During gestation week 3, pluripotent ectodermal cells proliferate along the surface of the embryo to form a plate that is termed the primitive streak. The cranial end of the primitive streak develops a nodular “mass” of rapidly proliferating cells; this is the primitive node (Hensen node). The primitive node secretes molecules that control the early stages of neural induction. A small depression (the primitive pit or the neuropore) that is contiguous with a groove in the primitive streak develops in the primitive node. At approximately the sixth embryonic day, cells migrate from the primitive node in a cephalad direction to form the notochordal process, which eventually develops into the notochord. The notochord is involved in the formation of the vertebral column.
The notochord induces a thickening of the dorsal ectoderm that results in the formation of the neural plate. By the end of the third week of gestation, the lateral portions of the neural plate are thickened and elevated, thereby forming the neural folds. The thinner midline portion of the plate forms the neural groove. The neural folds progressively bend dorsally along the entire length of the neural groove, eventually fusing in the midline to form the neural tube. The Pax3 gene has a role in neural tube fusion.
Neurulation is the process of spinal cord development in which the neural plate forms from neural ectoderm and then folds into a neural tube. Fusion of the neural folds begins in the upper cervical region and proceeds in both cephalad and caudal directions. As fusion occurs, a continuous ectodermal covering forms over the neural structures. The anterior neuropore is the most cephalad segment of the neural tube; this closes at approximately gestation day 24 at the lamina terminalis. The posterior neuropore is the caudal end of the neural tube; this closes in the region of the future lumbar spine at approximately day 26 or 27.
Separation of the neural tube from the overlying ectoderm is termed disjunction. As this separation is occurring, the overlying ectoderm fuses at the midline, dorsal to the closed neural tube. Perineural mesenchyme migrates into this space between the neural tube and the ectoderm and surrounds the neural tube; this tissue (paraxial mesoderm) eventually forms the meninges, the vertebrae, and the paraspinal muscles.
The distal portion of the spinal cord forms by the processes of canalization and retrogressive differentiation (secondary neurulation). The remnant of the primitive streak at the caudal pole of the embryo gives rise to an aggregate of undifferentiated cells, termed the caudal cell mass. This tissue extends between the posterior neuropore and the cloacal membrane in the tail fold of the embryo. The caudal cell mass gives rise to neural tissue and vertebrae caudal to the posterior neuropore. The resulting solid cord of cells in the lumbosacral region develops small cavities that coalesce to form a central lumen, which ultimately becomes contiguous with the lumen of the neural tube.
At approximately gestation day 38, apoptosis leads to decrease in the size of the cell mass in the caudal aspect of the neural tube. The major portion of the caudal aspect of the cord thereby undergoes involution. This process of retrogressive differentiation results in formation of the distal portion of the conus medullaris, the filum terminale, and the ventriculus terminalis (a focal dilatation of the central canal that is contained in the conus medullaris). The transition zone between those portions of the cord formed by primary neurulation and secondary neurulation is the most common location of dysraphic lesions.
The tip of the conus medullaris is in the sacrococcygeal region early in embryogenesis, and “ascends” to the L2-3 level by term. By 3 months of age, the conus is at a normal adult level (L1-L2). As a rule, a conus location at or below L3 is considered to be abnormal. The filum terminale should measure less than 2 mm in diameter (Figure 22-1).
Figure 22–1
Normal filum terminale.
The filum terminale internum is a fibrous band that extends from the apex of the conus medullaris to the inferior aspect of the thecal sac. On sonography, the filum appears as a thin echogenic band (arrows on this image of a 22-day-old infant). Real-time observation usually shows a wafting motion of the filum during respiration. The filum terminale externum continues inferiorly and attaches to the first segment of the coccyx; this portion is not discernible on ultrasound.

During week 3 of embryogenesis, the paraxial mesoderm (which is located lateral to the notochord and beneath the neural plate) thickens to form a pair of longitudinal strips.
This tissue segments into paired blocks of tissue called somites. The first somites appear at days 19 to 21 in the future cervical region; additional somite formation occurs caudally as the embryo lengthens. Eventually, between 42 and 44 pairs of somites develop; the first occipital and several of the coccygeal somites eventually regress. The somites subdivide into dorsolateral components termed dermatomyotomes and ventral medial portions termed sclerotomes. The sclerotomal cells form the primordia of the cartilage, bones, and ligaments of the vertebrae and ribs. The dermatomyotomes form the dermis and subcutaneous tissues of the back and the dorsal skeletal muscle myocytes of the trunk.1
Stages of vertebral development include formation of the membranous (mesenchymal) vertebral column, formation of the cartilaginous vertebral column, and ossification of the vertebral column. This process begins in the future occipital region, and progresses caudally. During the membranous stage of spinal development, there is migration of sclerotomal cells to form the membranous anlagen of the vertebrae and ribs, that is, a scaffold for bone development. At approximately day 25 of gestation, the notochord separates from the ectoderm and endoderm. At this stage, there are 2 zones: a ventral subchordal zone between the notochord and the endoderm, and a dorsal epichordal zone between the notochord and the neural tube. As this separation occurs, sclerotomal cells migrate medially into both the subchordal and epichordal zones, thereby surrounding the notochord and neural tube. These cells unite to form a dense longitudinal column of mesenchyme that is termed the perichordal sheath. This process begins in the cervical region and progresses caudally.
The perichordal sheath retains a segmental configuration, with each sclerotome level consisting of densely packed cells caudally and loosely arranged cells cranially that surround the intersegmental arteries. These layers of cells are the precursors of the vertebral bodies and intervertebral discs. As the superficial ectoderm separates from the closed neural tube, sclerotomal cells complete their migration around the dorsal aspect of the neural tube; these cells eventually form the membranous neural arches of the vertebrae. This tissue is also the precursor of the meninges and paraspinous muscles. Cells derived from the ventrolateral aspects of the sclerotomes give rise to the membranous costal processes of the vertebrae and the membranous ribs.
Beginning on approximately day 24, division and resegmentation occur in the membranous vertebral bodies. The cells of the caudal half of each sclerotome proliferate more rapidly than those in the cranial half. Clefts develop at the midportion of each sclerotome (fissures of von Ebner). The sclerotomes then separate along these clefts and reunite in a complex fashion, such that the dense caudal portion of one sclerotome unites with the more loosely packed cells of the next most caudal sclerotome; this results in formation of new “pre-cartilaginous” primitive vertebral bodies. This process of resegmentation also causes entrapment of the intersegmental arteries in the central portions of the new vertebral bodies. In infants, the site of sclerotome fusion is often visible on lateral radiographs of the spine as a lucent band across the midportion of each vertebral body.
Some of the cells from the denser (caudal) half of the sclerotome contribute to the formation of the annulus fibrosus of the intervertebral disc and the endochondral growth plates of the vertebral centra. This fusion proceeds bilaterally and symmetrically at each segment, such that cleavage and fusion of the sclerotomes on each side form the ipsilateral half of each vertebral body. This process begins in the lower cervical/upper thoracic region and proceeds both cranially and caudally.
During resegmentation and fusion of the membranous vertebral bodies, the notochord also undergoes changes. Initially, the notochord has a uniform caliber throughout its length. During formation of the membranous vertebrae, the cells of the notochord that are located in the region of the future centrum become compressed and undergo degeneration. The cells in the region of the future intervertebral disc proliferate and undergo mucoid degeneration. This portion of the notochord forms the nucleus pulposus of the intervertebral disc. Additional notochordal tissue persists as the apical ligaments of the dens and as occasional remnants in the skull base, sacrum, and vertebral bodies.
The chondrification stage of vertebral development begins with the appearance of centers of chondrification in the mesenchyme of the membranous vertebral column on approximately day 39. Molecules secreted from the adjacent notochord and ventral neural tube induce chondrification. The process of chondrification begins at the cervicothoracic junction and extends both cranially and caudally. Chondrification occurs earlier in the centrum than in the neural arches. In the centrum, 2 centers of chondrification appear, 1 on each side of the midline. A chondrification center also develops in each half of the neural arch lateral to the neural tube. Each of these centers later fuses with its mate to form the chondral neural arch and the spinous process. Two additional centers of chondrification appear at the junction of the neural arch with the centrum; these centers extend laterally into the transverse processes. Therefore, 3 pairs of chondrification centers develop in each vertebra.
The process of ossification overlaps that of chondrification. The primary ossification stage begins at gestation week 8 or 9. Each neural arch develops 2 primary ossification centers, 1 on each side. Ossification of the arches begins in the cervical and thoracic regions and extends caudally. Fusion of the laminae into complete bony arches begins in the lumbar region and progresses cranially.
Ossification of the vertebral bodies begins in the lower thoracic/upper lumbar region, with subsequent progression cranially and caudally. Initially, each vertebral body centrum develops 2 primary ossification centers, 1 anterior to the vestigial mucoid streak of the notochord and 1 posterior to this structure. These centers soon fuse into a single ossification center that progressively enlarges. The residual unossified cartilage cephalad and caudal to the ossification center in the centrum then forms 2 cartilaginous plates adjacent to the intervertebral discs. The annular (ring) epiphysis arises from a C-shaped cartilaginous ring that develops at the ventral and lateral aspects of the interspace between the centrum and the disc.
The eventual fusion between the neural arch and the centrum occurs anterior to the pedicles at the site of the neurocentral synchondrosis. Consequently, the vertebral body is composed of bone derived from the ossification centers of the arch as well as those of the centrum. The neurocentral synchondroses begin to fuse in the cervical region at approximately 3 years of age. This fusion progresses inferiorly to the lumbar region and is complete by approximately 6 years of age. Conversely, the posterior neural arches fuse in the lumbar region during the first year of life, with the fusion progressing cephalad. The cervical laminae are the last to fuse. This occurs during the third year.
Development and maturation of the vertebral column after birth affect the radiographic appearance. The vertebral bodies of the neonate tend to be rectangular in the thoracic area and oval in the lumbar region. A bone-within-bone appearance is common during the first 2 months of life. The thoracic and lumbar vertebral bodies of young children have well-defined anterior and posterior indentations. The posterior indentations are due to normal vascular structures. This posterior notch is present throughout the life, but is radiographically most prominent in young children. The anterior vertebral body indentation is caused by alterations in radiodensity of different components of the developing vertebral body. At birth, the synchondroses between the ossified halves of the posterior arches are readily visible on frontal radiographs as midline linear vertebral radiolucencies. The costovertebral synchondroses (between the ribs and the neural arches) and the neurocentral junctions (between the centra and arches) are also visible.
Between the ages of 7 and 10 years, formation of secondary ossification centers begins in the annular epiphyses (rim apophyses; ring epiphyses) at the superior and inferior margins of the vertebral bodies. Each is formed from 2 to 20 separate nuclei. Before fusion, the multicentric nature of ossification of the annular epiphyses results in irregularity on frontal radiographs that is most prominent in the mid-thoracic and mid-lumbar regions. On lateral radiographs, the cartilaginous epiphysis appears as a step-like defect in the vertebral body. The defect is deep along the anterior surface and shallow posteriorly. As ossification occurs, irregular foci of calcification appear in the bony defect, as linear or wedge-shaped fragments (Figure 22-2). Care must be taken to avoid confusing these irregularities with fractures or other pathological entities. Fusion of the annular epiphyses with the vertebral bodies occurs between 20 and 25 years of age.
At about the time of puberty, secondary ossification centers develop at the tips of the transverse processes, at the tips of the spinous processes, and in the annular epiphyses. Each lumbar vertebra has 2 additional secondary ossification centers for the mamillary processes. Complete fusion of these secondary centers does not occur until approximately 25 years of age; therefore, these ossification centers are visible radiographically in teenagers.
A coronal vertebral body cleft can result from persistence of separate ventral and dorsal ossification centers for the centrum. This anomaly is more frequent in males than females. The thoracolumbar region is the most common site. This is a common transient radiographic finding in young infants. Coronal cleft vertebrae, sometimes multiple, occasionally occur in association with other anomalies, such as myelodysplasia and imperforate anus. Prominent coronal clefts composed of cartilage are present in the vertebral columns of infants with many of the forms of chondrodysplasia punctata; these become less pronounced with skeletal maturation.
Sagittal vertebral body clefts in young infants are related to separate ossification centers within each of the paired chondrification centers. These usually disappear during the first several months of infancy. Persistence of a sagittal cleft (i.e., failure of fusion) results in a butterfly vertebra.
The development of the atlas (C1) and axis (C2) differs from that of the remainder of the vertebral column. During the mesenchymal stage of development, the atlas arises from the fourth occipital and first cervical sclerotomes. Ossification of the atlas occurs from 3 primary centers, 1 for each lateral mass (appearing at the end of month 2 of gestation) and 1 for the anterior arch (appearing in the first year of life). The posterior arch of the atlas is ossified by extension from the lateral masses. The 3 ossification centers are demarcated by open synchondroses in infants and young children. The neural arches fuse posteriorly by 3 to 5 years of age and fusion between the anterior arch and the 2 neural arches usually occurs by 7 years of age. Failure of development of the anterior ossification center of C1 results in a radiographically identifiable anterior defect that is of no clinical significance.2
The odontoid process, which developmentally represents the body of the atlas, primarily arises from the caudal half of the fourth occipital sclerotome and the cranial half of the first cervical sclerotome. The apex of the odontoid process develops from the cranial portion of the fourth occipital sclerotome. The body and neural arch of the axis arise from the first and second cervical sclerotomes. The odontoid process forms from side-by-side ossification centers that appear during the fifth or sixth months in the fetus, and fuse by 1 year of age. If these ossification centers fail to fuse at the midline, a bifid dens results. A secondary ossification center appears at the tip of the odontoid process between 3 and 6 years of age and usually fuses with the odontoid by 12 years of age; this is the os terminale. Failure of osseous fusion results in the clinically inconsequential ossiculum terminale persistens.
In infants, there is separation of the odontoid process from the body of the axis by a disc-shaped cartilaginous plate that persists throughout infancy into early childhood. The odontoid process usually begins to fuse with the body of the axis between the ages of 3 and 6 years; the subdental synchondrosis is visible radiographically until approximately 11 years of age. Ossification begins at the periphery of the plate and progresses toward the center; a portion may remain cartilaginous until early adulthood. Failure of complete ossification of this plate results in the appearance of the dens as a separate bone, the os odontoideum.
In addition to the ossification center for the dens, the axis typically develops from 3 primary ossification centers, in a similar manner to the other cervical vertebrae. One ossification center is located in the body and 2 in the neural arches. Fusion of these primary centers occurs between the ages of 3 and 6 years. The third through seventh cervical vertebrae also have 1 ossification center for the body and 1 for each half of the neural arch. Ossification of the posterior synchondrosis typically occurs between the ages of 2 and 3 years. The neurocentral synchondroses unite by 3 to 6 years of age. Secondary ossification centers may arise at the anterior portions of the transverse processes; at C7, these secondary ossification centers can lead to the formation of cervical ribs.
On imaging studies of children, the distance between the anterior aspect of the dens and the posterior aspect of the anterior ring of the atlas (the atlantodens interval) should be 5 mm or less with neutral or flexion positioning and 4 mm or less in extension positioning. Motion during flexion and extension should be less than 3.5 mm. In adolescents and adults, the normal atlantodens interval is ≤3 mm. Motion at the occipitoaxial joint during flexion and extension maneuvers should be no more than 4 mm.
On lateral flexion radiographs of normal young children, marked widening can occur between the spinous processes of C1 and C2. Throughout the remainder of the cervical spine, the posterior intraspinous distance should be no more than 1.5 times greater than the distance at 1 level either above or below.
On frontal radiographs of young children, the lateral margins of the atlas frequently project beyond the axis; this may simulate a Jefferson fracture. Up to 6 mm of overlap is normal in children up to 7 years of age. An additional normal variation in children at this level is overriding of the anterior arch of the atlas on the odontoid process as viewed on lateral extension radiographs.
The cervical vertebral bodies have an oval appearance in infants. There is progression to a rectangular configuration during early childhood. Ossification of the annular epiphyses in older children produces thin densities that parallel the endplates (Figure 22-3). The anterior aspects of the vertebral bodies in infants and young children are relatively short, and the superior aspects have sloped appearances, producing a configuration of anterior wedging. Anterior wedging of up to 3 mm is normal. This finding is usually most prominent in the third cervical vertebrae (Figure 22-4).
Widening of the prevertebral soft tissues is a common normal radiographic finding in infants and young children. The thickness of the soft tissues on lateral radiographs increases greatly with neck flexion, expiration, and swallowing. On a properly positioned lateral neck radiograph, a prevertebral space of less than 6 mm at the level of C3 is normal in children.
Pseudosubluxation of C2 on C3 refers to normal physiological displacement at this level on lateral radiographs with the neck flexed. This occurs in about half of children under the age of 8 years.3 The posterior cervical line can be utilized to further evaluate this finding. This is a line drawn from the anterior aspect of the spinous process of C1 to the anterior aspect of the spinous process of C3. The anterior margins of the spinous processes of C1, C2, and C3 should align within 1 mm of each other on both flexion and extension views (Figure 22-5). Pathological subluxation of C2 on C3 is suggested if the posterior cervical line does not overlap the anterior aspect of the spinous process of C2 by 2 mm or more.
Figure 22–5
Pseudosubluxation.
There is anterior slippage of the C2 vertebral body relative to C3 on this lateral flexion image of a 3-year-old child. There is appropriate orientation of the spinous processes with the posterior cervical line. Also, note physiological prominence of the atlantodens interval and widening of the distance between the spinous processes of C1 and C2.

The vertebral column forms from chondrification and subsequent ossification of growth centers for the centra and posterior arches. Abnormal development or fusion of these growth centers and persistence of portions of the notochord are responsible for most of the major vertebral malformations. Various osseous spinal abnormalities also occur in conjunction with developmental anomalies of the meninges and spinal cord.
Developmental anomalies of the vertebrae include segmentation failure and failure of formation. The most common primary vertebral anomalies are block vertebrae, wedge vertebrae, bifid vertebrae, and spina bifida occulta. Scoliosis, kyphosis, and spinal canal encroachment are potential complications.
A block vertebra refers to fusion of 2 adjacent vertebrae, with an absent or rudimentary intervertebral disc. The height of the resulting fused vertebra is variable. There is deficient growth at the fusion site, leading to a narrow sagittal diameter and an overall concave configuration of the block vertebra (Figure 22-6). Malsegmentation of the posterior elements of a block vertebra is also common. Anomalies such as hemivertebra are often present at adjacent levels.
Figure 22–6
Block vertebra.
The C6-C7 intervertebral disc (arrow) is narrow and lacks normal central hyperintensity on this sagittal T2-weighted image of an asymptomatic 10-year old. Undergrowth of the portions of the vertebral bodies adjacent to the hypoplastic disc results in focal expansion of the CSF-filled spinal canal.

Fusion that involves only 2 vertebral segments is usually asymptomatic. The incorporation of numerous vertebrae into a single block vertebra can lead to spinal deformity and limitation of motion. Block vertebrae occur most commonly in the cervical and thoracic regions, usually in the upper thoracic and lower cervical areas. In most cases, the anomaly is isolated. However, block vertebrae are common in association with various skeletal anomaly syndromes that affect the spine, such as dysraphism and Klippel-Feil syndrome.
As viewed on lateral radiographs, the bodies of a block vertebra are variably increased in height compared to adjacent normal vertebrae. The sagittal diameter of the vertebral bodies is usually decreased, particularly at the level of fusion. Bone trabeculae are sometimes visible coursing across the fusion site (Figure 22-7). A line of condensation or a linear radio-lucency is often present at the site of the absent or rudimentary intervertebral disc (Figure 22-8). Frequently, the interspaces above and below the block vertebra are somewhat narrowed. Fusion also may involve the posterior elements, sometimes causing narrowing or obliteration of the neural foramina at the level of the fusion. Posterior element involvement is usually accompanied by zygapophyseal (facet) joint fusion.
Figure 22–8
Block vertebra.
A. A coronal reformatted CT image demonstrates congenital fusion of 2 thoracic vertebrae. There is a thin rudimentary disc. B. Fusion of the posterior elements, including the facet joint, is visible on the sagittal image. There is only mild narrowing of the neural foramen.

Klippel-Feil syndrome is the clinical triad of short neck, marked limitation of neck mobility, and a low posterior hairline. Radiographs demonstrate one or more segmentation anomalies in the cervical and upper thoracic portions of the spine. Klippel-Feil syndrome is of unknown etiology and occurs in 1 in 40,000 to 1 in 50,000 births. The mean age at the time of diagnosis is 2 to 3 years. The presumed embryonic insult likely occurs between the fourth and eighth weeks of gestation.4
There are 3 types of Klippel-Feil syndrome. Type I refers to extensive fusion of most of the cervical and upper thoracic portions of the spine. Associated anomalies and severe neurological consequences are common with this type. Type II is the most common form of Klippel-Feil syndrome, accounting for more than 80% of cases. These patients have fusions of 1 or 2 cervical interspaces; the most common sites are C2-3 and C5-6. Type III refers to fusions of the cervical spine in conjunction with fusion anomalies of the lower thoracic or lumbar regions.5
Additional anomalies of the cervical spine can be present in patients with Klippel-Feil syndrome. These include spina bifida, hemivertebra, vertebral body clefts, spinal stenosis, enlarged or obliterated neural foramina, occipital assimilation, deformity of the odontoid process, and basilar invagination. Other anomalies that sometimes occur in association with Klippel-Feil syndrome include diastematomyelia, Chiari I malformation, genitourinary anomalies (e.g., unilateral renal agenesis, ectopia or dysgenesis of the kidney, hypospadias, cryptorchidism, vaginal agenesis, and bicornuate uterus), hearing impairment (due to ear anomalies), and congenital heart disease. Neurological disabilities in these children are sometimes related to spinal stenosis or vertebral artery compression in the involved portions of the cervical spine. Rarely, there is an associated motor disorder called synkinesia, consisting of involuntary mirror motion of the hands; this apparently is due to incomplete decussation of the pyramidal tracts. Progressive scoliosis occurs in about half of children with Klippel-Feil syndrome.
In approximately 25% of patients with Klippel-Feil syndrome, there is high position of a scapula, that is, Sprengel deformity. Conversely, approximately 40% of patients who present with clinical manifestations of Sprengel deformity have radiographic evidence of Klippel-Feil syndrome. Unilateral or bilateral omovertebral bones (an anomalous bone that connects the scapula with the cervical spine) are visible on radiographs in many of these patients (Figure 22-9).6
Figure 22–9
Klippel-Feil syndrome and Sprengel deformity.
A. A lateral radiograph of an infant with torticollis, a short neck, and elevated shoulders shows multiple neural arch fusions of the cervical spine. Large omovertebral bones are present (arrow). B. CT confirms the presence of omovertebral bones (arrows) extending toward the scapulae.

Radiographs of patients with Klippel-Feil syndrome show a short neck and segmentation anomalies of the cervical spine. Most often, there are 2 or more cervical block vertebrae. In severe cases, there is extensive fusion of all or most of the cervical vertebrae (type I). Abnormal curvature of the cervical spine may also be present. Anomalies of the upper thoracic vertebrae and the upper ribs sometimes occur in association with Klippel-Feil syndrome. In infants, Klippel-Feil syndrome can be difficult to detect radiographically because the sites of fusion are mostly cartilaginous at this age. Fusion of one or more spinous processes in the cervical spine is often the earliest radiographic indicator of Klippel-Feil syndrome in infants. Subsequent evaluation as the child ages shows progression to fusion of several cervical vertebrae. Isolated fusion anomalies of the cervical spine are sometimes considered part of the Klippel-Feil spectrum; however, patients with true Klippel-Feil syndrome have one or more of the above-described clinical features (Figure 22-10).
Klippel-Feil deformity is readily demonstrated on CT and MRI. The block vertebrae appear as narrowed, fused vertebral bodies, sometimes with partial interspace formation. This is best visualized on sagittal images (Figure 22-11). MR is particularly useful for demonstrating associated abnormalities of the intracranial and intraspinal structures in these patients.
Most hemivertebrae are the result of failure of normal embryonic development of one or more chondrification or ossification centers. As described above, the vertebral centra are predominantly formed from 2 side-by-side (i.e., paramedian) chondrification centers and 2 primary ossification centers, 1 anterior and 1 posterior. In most instances, the patterns of hemivertebra formation are readily explained by alterations in these developmental processes. A hemivertebra also can result from the formation of a supernumerary ossification center.
Hemivertebrae are most common in the thoracolumbar spine. A hemivertebra may or may not have a pedicle and may or may not fuse with the adjacent vertebra; the pedicle ipsilateral to the absent ossification center is often hypoplastic or absent. Absence of a pedicle on a frontal view is an important radiographic clue to the presence of a hemivertebra. Hemivertebrae may occur at single or multiple levels. Multiple hemivertebrae can be ipsilateral or alternate. Some hemivertebrae are clinically inconsequential, while others cause progressive scoliosis. Thoracic lateral hemivertebrae are usually associated with rib anomalies, such as aplasia, hypoplasia, or fusion. The rib contralateral to a lateral hemivertebra is usually absent. A thoracic hemivertebra may result in the presence of an ipsilateral supernumerary rib.
The rare failure of development of the ventral half of the embryonic vertebral body results in a dorsal (posterior) hemivertebra; this is usually associated with progressive angular kyphosis (gibbous deformity). Posterior positioning of a dorsal hemivertebra due to underdevelopment of the neural arch can lead to spinal stenosis and impingement on the spinal cord or nerve roots, sometimes causing paraplegia, respiratory compromise, or other neurological problems (Figure 22-12).
Figure 22–12
Dorsal hemivertebra.
A. A lateral radiograph of a 3-year-old boy shows failure of development of the anterior aspect of the L3 vertebral body. There is mild angular kyphosis at this level. There is posterior positioning of the vertebral body relative to the adjacent vertebra due to a hypoplastic neural arch. B. Secondary expansion of the discs is visible on this T2-weighted MR image. The discs bulge into the ventral defect. Bulging discs adjacent to the posteriorly positioned vertebra contribute to spinal stenosis.

Lateral hemivertebrae are characterized as “balanced” or “unbalanced” according to the relationship to the adjacent vertebrae. With balanced multiple hemivertebrae, there are alternating hemivertebrae in a pattern that mitigates the deforming effects. A balanced single hemivertebra refers to ipsilateral undergrowth and contralateral overgrowth of the adjacent vertebral bodies, such that substantial scoliosis is lacking. With unbalanced hemivertebrae, clinically evident scoliosis (often angular) usually occurs (Figure 22-13). All types of hemivertebra cause some degree of spinal deformity. Clinical monitoring and serial radiographic examinations of these children are important to ensure early detection of progressive scoliosis, kyphosis, or kyphoscoliosis.
Figure 22–13
Fused hemivertebra.
A. There is an extra pedicle on the left between L2 and L3. The left side of the vertebral body is widened and there is associated levoscoliosis (i.e., an unbalanced hemivertebra). B. A sagittal reformatted CT image though the left side of the lumbar spine shows fusion of the body and posterior elements (arrow) of the hemivertebra to those of L2, resulting in a complete ring at the neural foramen.

A butterfly vertebra (double wedge vertebra) has a developmental sagittal cleft in the vertebral body. This anomaly is due to failure of fusion of the lateral chondrification centers of the vertebral centrum. In some instances, there are persistent remnants of the fetal notochord in the center of the anomalous vertebral body. Some degree of lateral displacement of the halves of the vertebral body often occurs; this may increase in severity when the child reaches the age of regular weight bearing. On frontal radiographs, each half of the vertebral body has a triangular configuration or C-shape (Figure 22-14). The pedicles are usually slightly splayed. If asymmetric, a butterfly vertebra can cause scoliosis. Anterior hypoplasia can cause angular kyphosis (Figure 22-15). Butterfly vertebra can occur as an isolated abnormality, in association with Alagille syndrome (arteriohepatic dysplasia), and in various skeletal dysplasias (e.g., Crouzon syndrome).7
Delayed fusion of the ventral and dorsal ossification centers of a vertebral body can result in a coronal vertebral body cleft. This appears as a vertically oriented linear radio-lucency in the vertebral body when viewed on the lateral projection. Most often, multiple adjacent vertebral bodies are affected. This finding can occur as a developmental variation in infants or in association with a skeletal dysplasia, such as chondrodysplasia punctata. Spontaneous resolution during the first several weeks of life is typical.
The normal vertebral column is comprised of 7 cervical, 12 thoracic, 5 lumbar, 5 sacral, and 4 coccygeal vertebrae; this pattern is present in 90% to 95% of the population. The number of cervical vertebrae is relatively constant, but there is variability in the number of thoracic or lumbar vertebrae in at least 5% of individuals. Ribs, unilateral or bilateral, are present at the first lumbar vertebra in approximately 5% to 10% of individuals. Ribs are lacking at the T12 vertebra in approximately 2% of the population (Figure 22-16). Most alterations in the number of vertebrae involve incorporation of a vertebra at the margin of a segment of the spine (junctional vertebra) into a higher or lower segment; an actual deviation from the normal total number of vertebrae is rare. The most accurate method to assign appropriate designations of vertebrae is to count from the occiput.8,9
Numeric variability of vertebrae is most frequent in the sacrococcygeal region. A common anomaly in this portion of the spine is the addition of 1 vertebra to the sacrum and the loss of 1 vertebra from the coccyx. Variation in the lumbosacral and thoracolumbar regions is also common. This usually occurs as either an increase or a decrease in the number of lumbar vertebrae, accompanied by a corresponding increase or decrease in the number of sacral (most common) or thoracic vertebrae.
A transitional vertebra is a junctional vertebra that has characteristics common to both the vertebral segment above and the segment below. This anomaly most often occurs at the lumbosacral junction. A transitional vertebra is usually of no clinical significance. The extreme forms of transitional vertebrae are sacralization of L5 and lumbarization of Sl. In the normal spine, vertebra 24 is the last lumbar vertebra (L5) and vertebra 25 is the first sacral vertebra (S1).
Sacralization refers to developmental alteration in vertebra 24 (the presacral vertebra) such that it has the characteristics of a sacral vertebra; this occurs in approximately 6% of the population. The radiographic features of sacralization include unilateral or bilateral enlargement of the transverse processes and partial or complete fusion of a transverse process or vertebral body of the presacral vertebra with the sacrum. Sacralization results in the presence of only 4 vertebrae with lumbar characteristics. The disc space between the presacral vertebra and the first sacral vertebra is narrow (Figure 22-17).
Lumbarization refers to developmental morphology of vertebra 25 (normally S1) such that it has the characteristics of a lumbar vertebra. With lumbarization, there is unilateral or bilateral lack of formation of the lateral masses of the first sacral vertebra. The vertebral body may either be assimilated or become the last presacral vertebra. Lumbarization is less common than sacralization; the prevalence is approximately 2%.
Some transitional vertebrae at the lumbosacral junction have features intermediate between those of a lumbar vertebra and a sacral vertebra. One or both transverse processes are prominent and articulate with or fuse to the sacrum or the iliac bones. The body of the transitional vertebra is usually square and is separated from the next most inferior vertebral body by a rudimentary disc.10,11
Accurate numbering of lumbar vertebra is sometimes difficult on MR or CT examinations that do not include the cervical and thoracic segments of the spine. The 2 most reliable features for numbering are the inferior-most ribs and the iliolumbar ligaments. The last ribs are at T12 in approximately 97% of individuals. The iliolumbar ligaments are at L5 in 98%. On MR, the iliolumbar ligament appears as a single or double band that extends from the transverse process of L5 to the posteromedial aspect of the iliac crest. Other useful, but more variable, landmarks are the right renal artery (L1-2 disc), celiac trunk (L1), aortic bifurcation (L5), and the longest lumbar transverse process (L3).12
Limbus vertebra is a developmental variation that appears radiographically as a small osseous density adjacent to the endplate of one or more vertebral end plates. The pathogenesis involves protrusion of a portion of the intervertebral disc into the vertebral body between the cartilaginous endplate of the ring epiphysis and the border of the adjacent vertebral rim. Limbus vertebra is asymptomatic; there is no association with spinal deformity or growth disturbance. The major implication is the potential for confusion with a destructive lesion or an acute fracture.13
The radiographic appearance of limbus vertebra is that of a triangular osseous density adjacent to a vertebral body endplate. Both the limbus vertebra and the adjacent vertebral body have sclerotic margins; this finding aids in the differentiation from an acute fracture. Limbus vertebra most frequently occurs along the anterosuperior aspects of the mid-lumbar vertebral bodies; however, lateral, posterior, and inferior locations sometimes occur. In young individuals, the annular epiphyses produce similar radiographic findings, typically at multiple levels.14
Each neural arch ossifies from 2 primary centers that normally fuse during the first through third years of life. Fusion begins in the lumbar region and progresses to the cervical region. The radiographic appearance of a neural arch fusion defect can be due to a true bony gap or an apparent defect that consists of unossified cartilage. An isolated failure of bony fusion of the neural arches of a developing vertebra is termed spina bifida occulta (Figure 22-18). This clinically insignificant developmental variation occurs in up to 9% of females and 1% of males. The most common sites of spina bifida occulta are L5, S1, C1, C7, and T1.
In addition to a midline cleft, various other neural arch ossification defects can occur. These include any combination of defects of the laminae, pedicles, and spinous processes. Although the spinous process often remains unossified if there are bilateral defects in the laminae, there are instances in which the ossification center of the spinous process fuses unilaterally with a single lamina or joins the spinous process of the next higher or lower vertebra. Bilateral congenital defects in the laminae can result in spondylolisthesis.
Congenital absence of a pedicle is a rare anomaly that most often occurs in the lumbar spine or, less frequently, the cervical spine. This anomaly is extremely rare in the thoracic area. Unilateral congenital absence of a pedicle does not usually cause significant symptoms. Bilateral pedicle aplasia, however, may be associated with clinically apparent spinal instability. Congenital absence of the pedicle is apparently a defect in cartilaginous development. The chondrification center that develops in each side of the neural arch gives rise to a pedicle, the superior and inferior articular processes, the transverse process, and the lamina with its spinous process. Consequently, congenital absence of the pedicle is usually associated with other anomalies of the neural arch and vertebral appendages. Both absence and severe hypoplasia of the pedicle can occur in patients with neurofibromatosis type 1 (NF-1); this is a manifestation of mesodermal dysplasia. Deficiencies in lumbar vertebral pedicles occasionally occur in association with renal anomalies, such as unilateral renal aplasia.15–17
Unilateral congenital absence of a pedicle typically leads to stress changes in the contralateral pedicle and neural arch, that is, hypertrophy, with or without sclerosis. This is a useful radiographic finding that helps to distinguish congenital absence of the pedicle from acquired destruction (e.g., neoplasm or trauma). The severity of these secondary stress changes increases with patient age. Some degree of underdevelopment of the ipsilateral portion of the neural arch, particularly the superior facet, usually accompanies congenital absence of a pedicle. The spinous process is usually misshapen, with or without hypoplasia. The ipsilateral transverse process is hypoplastic and is usually abnormally angulated (up or down); this is best visualized on the lateral projection. Absence of a pedicle typically is associated with enlargement of the ipsilateral intervertebral foramen. Absence of the pedicle can result in a photopenic region on bone scintigraphy. CT and MR readily demonstrate absence of a pedicle, the ipsilateral vertebral deformities, and the contralateral stress alterations.
The most common anomaly of the transverse processes is the occurrence of unilateral or bilateral supernumerary ribs at C7 (cervical rib) (Figure 22-19). This anomaly occurs in approximately 0.5% of otherwise normal individuals. Four varieties of cervical ribs are recognized: (1) a complete rib that articulates anteriorly with the manubrium, (2) an incomplete rib with a bulbous free anterior end, (3) a rib that consists partly of bone and partly of fibrous tissue, and (4) a fibrous branch that is not visible on standard radiographs. The anomaly is unilateral in about half of individuals with a cervical rib.
Most cervical ribs are asymptomatic. Rarely, clinical manifestations result from compression of adjacent nerves or vessels. The subclavian artery and brachial plexus pass through a narrow triangle that is bounded anteriorly by the scalenus anterior muscle, posteriorly by the scalenus medius muscle, and inferiorly by the first rib. Elevation of the inferior border of the triangle as a result of the inter-position of a cervical rib occasionally causes compression of the subclavian artery and the first thoracic nerve. This can lead to neck pain, manifestations of upper extremity hypoperfusion, or paralysis in the distribution of the first thoracic or lower cervical nerves (i.e., thoracic outlet or scalene syndrome). MR or contrast-enhanced CT is useful for demonstrating the osseous and soft tissue anatomy adjacent to a cervical rib in symptomatic patients.
Anomalies at the craniocervical junction range from asymptomatic developmental variations to lesions that cause cord compression and neurological compromise. The included structures are the basiocciput, atlas, and axis. The pathological spectrum includes aplasia/hypoplasia, focal ossification defects, segmentation anomalies, and fusion/assimilation (Table 22-1). Potential signs and symptoms of craniocervical junction pathology include motor myelopathy, sensory abnormalities, and brainstem dysfunction (e.g., nystagmus, ataxia, apnea)18,19
Anomalies of the craniocervical region | ||
---|---|---|
Occipital bone malformations | Occipital vertebra | |
Basilar invagination | ||
Condylar hypoplasia | ||
Assimilation of the atlas | ||
Basiocciput hypoplasia (short clivus) | ||
Atlas malformations | Assimilation of the atlas | |
Atlantoaxial fusion | ||
Atlas arch aplasia | ||
Axis malformations | Segmentation anomalies | |
Dysplasia of the dens | Os odontoideum | |
Aplasia/hypoplasia | ||
Ossiculum terminale persistens | ||
Bifid dens |
Ponticulus posticus, or arcuate foramen, is the most common anomaly of the atlas, occurring in up to 10% to 15% of the population. This is a bony bridge along the superior aspect of the groove in the neural arch for the vertebral artery (the vertebral artery sulcus). This ossification occurs in the atlantooccipital ligament. Ossification of the ligament can be partial or complete and unilateral or bilateral. Although generally considered to be a common normal variation, ponticulus posticus is often present in children who suffer vertebral artery dissection. Ponticulus posticus is visible on lateral cervical spine radiographs as a thin bony arch along the superior aspect of the posterior ring of C1 (Figure 22-20). The vertebral artery and suboccipital nerve pass through this ring.20
Basilar invagination refers to superior displacement of the margins of the foramen magnum into the posterior fossa of the skull and superior prolapse of the first cervical vertebra and odontoid process. This deformity occurs in congenital and acquired forms. Congenital basilar invagination can occur as an isolated anomaly or in association with a developmental abnormality such as occipitalization of the atlas, hypoplasia of the atlas, Klippel-Feil syndrome, achondroplasia, or cleidocranial dysplasia. The acquired variety of basilar invagination is more properly termed basilar impression. This is much less common than the developmental form. Potential underlying conditions include rickets, osteogenesis imperfecta, hypothyroidism, rheumatoid arthritis, Paget disease, vertebral neoplasm, and vertebral osteomyelitis.
Most patients with basilar invagination are asymptomatic. However, severe deformity leads to protrusion of the odontoid process into the foramen magnum, with the potential for encroachment on the brainstem or basilar artery. Neurological symptoms in patients with basilar invagination usually are nonspecific. Potential findings include cervical or occipital pain, cranial nerve palsy (due to compression of the upper cervical cord or brainstem), and manifestations of syringomyelia or syringobulbia.
The diagnosis of basilar invagination is usually made by conventional radiography. CT provides a more accurate depiction of the pathologic osseous anatomy, however. MR is frequently helpful in these patients to determine the relationship of the bony abnormality to adjacent neural structures (Figure 22-21). Potential central nervous system (CNS) lesions in these patients include syringomyelia, hydrocephalus, and compression of the cord or brainstem.
Figure 22–21
Basilar invagination.
A. A prominent odontoid process prolapses through the foramen magnum of this 6-year-old boy, extending superior to the Chamberlain line. The posterior margin of the clivus is blunt. There is deficient ossification of the atlas. B. There is cord compression between the odontoid and posterior arch of the hypoplastic atlas. The cartilaginous C1 arch is hypointense on this T2-weighted MR image. There is signal alteration in the compressed segment of cord due to gliosis or edema.

A number of radiological measurements have been devised to aid in the diagnosis and characterization of basilar invagination. These include the Chamberlain line, McGregor line, Bull angle, and McRae line. The Chamberlain line extends from the dorsal margin of the hard palate to the dorsal lip of the foramen magnum as viewed on a true lateral skull radiograph (or sagittal CT or MR image). Projection of the odontoid process 6.6 mm or more above this line is the 2-standard deviation (SD) value for this measurement. The McGregor line is drawn from the superior surface of the posterior aspect of the hard palate to the most caudal plane of the occipital curve of the occipital bone as viewed on a lateral skull radiograph. This line is useful when the opisthion is not visible. The tip of the odontoid should project no more than 5 mm above this line. Normal values for this measurement according to patient age are available. The Bull angle is measured between the Chamberlain line and a line connecting the central plane of the anterior and posterior portions of the atlas. An angle of greater than 13° indicates the presence of basilar invagination. The McRae line is drawn from the anterior lip of the foramen magnum (the basion) to the posterior lip of the foramen magnum (the opisthion) on a lateral radiograph of the skull or midline sagittal CT/MR. This line measures the anteroposterior dimension of the foramen magnum. Normally, the odontoid tip is below or only slightly above this line.21–25
The usefulness of the Bull angle method for the diagnosis of basilar invagination is limited because of its variation with flexion and extension of the neck and head. A major shortcoming of the Chamberlain line and McGregor line methods for the determination of basilar invagination is that these lines are drawn from the hard palate, a structure that is not directly related to the anatomy of the skull base. The position and plane of the hard palate are somewhat variable and are altered by developmental alterations in the facial bones. A low palate may give the false impression of basilar invagination, whereas a high palate may cause the correct diagnosis to be missed by these methods. Further complicating the diagnosis of this anomaly is the fact that the odontoid process can extend to a high position without true basilar invagination. This may occur with a congenitally elongated odontoid or with occipitalization of the atlas.
Platybasia is an anthropological term that refers to an abnormal obtuseness of the basal angle of the skull (i.e., flattening of the skull base). Although basilar invagination and platybasia may coexist, the 2 terms are not synonymous. The basal angle of the skull is the angle between a line drawn from the nasion to the center of the pituitary fossa and the line from the center of the pituitary fossa to the anterior rim of the foramen magnum. The normal basal angle measures between 103° and 131°. Platybasia exists if the basal angle is equal to or exceeds 145°.26
Occipitalization of the atlas (atlanto-occipital nonsegmentation) refers to partial or complete fusion of the atlas (C1) with the occipital bone. If the fusion is complete, this is termed assimilation of the atlas. Occipitalization of the atlas can be an isolated and asymptomatic anomaly, but it is most often associated with other craniovertebral anomalies, such as atlantoaxial subluxation, cervical vertebral fusions, basal invagination, herniation of the cerebellar tonsils, and dural bands. These associated anomalies can lead to clinical manifestations of spinal cord compression. Associated fusion of C2-3 occurs in approximately 70% of patients with occipitalization of the atlas.27
Fusion of the atlas with the occiput may occur anteriorly, posteriorly, or both. Typically, imaging studies show fusion of the anterior arch of the atlas with the anterior lip of the foramen magnum (Figure 22-22). When present, a fused posterior arch is usually visible as a bony ridge at the posterior margin of the foramen magnum. The lateral masses of the atlas may fuse with the occiput either unilaterally or bilaterally; this most often occurs asymmetrically. With complete occipitalization, or assimilation, of the atlas, all components of the atlas are fused to the base of the skull.
Occipitalization of the atlas is often difficult to detect radiographically in infants and young children. The anterior arch of C1 is unossified at birth in approximately 80% of newborns, and a portion of the posterior arch of C1 is normally unossified at this age as well. In older children, standard radiographs are usually diagnostic. However, CT or MR is usually indicated to better define the pathological anatomy and to detect CNS involvement.
The atlas develops from 3 primary ossification centers: 1 for the anterior arch and 1 for each of the 2 lateral masses. The posterior elements ossify from the posterior portions of the lateral ossification centers. The 2 sides of the posterior arch usually unite between years 3 and 5, either directly or with an interposed separate ossicle. Occasionally, fusion does not occur until 10 to 12 years of age. Failure of complete midline osseous fusion of the posterior arch of the atlas occurs in approximately 3% of the general population. This anomaly is entirely asymptomatic. Failure of fusion of the posterior elements of the atlas is usually best visualized on frontal or oblique radiographs but may also be detectable on the lateral view. The laminae usually have a rounded appearance near the midline and do not meet. Occasionally, a separate ossicle is visible at the midline between the separated laminae.28
Failure of posterior midline fusion of the hemiarches accounts for at least 90% of posterior arch defects of the atlas. Additional potential ossification defects include a unilateral arch defect, bilateral arch defects, absence of the posterior arch(es) with preservation of the posterior tubercle, and absence of the posterior arches in conjunction with absence of the posterior tubercle (Figure 22-23).29
There are 2 types of anomalies of ossification of the anterior arch of the atlas: accessory ossicles and ossification defects. At birth, the anterior arch of the atlas consists of a ring of fibrocartilage that undergoes ossification near the end of the first year, and osseous fusion with the neural arch by approximately the age of 7 years. There are 2 contiguous nuclei in the anterior arch that fuse to form a single ossification center. Occasionally, an extra inferior ossification center persists to form an accessory ossicle of variable size. An ossification defect of the anterior arch of the atlas is an unusual anomaly; radiographs show absence of the bony ring of C1 anterior to the dens.
Asymmetry of the lateral masses of the atlas is an unusual but clinically significant anomaly. Torticollis or limitation of neck motion is the clinical consequence of this developmental lesion. There is usually partial compensation for the deformity by reverse asymmetry of the lateral masses of the axis.
Hypoplasia of the neural arch of C1 is an uncommon congenital anomaly that can cause symptomatic cervical spinal stenosis. Most often, the anomalous small arch is completely ossified, has a curved configuration, and is smooth. Occasionally, there is an abnormal straight or incurved shape. There is failure of midline ossification in some patients with atlas hypoplasia. Radiography and CT show an abnormal anterior location of the C1 arch, despite appropriate alignment of the atlantoaxial joint. MR demonstrates the effects on the thecal sac and cord (Figure 22-21) (Figure 22-24).30
Figure 22–24
Atlas hypoplasia.
A. A sagittal midline CT image of a 3-year-old child shows an abnormal anterior position of the C1 neural arch (arrow). B. An abnormally straightened configuration of the arch is visible on this axial image at the C1 level. C. A sagittal T2-weighted MR image confirms localized cervical spinal stenosis and slight impression on the dorsal aspect of the cord.


The occipital vertebra, or proatlas, is the most cephalad of the embryonic primitive vertebrae. Normally, it fuses with other primitive vertebrae to form the basilar process of the occipital bone. Failure of normal fusion leads to the development of a small area of abnormal bone along the outer surface of the skull adjacent to the anterior portion of the foramen magnum. It may be an isolated entity or occur in combination with other craniocervical anomalies.
The most frequent radiographic appearance of an occipital vertebra is the so-called third condyle; this is a midline bony structure that is adjacent to, but distinct from, the anterior portion of the foramen magnum. This “condyle” may be a single tuberosity with an anterior articular facet or an articular depression in the foramen magnum. With standard radiographs, an occipital vertebra is best visualized on the lateral view.
Iniencephaly is a rare, usually fatal, axial dysraphic malformation complex characterized by spina bifida of the cervical vertebrae, fixed retroflexion of the head on the cervical spine, and an occipital bone defect. One or more CNS malformations are present in these infants, such as anencephaly, posterior encephalocele, or polymicrogyria. Other reported associated malformations include diaphragmatic hernia, omphalocele, anomalies of the GI, cardiovascular, or genitourinary systems, facial cleft, spina bifida, hypoplastic lungs, clubfoot, and single umbilical artery. The diagnosis is frequently established with prenatal sonography. The pathological bony anatomy in a surviving infant is optimally demonstrated with 3D CT, whereas the neurological abnormalities can be documented with MR.31–34
Congenital anomalies of the odontoid process include aplasia, hypoplasia, and anomalies of fusion. Ossification anomalies of the dens are clinically important because they can simulate traumatic or other pathologic abnormalities on radiographs. The most common anomalies are the ossiculum terminale, os odontoideum, and hypoplasia of the dens. Congenital absence of the odontoid process is rare.
Os odontoideum refers to the appearance of the dens as a separate ossicle. This is most often due to congenital failure of osseous fusion between the odontoid process and the body of the axis. Other individuals have a fusion defect in the odontoid itself. The odontoid process is cartilaginous during the neonatal period; ossification does not occur until later in infancy and childhood. The majority of the odontoid process is ossified by 2 to 3 years of age, although there is a persistent V-shaped vertical cleft at its tip. The synchondrosis between the dens and the body of the axis begins to ossify between the ages of 3 and 6 years, but may remain at least partially apparent radiographically until approximately 11 years of age. This normal synchondrosis is visible radiographically in all young children as a lucent line that separates the odontoid process from the main body of the axis. In most patients with os odontoideum, the cartilaginous synchondrosis is intact despite lack of osseous fusion; therefore, the atlantoaxial joint is stable and no symptoms occur. Integrity of the transverse ligament is also important in maintaining alignment.35–37
Radiographs and CT of a classic os odontoideum show a radiolucent gap between the odontoid process and the body of the axis. There is usually a plate-like sclerotic depression in the superior facet of the axis. The osseous margins of the subdental synchondrosis are smooth and corticated. These findings help in the differentiation from an acute fracture. In some instances, the os odontoideum has the configuration of a small oval or round ossicle that is separated from, and cephalad to, a hypoplastic odontoid process that is appropriately fused to the body of the axis. The ossicle sometimes occupies the normal position at the tip of the odontoid process. Frequently, however, there is abnormal superior migration of the os odontoideum such that it is near the basion, either separate from or fused to it (Figure 22-25). This type of os odontoideum is often unstable.
Figure 22–25
Os odontoideum.
A, B. Sagittal and coronal reformatted CT images show a gap between the superior and mid portions of the dens (arrows). The bony margins are smooth and corticated. The superior tip of the os extends closer to the basion than is typical of a normal dens. The main portion of the dens is somewhat malformed. There is no significant anterior-posterior malalignment.

Some instances of os odontoideum apparently represent the sequela of an unrecognized fracture of the dens during infancy. This can be followed by nonunion, vascular compromise of the bone above the fracture, and partial or complete failure of further development of this avascularized segment. In these cases, the dens is small due to bone resorption and diminished growth. In addition, the malformed dens is usually separated from the main body of C2 by a larger radiolucent gap than is typical for congenital os odontoideum. The atlantoaxial joint is often unstable in these children, and there is a risk of neurological complications.
Lateral flexion and extension views or fluoroscopic evaluation are useful for selected patients with os odontoideum to detect atlantoaxial instability (Figure 22-26). There is considerable individual variability in the presence or absence of, and the severity of, motion. There is a greater tendency for pathological atlantoaxial motion in patients with the acquired form. Most patients with congenital os odontoideum have only slight motion at the subdental synchondrosis, with the odontoid moving in concert with the ring of C1. Most often, this motion is less than 3 mm, but greater degrees of motion sometimes occur in patients despite a lack of symptoms. Substantial atlantoaxial motion or fixed malalignment can lead to neck pain or symptoms of cervical spinal cord impingement. MR evaluation is indicated for symptomatic patients to evaluate the cord. CT is helpful to optimally define the osseous anatomy (Figure 22-27).
Figure 22–26
Os odontoideum.
A. An os odontoideum appears on this lateral flexion view as a corticated ossicle (arrow) posterior and superior to the anterior arch of C1. The os impinges upon the basion. There is minimal anterior motion relative to the body of C2. B. There is posterior displacement of C1 and the contiguous os odontoideum (arrow) on this extension view, such that the anterior arch of C1 is perched above the body of C2.

Figure 22–27
Os odontoideum.
A sagittal CT image of a 14-year-old patient with neck pain shows an os odontoideum as a well-corticated ossicle (arrow) that articulates with the anterior arch of C1. The ossicle extends superiorly to approach the basion. There is a large gap between the ossicle and the slightly malformed and retroflexed base of the odontoid. The anteriorly displaced atlas causes marked spinal canal narrowing.

Ossiculum terminale (os terminale) is a common, clinically insignificant, developmental variation of the odontoid process. In infants and young children, there is a V-shaped cleft at the tip of the ossified portion of the odontoid. A separate ossification center within this cleft is visible radiographically in about one-quarter of children between the ages of 5 and 11 years (Figure 22-28). This ossification center usually fuses with the tip of the odontoid process by the age of 12 years. Failure of fusion results in a small persistent ossicle at the tip of the dens, that is the ossiculum terminale persistens (Figure 22-29). This diagnosis should not be made based on the radiographic appearance in children younger than 12 years, as normal fusion of the structures does not occur until this age.
Segmental spinal dysgenesis is a rare complex anomaly in which an isolated portion of the thoracic or lumbar vertebral column and the adjacent portion of the spinal cord are either aplastic or markedly hypoplastic (Figure 22-30). Patients with segmental spinal dysgenesis have spinal deformity, paraplegia or paraparesis, and lower limb deformities. Gibbus deformity is present at the site of the lesion. The spinal canal is focally stenotic. When the defect is in the thoracic portion of the spine, the ribs at the level of the lesion are absent.38
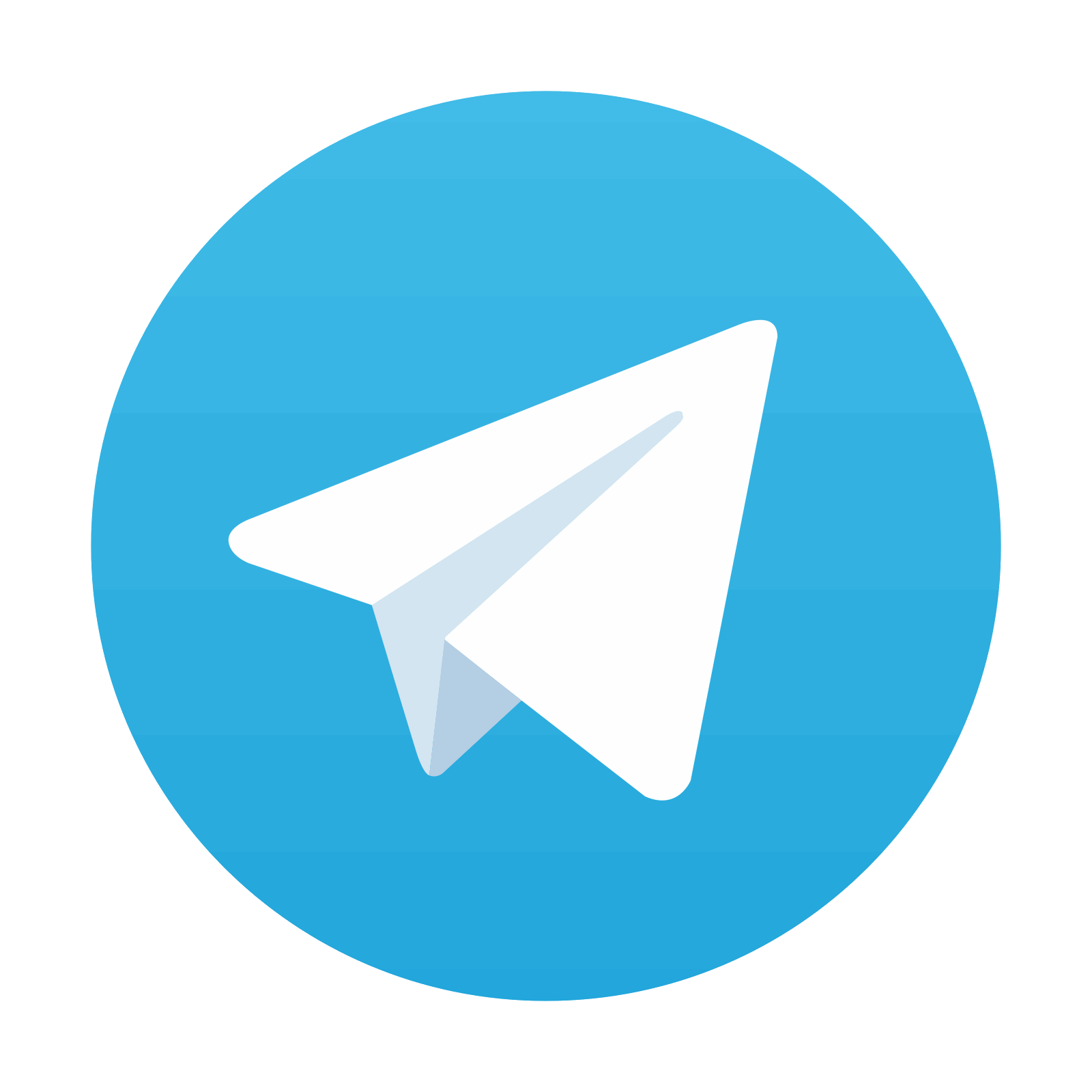
Stay updated, free articles. Join our Telegram channel

Full access? Get Clinical Tree
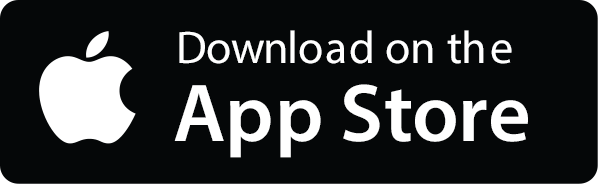
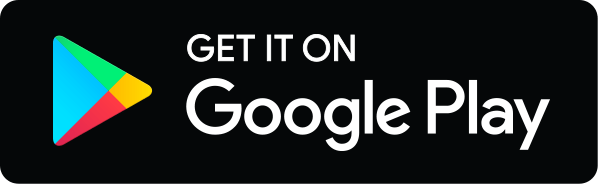