Development of Eyes and Related Structures
17
The eyes begin to develop in 22-day embryos when optic grooves appear ( Fig. 18.1 A and B ). The eyes are derived from four sources:
- •
Neuroectoderm of the forebrain
- •
Surface ectoderm of the head
- •
Mesoderm between the previous two layers
- •
Neural crest cells

The neuroectoderm differentiates into the retina, posterior layers of iris, and optic nerve. The surface ectoderm forms the lens of the eye, sclera, and corneal epithelium. The mesoderm between the neuroectoderm and surface ectoderm gives rise to the fibrous and vascular coats of the eye. Three waves of neural crest cells from the prosencephalon and mesencephalon migrate into the mesenchyme and differentiate into the portion of the corneal endothelium and stroma of the cornea, ciliary body, ciliary muscles, and trabecular network.
Early eye development results from a series of inductive signals. Homeobox-containing genes, including the transcription regulator PAX6, fibroblast growth factors, and other inducing factors, such as the gene PITX2 , play important roles in the molecular development of the eye .
The first evidence of eye development is the appearance of optic grooves in the neural folds at the cranial end of the embryo (see Fig. 18.1 A and B ). As the neural folds fuse to form the forebrain, the optic grooves evaginated (protruded) from the future diencephalon to form hollow diverticula (outpocketings) called optic vesicles , which project from the wall of the forebrain into the adjacent mesenchyme. The vesicles soon come in contact with the surface ectoderm ( Fig. 18.1 C and D ). The cavities of the optic vesicles are continuous with the cavity of the forebrain. Formation of the optic vesicles is induced by the mesenchyme adjacent to the developing brain.
As the optic vesicles grow, their distal ends expand, and their connections with the forebrain constrict to form hollow optic stalks (see Fig. 18.1 D ). Concurrently, the surface ectoderm adjacent to the vesicles thickens to form lens placodes , which are the primordia of the lenses (see Fig. 18.1 C and D ). Formation of placodes in a precursor field (preplacodal region) is induced by the optic vesicles after the surface ectoderm has been conditioned by the underlying mesenchyme. An inductive message passes from the vesicles, stimulating the surface ectodermal cells to form the primordia of the lens . The lens placodes invaginate as they sink deep to the surface ectoderm, forming lens pits ( Fig. 18.2 ; see 18-1 D ). The edges of the lens pits approach each other and fuse to form spherical lens vesicles (see Figs. 18.1 F and H ), which gradually lose their connection with the surface ectoderm.

As the lens vesicles are developing, the optic vesicles invaginate to form double-walled optic cups , which consist of two layers that are connected to the developing brain by optic stalks (see Figs. 18.1 E and F and 18.2 ). The optic cup becomes the retina, and the optic stalk becomes the optic nerve. The lens and part of the cornea develop from the ectoderm and mesoderm. The opening of each optic cup is large at first, but the rim of the cup infolds around the lens ( Fig. 18.3 A ). By this time, the lens vesicles have lost their connection with the surface ectoderm and have entered the cavities of the optic cups ( Fig. 18.4 ).


Linear grooves (retinal fissures) develop on the ventral surface of the optic cups and along the optic stalks (see Figs. 18.1 E to H and 18.3 A to D ). The center of the optic cup, where the retinal fissure is deepest, forms the optic disc , where the neural retina is continuous with the optic stalk (see Figs. 18.2 and 18.3 C and D ). The developing axons of the ganglion cells pass directly into the optic stalk and convert it into the optic nerve (see Fig. 18.3 B and C ). Myelination of nerve fibers begins during the latter part of fetal development and during the first postnatal year.
The retinal fissures contain vascular mesenchyme from which hyaloid blood vessels develop (see Fig. 18.3 C and D ). The hyaloid artery , a branch of the ophthalmic artery, supplies the inner layer of the optic cup, the lens vesicle, and the mesenchyme in the cavity of the optic cup (see Figs. 18.1 H and 18.3 C ). The hyaloid vein returns blood from these structures. As the edges of the retinal fissure fuse, the hyaloid vessels are enclosed within the primordial optic nerve (see Fig. 18.3 C to F ). Distal parts of the hyaloid vessels eventually degenerate, but proximal parts of them persist as the central artery and vein of retina (see Fig. 18.3 E and 18.8 D ). Bone morphogenic protein (BMP), sonic hedgehog (SHH), and fibroblast growth factor (FGF) are essential for signaling of the optic vesicle and closure of the retinal fissure.
Retina
The retina develops from the walls of the optic cup, an outgrowth of the forebrain (see Figs. 18.1 C to F and 18.2 ). The walls of the cup develop into the two layers of the retina: the outer, thin layer of the cup becomes the pigment layer of the retina , and the inner, thick (neural) layer differentiates into the neural retina (see Figs. 18.1 H , 18.4, and 18.8 A ). The proliferation and differentiation of retinal precursor cells are regulated by forkhead transcription factors. Lhx2, Six2, Pax6, and Rax are eyelid-specific transcription factors involved in retinal neurogenesis. By the sixth week, melanin appears in the retinal pigment epithelium (see Fig. 18.8 A ).
During the embryonic and early fetal periods, the two layers of the retina are separated by an intraretinal space (see Figs. 18.4 and 18.8 A and B ), which is derived from the cavity of the optic cup. This space gradually disappears as the two layers of the retina fuse (see Figs. 18.7 and 18.8 D ), but the fusion is not firm. Because the optic cup is an outgrowth of the forebrain, the layers of the optic cup are continuous with the wall of the brain (see Fig. 18.1 H ).
Under the influence of the developing lens, the inner layer of the optic cup proliferates to form a thick neuroepithelium (see Figs. 18.2 and 18.4 ). Subsequently, the cells of this layer differentiate into the neural retina, the light-sensitive region of the retina. This region contains photoreceptors (rods and cones) and the cell bodies of neurons (e.g., bipolar cells, ganglion cells). FGF signaling regulates retinal ganglion cell differentiation.
Because the optic vesicle invaginates as it forms the optic cup, the neural retina is inverted; light-sensitive parts of the photoreceptor cells are adjacent to the outer retinal pigment epithelium. As a result, light traverses the thickest part of the retina before reaching the photoreceptors. However, because the neural retina is transparent, it does not form a barrier to light. The axons of ganglion cells in the superficial layer of the neural retina grow proximally in the wall of the optic stalk (see Figs. 18.3 B to D and 18.4 ). As a result, the cavity of the optic stalk is gradually obliterated as the axons of the many ganglion cells form the optic nerve (see Fig. 18.3 E and F ).
The optic nerve is surrounded by three sheaths that evaginate with the optic vesicle and stalk. Consequently, they are continuous with the meninges of the brain (see Fig. 18.3 F ).
- •
The outer dural sheath from the dura mater is thick and fibrous and blends with the sclera.
- •
The intermediate sheath from the arachnoid mater is thin.
- •
The inner sheath from the pia mater is vascular and closely invests the optic nerve and central arterial and venous vessels of the retina as far as the optic disc.
Cerebrospinal fluid is found in the subarachnoid space between the intermediate and inner sheaths of the optic nerve.
Myelination of the axons within the optic nerves begins in the late fetal period. After the eyes have been exposed to light for approximately 10 weeks, myelination is complete, but the process normally stops short of the optic disc, where the optic nerves leave the eyeballs. By 26 weeks, fetuses blink in response to bright light. Color perception begins by approximately week 34. Normal neonates can see but not too well because they are farsighted and can focus only to about 25 cm. They respond to changes in illumination and are able to fixate points of contrast. Visual acuity improves rapidly over the first year of infancy to almost normal adult levels.
Coloboma
Coloboma results when there is incomplete closure of the retinal fissure, creating a gap in the eye structure. These defects can occur in any ocular structure from the cornea to the optic nerve. The eyelid can develop such a defect, but it is caused by other mechanisms (see p. 405 ). Retinochoroidal coloboma is characterized by a localized gap in the retina, usually inferior to the optic disc. The defect is bilateral in most cases.
Coloboma of the iris is a defect in the inferior sector of the iris or a notch in the pupillary margin, giving the pupil a keyhole appearance ( Fig. 18.5 ). The defect may be limited to the iris, or it may extend deeper and involve the ciliary body and retina. The coloboma may be caused by environmental factors, but a simple coloboma often is hereditary and is transmitted as an autosomal dominant characteristic.
Detachment of Retina
Retinal detachment occurs when the inner and outer layers of the optic cup fail to fuse during the fetal period to form the retina and obliterate the intraretinal space (see Figs. 18.3 and 18.8 A and B ). It occurs in conjunction with Down syndrome and Marfan syndrome (connective tissue multisystemic disorder). The separation of the neural and pigment layers of the retina may be partial or complete. Retinal detachment may result from unequal rates of growth of the two retinal layers; as a result, the layers of the optic cup are not in perfect apposition. Sometimes, the layers of the optic cup appear to have fused and separate later; secondary detachments usually are associated with other defects of the eye and head trauma.
When there is a detached retina, it is not a detachment of the entire retina; the retinal pigment layer remains firmly attached to the choroid (vascular layer of eyeball) (see Fig. 18.8 D ). The detachment is at the site of adherence of the outer and inner layers of the optic cup. Although detached from the pigment layer of the retina, the neural retina retains its blood supply (central artery of retina) , which is derived from the embryonic hyaloid artery (see Fig. 18.8 A and D ).
Postnatally, the pigment layer normally becomes fixed to the choroid; however, because its attachment to the neural retina is not firm, a detached retina may follow a blow to the eye or occur spontaneously. Fluid accumulates between the pigment and neural layers, and vision is impaired.
Cyclopia
Cyclopia is a rare defect. The eyes are partially or completely fused, forming a single median eye enclosed in a single orbit ( Fig. 18.6 ). There is usually a tubular nose (proboscis) superior to the eye. Cyclopia and synophthalmia (fusion of the eyes) represent a spectrum of ocular defects. These severe defects are associated with other craniocerebral defects that are incompatible with life. Cyclopia appears to result from severe suppression of midline cerebral structures ( holoprosencephaly ; see Chapter 17 , Fig. 17.40 ) that develop from the cranial part of the neural plate. Cyclopia is a hereditary condition transmitted by a recessive trait.
Microphthalmia
Congenital microphthalmia is a heterogeneous group of eye defects. The eye may be very small and associated with other ocular defects, such as a facial cleft (see Chapter 9 , Fig. 9.44 A ) and trisomy 13 (see Chapter 20 , Fig. 20.8 and Table 20.1 ), or it may be normal-appearing. The affected side of the face is underdeveloped, and the orbit is small.
Severe microphthalmia results from arrested development of the eye before or shortly after the optic vesicles have formed in the fourth week. The eye is essentially underdeveloped, and the lens does not form. If the interference with development occurs before the retinal fissure closes in the sixth week, the eye is larger, but the microphthalmos is associated with gross ocular defects. When eye development is arrested in the eighth week or during the early fetal period, simple microphthalmos results (small eye with minor ocular abnormalities). Some cases of microphthalmos are inherited. The hereditary pattern may be autosomal dominant, autosomal recessive, or X-linked. Most cases of simple microphthalmia are caused by infectious agents (e.g., rubella virus, Toxoplasma gondii , herpes simplex virus) that cross the placental membrane during the late embryonic and early fetal periods (see Chapter 20 , Table 20.6 ).
Anophthalmia
Unilateral or bilateral anophthalmia denotes the absence of the eye, which is rare. The eyelids form, but no eyeball develops. Because formation of the orbit relies on stimulation from the developing eye, orbital defects are always present. This severe defect is usually accompanied by other severe craniocerebral defects. In primary anophthalmos , eye development is arrested early in the fourth week and results from failure of the optic vesicle to form. In secondary anophthalmos , development of the forebrain is suppressed, and absence of the eye or eyes is one of several associated defects.


Ciliary Body
The ciliary body is a wedge-shaped extension of the choroid (see Fig. 18.4 ). Its medial surface projects toward the lens, forming ciliary processes (see Fig. 18.8 C and D ). The pigmented portion of the ciliary epithelium is derived from the outer layer of the optic cup, which is continuous with the pigment layer of the retina ( Figs. 18.7 and 18.8 D ). The nonvisual retina is the nonpigmented ciliary epithelium , which represents the anterior prolongation of the neural retina in which no neural elements develop ( Fig. 18.9 ).



The ciliary muscle (smooth muscle of the ciliary body) is responsible for focusing the lens. The connective tissue in the ciliary body develops from mesenchyme located at the edge of the optic cup in the region between the anterior scleral condensation and the ciliary pigment epithelium.
Iris
The iris develops from the rim of the optic cup (see Fig. 18.3 A ), which grows inward and partially covers the lens (see Figs. 18.7 and 18.8 ). The two layers of the optic cup remain thin in this area. The epithelium of the iris represents both layers of the optic cup; it is continuous with the double-layered epithelium of the ciliary body and with the retinal pigment epithelium and neural retina. The connective tissue framework (stroma) of the iris is derived from neural crest cells that migrate into the iris.
The dilator pupillae and sphincter pupillae muscles of the iris are derived from neuroectoderm of the optic cup. They appear to arise from the anterior epithelial cells of the iris. These smooth muscles result from a transformation of epithelial cells into smooth muscle cells.
Iris color is typically light blue or gray in most neonates. The iris acquires its definitive color as pigmentation occurs during the first 6 to 10 months. The concentration and distribution of pigment-containing cells (chromatophores) in the loose vascular connective tissue of the iris determine eye color. If the melanin pigment is confined to the pigmented epithelium on the posterior surface of the iris, the iris appears blue. If melanin is also distributed throughout the stroma (supporting tissue) of the iris, the eye appears brown. Iris heterochromia (mixed coloration) can result from changes to the sympathetic innervations to the eye.
Congenital aniridia is a rare anomaly. There is a lack of iris tissue or almost complete absence of the iris . This defect results from an arrest of development at the rim of the optic cup during the eighth week (see Fig. 18.3 A ). The defect may be associated with glaucoma, cataracts, and other eye abnormalities ( Figs. 18.10 and 18.11 ). Aniridia may be familial ; the trait may be transmitted in a dominant or sporadic pattern. Mutation of the PAX6 gene results in aniridia.


Lens
The lens develops from the lens vesicle , a derivative of the surface ectoderm (see Fig. 18.1 F and H ). The anterior wall of the vesicle, which is composed of cuboidal epithelium, becomes the subcapsular lens epithelium (see Fig. 18.8 C ). The nuclei of the tall columnar cells forming the posterior wall of the lens vesicle undergo dissolution (see Fig. 18.4 ). These cells lengthen considerably to form highly transparent epithelial cells, the primary lens fibers . As these fibers grow, they gradually obliterate the cavity of the lens vesicle (see Figs. 18.8 A to C and 18.9 ). Expression of PAX6 and SOX2 is required for the induction of the lens. The transcription factors PITX3, GATA3, and FOXE3 regulate the formation and differentiation of the lens fibers.
The rim of the lens is called the equatorial zone because it is located midway between the anterior and posterior poles of the lens (see Figs. 18.8 C and 18.9 ). The cells in the equatorial zone are cuboidal. As they elongate, they lose their nuclei and become secondary lens fibers . These new lens fibers are added to the external sides of the primary lens fibers. Although secondary lens fibers continue to form during adulthood and the lens increases in diameter, the primary lens fibers must last a lifetime.
The developing lens is supplied with blood by the distal part of the hyaloid artery (see Figs. 18.4 and 18.8 ). However, the lens becomes avascular in the fetal period, when this part of the hyaloid artery degenerates. Thereafter, the lens depends on diffusion from the aqueous humor in the anterior chamber of the eye (see Fig. 18.8 C ), which bathes its anterior surface, and from the vitreous humor (fluid component of vitreous body) in other parts. The developing lens is invested by a vascular mesenchymal layer, the tunica vasculosa lentis (see Fig. 18.8 C ). The anterior part of this capsule is the pupillary membrane (see Fig. 18.8 B ).
The pupillary membrane develops from the mesenchyme posterior to the cornea in continuity with the mesenchyme developing in the sclera. The part of the hyaloid artery that supplies the tunica vasculosa lentis disappears during the late fetal period (see Fig. 18.8 A and D ). The tunica vasculosa lentis and pupillary membrane degenerate (see Fig. 18.8 C and D ), but the lens capsule produced by the anterior lens epithelium and the lens fibers persists. This capsule represents a greatly thickened basement membrane and has a lamellar structure because of its development. The former site of the hyaloid artery is indicated by the hyaloid canal in the vitreous body (see Fig. 18.8 D ), which is usually inconspicuous in the living eye.
The vitreous body forms within the cavity of the optic cup (see Figs. 18.4 and 18.8 C ). It is composed of vitreous humor , which is its fluid component. The primary vitreous humor is derived from mesenchymal cells of neural crest origin, which secrete a gelatinous matrix ; this surrounding substance is called the primary vitreous body . The primary humor is surrounded later by a gelatinous secondary vitreous humor , which is thought to arise from the inner layer of the optic cup. The secondary humor consists of primitive hyalocytes (vitreous cells), collagenous material, and traces of hyaluronic acid.
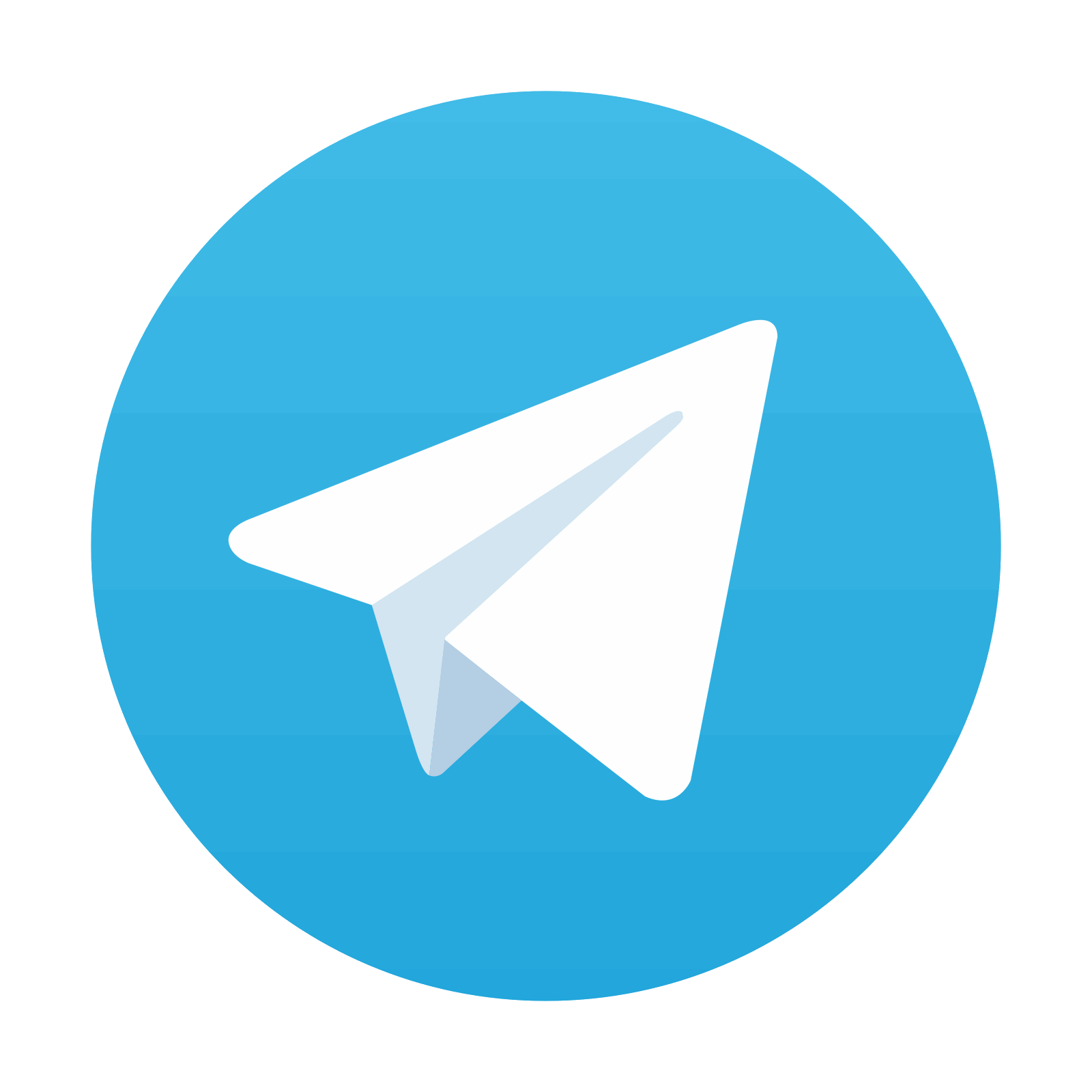
Stay updated, free articles. Join our Telegram channel

Full access? Get Clinical Tree
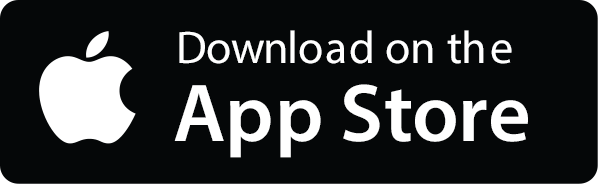
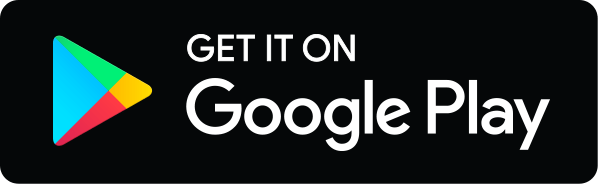
