatively. Contraindications to nonoperative management are the same as indications for operative management and are summarized in Table 1. These include open fractures, irreducible fractures, excessive swelling, and risk for or presence of neurovascular compromise. Additionally, displaced Salter-Harris type III or IV patterns and triplane fractures or equivalents require surgical management for anatomic reduction. Nonoperative management is contraindicated in the presence of polytrauma or ipsilateral humerus fracture due to the risk of compartment syndrome. Furthermore, fractures which lose their initial reduction and refractures with displacement often have poor outcomes when managed nonoperatively. Finally, internal fixation for all displaced fractures in patients with less than 2 years remaining until skeletal maturity can be considered due to their reduced capacity for remodeling compared to younger patients.
Table 1
Nonoperative management
Indications | Contraindications |
---|---|
Most non-displaced fractures | Open fractures |
Most Salter-Harris type I or II fractures | Irreducible fractures |
Most greenstick fractures | Neurovascular compromise |
Most torus fractures | Excessive swelling |
Most complete fractures | Displaced Salter-Harris type III or IV fractures |
Plastic deformation injuries | Triplane fractures or equivalents |
Ipsilateral humerus fractures | |
Polytrauma | |
Loss of initial reduction | |
Refractures with displacement | |
Displaced fractures and less than 2 years until skeletal maturity |
Nonoperative management for distal radius fractures entails immobilization with or without closed reduction. Traditionally, immobilization is accomplished with fiberglass or plaster of Paris casting or splinting; however, prefabricated splints and bandage therapy are also used. Both short-arm and long-arm casts are in widespread use. The decision between long-arm and short-arm cast immobilization depends on the displacement of the fracture and age of the patient. This is further discussed in the following sections. Closed reduction is performed with adequate analgesia, usually in the emergency room under conscious sedation. Portable fluoroscopy may be used for guidance and assessment of the reduction. While reduction is typically performed by an orthopedic surgeon where available, many emergency medicine physicians and family practitioners are also trained to evaluate and provide nonoperative management of distal radius fractures.
Non-displaced fractures of the physis and metaphysis with acceptable angulation and rotation may be amenable to immobilization without reduction. Metaphyseal fractures have excellent remodeling potential and up to 10° per year of dorsal-volar angulation may correct with continued growth. The range of angular deformity accepted in practice varies and is clinician dependent. As a general guide, the traditional tolerances are provided in Table 2, adapted from the Rockwood and Wilkins text (Waters and Bae 2010).
Table 2
Acceptable angular deformity for metaphyseal fractures (degrees)
Dorsal-volar | Radial-ulnar | ||
---|---|---|---|
Age (year) | Boys | Girls | Boys and girls |
4–9 | 20 | 15 | 15 |
9–11 | 15 | 10 | 5 |
11–13 | 10 | 10 | 0 |
>13 | 5 | 0 | 0 |
In contrast, rotational deformity will not remodel and is an indication for reduction. Malrotation is often present when both the radius and ulna are fractured and the fracture sites are at two different levels, proximal and distal to each other. Apex-volar angulation is often associated with supination of the distal fragment; apex-dorsal angulation, with pronation. Failure to recognize and reduce rotational deformity is a common pitfall in the treatment of greenstick fractures. The indications and contraindications for immobilization without reduction for distal radius fractures are summarized in Table 3.
Table 3
Immobilization without reduction
Indications | Contraindications |
---|---|
Most torus fractures | Excessive angular deformity (see Table 2) |
Consider for S-H II, <40 % displacement, <20° angulation, child <10 years old | Most displaced physeal fractures |
Consider for complete fractures with bayonet apposition in very young child | Most complete fractures |
Some greenstick fractures | Rotational deformity |
Plastic deformation |
Discussion: Nonoperative Management of Physeal Fractures
Salter-Harris type I and II fractures of the distal radius are typically the result of an extension mechanism and consequently displace dorsally with apex-volar angulation (Fig. 4). Alignment of the fragments is traditionally acceptable with less than 50 % displacement and no angular or rotational deformity (Egol et al. 2010); however, some advocate immobilization without reduction for Salter-Harris type II fractures with less than 20° of angulation and less than 40 % displacement in children under age 10 (Houshian et al. 2004). Physical and occupational therapy are not usually required for pediatric distal radius fractures.
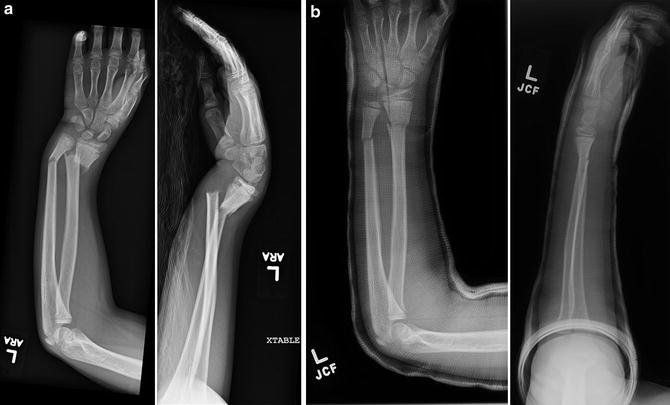
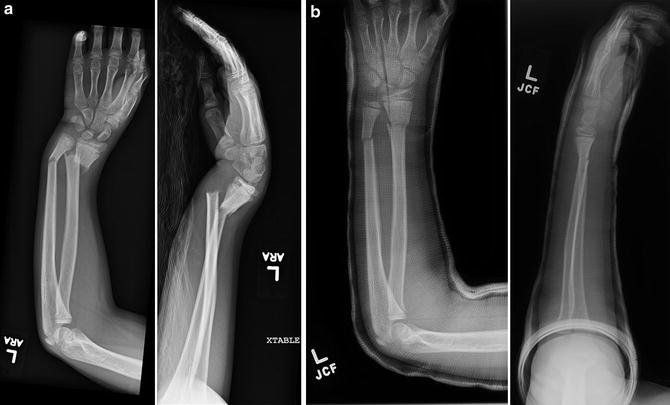
Fig. 2
(a) AP and Lat pre-reduction, and (b) Post-reduction and casting of displaced unstable diametaphyseal fractures of the distal radius and ulna
Technique: Closed Reduction of Physeal Fractures
In Salter-Harris type I and II fractures, the dorsal periosteum is usually intact and can be used as a tension band to aid reduction. Although the thickness of the periosteum limits the utility of pulley-weight traction, finger traps with less than 10 lb of counterweight or an assistant are helpful to support and stabilize the extremity for reduction and casting. The fracture may reduce with traction alone; otherwise, gentle thumb pressure applied at the fracture site in a distal and volar direction facilitates atraumatic flexion of the distal epiphysis (Fig. 5). Alignment of the fragments is traditionally acceptable with less than 50 % displacement and no angular or rotational deformity. Multiple reduction attempts may increase the risk of growth arrest due to increased shear forces across the physis. Immobilization in the neutral position or pronation is recommended. Portable fluoroscopy, if available, could be used to immediately assess the reduction before immobilization. Irreducibility is most often due to entrapment of the periosteum or pronator quadratus.
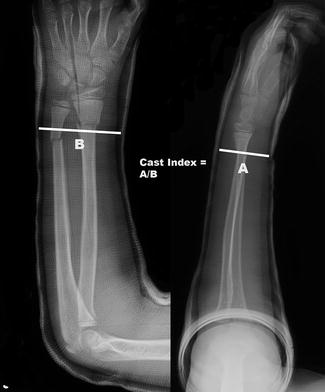
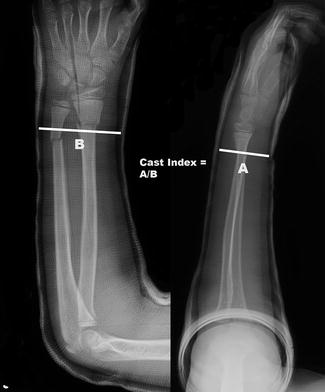
Fig. 3
The cast index is measurement of the inner diameter of the cast in the sagittal plane (a) divided by that in the coronal plane (b) ideally being less than 0.8
Discussion: Nonoperative Management of Torus Fractures
The traditional standard of care for torus fractures is immobilization in a short-arm cast for 3–4 weeks. The theoretical benefits of casting are protection against pain, displacement, and refracture. Torus fractures usually do not require reduction, are inherently stable, and have little risk of late displacement; accordingly, alternatives to cast immobilization have been studied (Bae and Howard 2012). Treatment with removable splints may reduce the amount of clinic visits required, enables easier bathing, and avoids cast-saw-related anxiety. Several prospective, randomized controlled trials have compared removable wrist splints to casts. Davidson et al. reported successful healing with no complications in all patients treated with splinting or casting (Davidson et al. 2001). In addition, Plint et al. found no difference in pain on the VAS between the two groups. The splint group had significantly better ASKp scores at one of four time points assessed, suggesting that children have less difficulty with activities while in splints compared to while in casts (Plint et al. 2006). In contrast, Oakley et al. found that patients treated with a volar slab splint had longer durations of pain and longer times until resumption of normal activity (Oakley et al. 2008). Splint management has been found to reduce total cost per patient by approximately $73–82, with the majority of savings due to attending one fewer clinic visit for cast or splint removal (Davidson et al. 2001; von Keyserlingk et al. 2011).
Soft casts are the preferred treatment in some institutions. Trials by Khan et al. and Witney-Lagen et al. reported full recovery, according to parental evaluation, in both soft cast and rigid cast treatment groups (Khan et al. 2007; Witney-Lagen et al. 2013). Parents may safely remove precut plaster back slabs (Symons et al. 2001) and soft casts (Khan et al. 2007) at home after 3 weeks if adequate explanation of removal is provided during the initial treatment. Most families prefer removal at home rather than in the clinic and prefer soft casts to rigid casts. Thus, although soft cast material is more expensive than rigid cast material, removal at home contributes to decreased total cost of care for both soft casts and splints.
Studies have also demonstrated satisfactory outcomes in the management of torus fractures with bandage therapy. West et al. randomized patients to either casting or treatment with a layer of orthopedic wool covered by crepe bandage and held in place with tape. They reported a decreased incidence of pain and decreased duration of pain in the bandage group; however, a validated pain scale was not used. Fracture healing was universal, and the bandage group had significantly greater range of motion compared to the cast group, as measured on the day of cast removal at 4 weeks (West et al. 2005). Kropman et al. performed a similar trial, and there were no complications in either group. In contrast to the findings of West et al., they reported that VAS pain scores were significantly increased in the bandage group during the first week of treatment. The cast group experienced more discomfort (i.e., itching). Range of motion was reduced in the casting group on the day of cast removal; however, there was no significant difference 2 weeks later. In one center, bandage therapy was made the standard of care for torus fractures, and no secondary angulation or refractures occurred in 49 consecutively treated patients (Vernooij et al. 2012).
In conclusion, there are several treatment options for torus fractures, which are inherently stable injuries. Although the traditional standard of care is rigid immobilization in a short-arm cast for 3–4 weeks, the literature supports treatment in a wrist splint or soft cast that can be removed at home after 3 weeks with no radiographic follow-up. Additionally, a growing body of evidence suggests that bandage therapy is safe and effective. The use of splints, soft casts, and bandages decreases the workload of the fracture clinic and reduces the cost of care. While most parents of children treated with splints, soft casts, and bandages would prefer them to rigid casts, many parents may be hesitant to forego traditional rigid immobilization and radiographic follow-up. Thus, treatment of torus fractures should be the result of a shared decision-making process involving the patient, parents, and physician and should consider any special needs of the child and the impact on the family of attending a follow-up visit. Finally, it warrants mentioning that errors in torus fracture diagnosis are not uncommon and are typically due to failure to recognize a greenstick fracture (Fig. 6). Therefore, review of the radiographs by an experienced physician is mandatory.
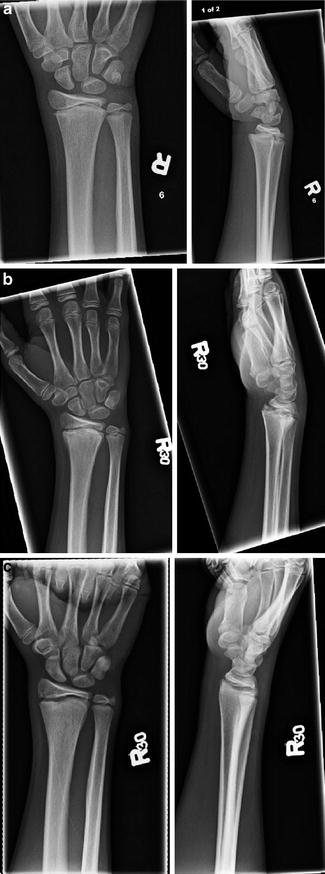
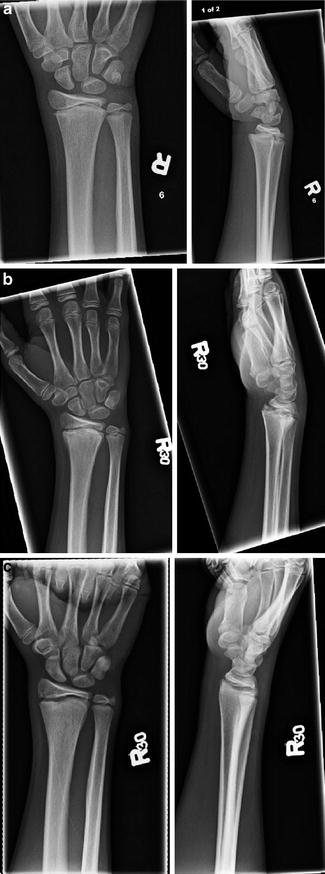
Fig. 4
(a) AP and Lat of a Salter-Harris II fracture of the distal radius with dorsal displacement and mild angulation (b) AP and Lat at 6 weeks and (c) at 4 months
Discussion: Nonoperative Management of Greenstick Fractures
Greenstick fractures typically present as failure of the volar cortex in tension and the dorsal cortex in compression. Radial displacement and apex ulnar angulation are often present. Traditional tolerances for angular deformity are shown in Table 2. Fractures which are minimally angulated on presentation may be immobilized without reduction (Al-Ansari et al. 2007; Do et al. 2003). However, excessive angulation presents a risk of lost forearm rotation and should be corrected to maintain the interosseous space.
Rotational deformity is also common, especially when there is an associated ulna fracture , and correction of malrotation is essential to achieving anatomic alignment. However, rotational correction often fractures the intact cortex, thus completing the fracture. Intentional completion of the fracture is controversial, and little data is available to evaluate this practice. Some argue that fracture completion decreases the risk of redisplacement; however, some evidence suggests that the opposite may be true (Waters and Bae 2010; Schmuck et al. 2010). Nevertheless, fracture completion appears to be useful when primary angulation exceeds remodeling capacity.
Clinical practice varies regarding the position and type of forearm immobilization. Some advocate for immobilization in supination to minimize the deforming effect of the brachioradialis; in the neutral position, to maintain the interosseous space and rotational range of motion; and in pronation, to reduce the common supination deformity (Waters and Bae 2010). Boyer et al. found no difference in angulation in patients randomized to supination, neutral position, or pronation (Boyer et al. 2002). Local practice determines the choice of long-arm cast, short-arm cast, or splint (Bae and Howard 2012). Classically, a long-arm cast is applied for 3–4 weeks, followed by a short-arm cast for 1–3 weeks. Elbow immobilization is thought to decrease the risk of displacement by limiting the child’s activities and reducing the deforming effect of the brachioradialis (as in supination). However, in trials by Bohm et al. and Webb et al., there was no difference in the rate of lost reduction between patients randomized to short-arm casts or long-arm casts after closed reduction (Bohm et al. 2006; Webb et al. 2006). Moreover, patients treated with a long-arm cast were more likely to require assistance with activities of daily living and missed, on average, one more day of school than those treated with a short-arm cast. Additionally, Boutis et al. found no significant difference between patients randomized to short-arm casts or prefabricated wrist splints in complication rate, ASK scores, grip strength, range of motion, and radiographic measurement of angulation (Boutis et al. 2010).
In conclusion, most greenstick fractures are treated with closed reduction and rigid immobilization, but the acceptable angulation, position, and type of immobilization vary in clinical practice. Failure to recognize and reduce rotational deformity is a common pitfall in the treatment of greenstick fractures. While long-arm casts are the traditional standard of care, the literature supports the use of well-molded short-arm casts. Redisplacement is common, and weekly radiographic follow-up is indicated until there is evidence of sufficient callus formation.
Technique: Closed Reduction of Greenstick Fractures
Correction of malrotation is essential to achieving anatomic alignment in greenstick fractures. Finger traps are not used. While pressure is applied to the apex of deformity, the patient’s thumb should be rotated toward the apex of angulation. In other words, apex-volar fractures require pronation of the distal fragment and apex-dorsal, supination. Fracture of the intact cortex during this maneuver may occur but is not necessary. Angular deformity should be corrected to fewer than 10°. Maintenance of alignment may be aided by immobilizing the forearm in the rotational position used to achieve the reduction. While portable fluoroscopy, if available, should be used to assess the reduction, postreduction radiographs should include the entire forearm to facilitate evaluation of malrotation.
Discussion: Management of Complete Fractures
Complete fractures of the distal radius often present with associated ulna fracture s, are usually dorsally displaced, and are frequently in bayonet apposition. In other words, the distal fragment frequently lies in a side-to-side rather than end-to-end relationship to the proximal fragment. The traditional standard of care is closed reduction and cast immobilization. Regardless of the presence of an associated ulna fracture, reduction is difficult to maintain. The rate of redisplacement following an initial, acceptable closed reduction has been reported between 21 % and 91 %, but in most studies, it is approximately 25 %. The greatest risk factors for redisplacement are initial angulation greater than 30°, incomplete reduction, and complete displacement, although displacement greater than 50 % also increases this risk (Zamzam and Khoshhal 2005; Alemdaroglu et al. 2008; McQuinn and Jaarsma 2012).
Loss of reduction may lead to a malunion, which has excellent potential for remodeling in the dorsal-volar plane (see “Pathoanatomy and Applied Anatomy Relating to Fractures of the Distal Radius” section). However, deformity in the radial-ulnar plane has less potential for remodeling (see Table 2). Repeat manipulation may be indicated following loss of reduction to avoid a malunion. One should use caution not re-manipulate a physeal fracture after 7–10 days of injury as this carries a higher risk of arrest. For their study, Alemdaroglu et al. (2008) defined redisplacement as dorsovolar angulation of 10° or greater, radioulnar angulation of 5° or greater, translation of 3 mm or greater, or the combination of dorsovolar angulation of 5° or greater and translation of 2 mm or greater. The authors remanipulated fractures that had dorsovolar angulation of greater than 20°, radioulnar angulation of greater than 10°, translation of greater than 4 mm, or any combination of two of the following: dorsovolar angulation of greater than 10°, radioulnar angulation of greater than 5°, and translation of greater than 3 mm (see Table 4).
Redisplacement | Remanipulation | |
---|---|---|
Dorsovolar angulation (degrees) | ≥10 isolated, ≥5 in combination | >20 isolated, >10 in combination |
Radioulnar angulation (degrees) | ≥5 isolated | >10 isolated, >5 in combination |
Translation (mm) | ≥3 isolated, ≥2 in combination | >4 isolated, >3 in combination |
Some surgeons advocate conservative management to take advantage of the tremendous remodeling potential of the distal radius. Do et al. (2003) found that fractures with less than 15° of dorsovolar or radioulnar angulation and less than 1 cm of shortening heal without deformity or clinical sequelae. The average time to bony healing and cast removal was 6 weeks, and remodeling was complete after an average of 4 months (up to 13 months in older children). Moreover, Crawford et al. (2012) reported excellent results in 51 consecutive children with fractures in bayonet apposition treated with no attempt at anatomic reduction. Within 72 h of injury, in an outpatient clinic with no analgesia or sedation, short-arm casts were applied and gently molded to correct angulation, leaving the fractures overriding and shortened. The average dorsovolar and radioulnar angulation after reduction were 4.0° and 3.2°, respectively, (range 0–13, 0–10) and at follow-up after 1 year were 2.2° and 0.75° (range 0–10, 0–5). All patients progressed to union and full range of wrist motion, there were no complications, and “only a few patients had a minimally noticeable clinical deformity.” The cost of care for this approach was approximately one-fifth that of closed reduction with conscious sedation and approximately one-eighth that of percutaneous pin fixation with general anesthesia. The authors advocate for this approach as first-line treatment, noting that it avoids the risks of anesthesia, lessens the time required by the treating physician, and reduces the cost of care.
In conclusion, the traditional standard of care for complete fractures of the distal radius is closed reduction and cast immobilization with close radiographic follow-up until there is evidence of healing (typically 6 weeks). Loss of reduction is common, and repeat manipulation may be indicated to avoid a malunion. The literature supports primary percutaneous pin fixation as an alternative for fractures at high risk of displacement or when excessive swelling is present to reduce the risk of neurovascular compromise. However, these fractures have excellent remodeling potential, and good results have been obtained by correcting angular deformity with gentle cast molding and allowing healing to occur in an overriding, shortened position. Thus, treatment should be guided by a shared decision-making process involving the patient, parents, and physician with consideration of the risk of loss of reduction based on the patient’s age and fracture characteristics.
Technique: Closed Reduction of Complete Fractures
Finger traps with weight of less than 10 lb may be useful to stabilize the hand during casting, but the intact periosteum will not usually stretch to permit reduction through traction, and the tense periosteum may hinder reduction (Fig. 7). Eichinger et al. (2011) described a traction technique designed to provide greater mechanical advantage by securing the patient’s arm beneath the surgeon’s thigh. Regardless of the traction technique employed, the key to anatomic reduction is initial exaggeration of the deformity (usually dorsal displacement and apex-volar angulation of the distal fragment) [see Video 1]. The dorsum of the hand is often brought to an acute angle with the dorsum of the forearm. Thumb pressure is then applied to distract the distal fragment (Fig. 8). Next, the distal fragment is flexed volarly to obtain reduction, and at the same time, malrotation is corrected if present. Finally, residual translation is corrected through “toggling” the distal fragment by slight dorsiflexion and volarly directed thumb pressure. Portable fluoroscopy, if available, should be used to immediately assess the reduction before immobilization.
