Delivery Room Management
Virender K. Rehan
Roderic H. Phibbs
Although more than 90% of neonates undergo smooth feto/neonatal transition, of the 10% who require some resuscitative assistance during delivery, a small minority requires extensive resuscitative efforts (1,2). More importantly, because the need for resuscitation can come as a complete surprise, at every delivery there should be at least one person whose primary responsibility is the management of the newly born and who is capable of initiating resuscitation. Either this person or someone else who is immediately available should be skilled in all aspects of neonatal resuscitation. However, if the need for resuscitation is anticipated, additional skilled personnel should be called on before delivery. Furthermore, because many high-risk deliveries occur in nonteaching and smaller hospitals, all personnel involved in delivery room care of the newborn should be trained adequately in all aspects of neonatal resuscitation. All necessary resuscitation equipment should be checked and in working order before each delivery.
There is a high risk of asphyxia, defined as a combination of hypoxemia, hypercapnia, and acidosis, during labor, delivery, and in the first minutes after birth. This is because the newborn infant must successfully inflate his or her lungs and make adaptations to the circulation immediately after birth. Failure of either to occur leads to asphyxia. The key changes during feto/neonatal transition are the establishment of effective ventilation and perfusion of the lungs to raise partial pressure of arterial oxygen (PaO2) from the normal low levels of the fetus to the normal, relatively higher level of the neonate, together with the shutting down of the fetal circulatory pathways, which include the right-to-left shunts through the foramen ovale and the ductus arteriosus. Skillful resuscitation of infants with impaired transition can prevent brain damage and minimize subsequent morbidity and mortality. An understanding of the physiologic changes in the respiratory and circulatory systems that occur normally as the newborn infant adapts to extrauterine life is essential for a rational and effective approach to resuscitation.
RESPIRATORY ADAPTATION
At birth, the lungs must transition rapidly to become the site for gas exchange or else hypoxia and cyanosis (see Color Plate) will rapidly develop. For the lungs to exchange gas adequately after birth, the airways and the alveoli must be cleared of fetal lung fluid, and an increase in pulmonary blood flow must occur. In utero, fetal pulmonary vascular resistance is high and the fetal systemic vascular resistance is low; most of the cardiac output is shunted away from the lungs and is directed to the placenta where fetoplacental gas exchange occurs. Within minutes of delivery, the newborn’s pulmonary vascular resistance may decrease eight- to tenfold, causing a corresponding increase in neonatal pulmonary blood flow. Effective transition requires that the lung fluid be expelled or quickly absorbed to allow effective gas exchange. In fact, the decrease in lung fluid begins during labor (3). As a consequence of increased catecholamine levels, during labor, there is also an increase in lymphatic drainage. The absence of these physiologic events accounts for the increased incidence of transient tachypnea of the newborn after a cesarean section without labor. The first breath must generate a high transpulmonary pressure to overcome the viscosity of the lung fluid and the intraalveolar surface tension. It also helps to drive the alveolar fluid across the alveolar epithelium. Lung expansion and aeration also stimulate surfactant release with the resultant establishment of an air-fluid interface and development of functional residual capacity (FRC) (4). Normally, 80% to 90% of FRC is established within the first hour of birth in the term neonate with spontaneous respirations.
CIRCULATORY ADAPTATION
At birth, the clamping of the umbilical cord increases the systemic vascular resistance with a resultant increase in left ventricular and aortic pressures. Lung aeration and subsequent
gas exchange result in increased PaO2 and pH, which result in pulmonary vasodilation. These physiologic changes increase flow of blood to the left atrium via the pulmonary veins, so that left atrial pressure exceeds right atrial pressure, resulting in functional closure of foramen ovale. When pulmonary vascular resistance decreases to a level lower than the systemic vascular resistance, the ductus arteriosus closes functionally. As a result of the cessation of umbilical venous return, clamping of the umbilical cord also leads to the closure of the ductus venosus.
gas exchange result in increased PaO2 and pH, which result in pulmonary vasodilation. These physiologic changes increase flow of blood to the left atrium via the pulmonary veins, so that left atrial pressure exceeds right atrial pressure, resulting in functional closure of foramen ovale. When pulmonary vascular resistance decreases to a level lower than the systemic vascular resistance, the ductus arteriosus closes functionally. As a result of the cessation of umbilical venous return, clamping of the umbilical cord also leads to the closure of the ductus venosus.
Asphyxia has the potential to set in motion a series of responses that can not only impair the normal feto/neonatal transition, but may, in fact, reverse this process and lead to the persistence of the fetal circulatory state. Hypoxia keeps the ductus arteriosus open and causes pulmonary vasoconstriction leading to right-to-left flow across the ductus. Tissue hypoxia causes metabolic acidosis worsening the pulmonary vasoconstriction. The pulmonary hypertension leads to tricuspid insufficiency, raising right atrial pressure, which leads to right-to-left shunting of blood through the foramen ovale, causing further tissue hypoxia. However, in the majority of instances, with timely and appropriate resuscitation, the changes leading to a persistent fetal circulation like state can be reversed quickly.
PATHOPHYSIOLOGY OF INTRAPARTUM ASPHYXIA AND RESUSCITATION
Asphyxia occurs when the organ of gas exchange fails. When this happens, arterial carbon dioxide partial pressure (PaCO2) rises, and PaO2 and pH fall. Despite the low PaO2, tissues continue to consume O2, although at a lower rate in some organs. When the PaO2 is very low, anaerobic metabolism sets in, producing large quantities of metabolic acids. These are buffered partly by the bicarbonate in the blood (5).
The human infant is particularly vulnerable to asphyxia in the perinatal period. During normal labor, transient hypoxemia occurs with uterine contractions, but the healthy fetus tolerates this well. There are five basic events that lead to asphyxia during labor and delivery:
Interruption of umbilical blood flow (e.g., cord compression);
Failure of gas exchange across the placenta (e.g., placental abruption);
Inadequate perfusion of the maternal side of the placenta (e.g., severe maternal hypotension);
An otherwise compromised fetus who cannot further tolerate the transient, intermittent hypoxia of normal labor (e.g., the anemic or growth-retarded fetus); and
Failure to inflate the lungs and complete the changes in ventilation and lung perfusion that must occur at birth. This failure may occur because of airway obstruction, excessive fluid in the lungs, or weak respiratory effort. Alternatively, it may occur as a result of fetal asphyxia from one of the other four events, because fetal asphyxia often results in an infant who is acidotic and apneic at birth.
The umbilical cord blood pH, partial pressure of oxygen (PO2), partial pressure of carbon dioxide (PCO2), and calculated base excess are standard measures of fetal asphyxia (6,7,8). With fetal acidosis, the pH can vary over a wide range. Consequently, it is important to remember that pH is a logarithmic function of hydrogen ion concentration. A decrease of 0.3 pH units from 7.40 to 7.10 indicates only a 40 nmol/L increase in hydrogen ion (i.e., from 40 to 80 nmol), whereas a 0.3 decrease from 7.10 to 6.80 indicates an increase of 80 nmol/L (i.e., from 80 to 160 nmol). The gradient in blood gas tensions between umbilical artery and vein gives some indication of placental perfusion at the time of birth. The slower the flow of fetal blood through the placenta, the more complete the equilibration of gas tensions between fetal and maternal blood. For example, an arterial PO2 of 25 mm Hg with a venous PO2 of 32 mm Hg suggests good placental blood flow. An arterial PO2 of 12 mm Hg with a venous PO2 of 45 mm Hg suggests very slow flow. Metabolic acidosis suggests asphyxia, although some of the increased lactic acid in the blood may be a result of reduced uptake of lactate by the asphyxiated liver rather than increased lactate production from anaerobic metabolism (6,9). If asphyxia occurred just before birth, there may be lactic acid in the tissues that has not yet reached the central circulation. This will be detected only by blood gas measurements a few minutes after birth. If the fetus was asphyxiated an hour before delivery and recovered, that event may not be reflected in the umbilical cord blood gases at birth. Other indicators of asphyxia include plasma hypoxanthine, which increases because of lack of aerobic metabolism, plasma erythropoietin, which increases in response to fetal hypoxia, increased plasma levels of several lipid mediators, such as platelet-activating factor, and increased cerebrospinal fluid levels of several proinflammatory cytokines such as interleukin (IL)-1β, IL-6, and IL-8 (10,11,12).
Asphyxia in the fetus or newborn infant (including a preterm infant) is a progressive and reversible process. The speed and extent of progression are highly variable. Sudden, severe asphyxia can be lethal in less than 10 minutes. Mild asphyxia may progressively worsen over 30 minutes or more. Repeated episodes of brief, mild asphyxia may reverse spontaneously but produce a cumulative effect of progressive asphyxia. In the early stages, asphyxia usually reverses spontaneously if its cause is removed. Once asphyxia is severe, spontaneous reversal is unlikely because of the circulatory and neurologic changes that accompany it. Other sources provide a more detailed review of these phenomena (13,14).
Figure 18-1 schematically represents the sequence of pathophysiologic changes that accompany asphyxia. Although there are some quantitative differences between the changes that occur in the fetus and those in the newborn infant, the scheme generally applies to both. It is useful to consider the changes in both fetus and newborn infant together, because many cases of neonatal asphyxia
begin in the fetus and continue after birth. Cardiac output is maintained early in asphyxia, but its distribution changes radically. Selective regional vasoconstriction reduces blood flow to less vital organs and tissues such as gut, kidneys, muscle, and skin (15). Blood flow to the brain and myocardium increases, thereby maintaining adequate oxygen delivery despite reduced oxygen content of the arterial blood. Other organs and tissues must depend on increased oxygen extraction to maintain oxygen consumption (16,17). Pulmonary blood flow is low in the fetus. It is decreased further by hypoxia and acidosis (18). As a consequence of these adaptations, fetal oxygen consumption decreases (19).
begin in the fetus and continue after birth. Cardiac output is maintained early in asphyxia, but its distribution changes radically. Selective regional vasoconstriction reduces blood flow to less vital organs and tissues such as gut, kidneys, muscle, and skin (15). Blood flow to the brain and myocardium increases, thereby maintaining adequate oxygen delivery despite reduced oxygen content of the arterial blood. Other organs and tissues must depend on increased oxygen extraction to maintain oxygen consumption (16,17). Pulmonary blood flow is low in the fetus. It is decreased further by hypoxia and acidosis (18). As a consequence of these adaptations, fetal oxygen consumption decreases (19).
Early in asphyxia, newborns make vigorous attempts to inflate their lungs. If successful, the lungs become adequately ventilated and perfused, but the mere presence of gasping does not ensure that this will happen. As asphyxia becomes more severe, the respiratory center is depressed, and the chances of an infant spontaneously establishing effective ventilation and pulmonary perfusion diminish.
If asphyxia progresses to the severe stage, oxygen delivery to the brain and heart decreases. The myocardium then uses its stored reserve of glycogen for energy. Eventually, the glycogen reserve is consumed and the myocardium is exposed simultaneously to progressively lower values of PO2 and pH. The combined effects of hypoxia and acidosis lead to decreased myocardial function and decreased blood flow to the vital organs (20,21). Brain injury begins late during this phase (13,14).
This sequence of cardiovascular events is manifested by changes in heart rate and aortic and central venous pressures (see Fig. 18-1), all of which are measured easily in the newborn immediately after birth. The early bradycardia and hypertension are caused by the reflexes that shunt blood away from nonvital organs. Early in asphyxia, central venous (i.e., right atrial) pressure may rise slightly, owing to pulmonary hypertension and constriction of systemic capacitance vessels. As the myocardium fails, central venous pressure rises further, aortic pressure decreases, and heart rate is reduced further.
The initial adaptations of the systemic circulation to asphyxia are mediated by various physiologic reflexes (22). There also are major hormonal responses to asphyxia, including elevations in plasma corticotrophin, glucocorticoids, catecholamines, arginine vasopressin, renin, and atrial natriuretic factor, and a decrease in insulin (23,24). Some of these are important in maintaining the circulatory adaptations to asphyxia. Catecholamines, which come mainly from the adrenal medulla, maintain myocardial function in the presence of asphyxia, thereby increasing survival (25,26). Arginine vasopressin helps maintain the hypertension, bradycardia, and redistribution of systemic flow (27). Increased hepatic glycogenolysis helps maintain plasma glucose concentrations (9).
The physiology of resuscitation is essentially a reversal of the pathophysiology of asphyxia. In Fig. 18-1, which illustrates both processes, asphyxia proceeds from left to right, and resuscitation from right to left. It is crucial to determine where the infant is in this sequence of pathophysiologic events when resuscitation is started. If asphyxia has proceeded to myocardial failure, resuscitation must include restoration of cardiac output as well as establishment of effective ventilation and perfusion of the lungs. Generally, myocardial failure does not occur until both pH and PaO2 are extremely low, approximately 6.9 and 20 mm Hg, respectively. Cardiac output is reestablished through rapid correction of the severe hypoxia and acidosis. Until this is done, output must be maintained by cardiac massage. As soon as pH is raised to approximately 7.1 and PaO2 to 50 mm Hg, the myocardium responds rapidly, heart rate rises, aortic pressures rise, and pulse pressure widens, whereas central venous pressure falls. These changes indicate that cardiac massage can be stopped. At this point, the infant may be hypertensive because the vasoconstriction in nonvital organs is still present. This vasoconstriction is relieved only by continued adequate oxygenation and correction of acidosis. Pressures then will fall toward normal. The vasoconstriction also is manifested by intense pallor of the skin. As the vasoconstriction is relieved, the skin becomes pink and well perfused, with rapid capillary refilling (i.e., less than 2 seconds) when blanched by pressure. As peripheral flow improves, lactic
acid sequestered in these tissues enters the central circulation and a large base deficit, which may have been corrected earlier, now reappears.
acid sequestered in these tissues enters the central circulation and a large base deficit, which may have been corrected earlier, now reappears.
If asphyxia is only moderately severe, resuscitation begins in the middle of the sequence depicted in Fig. 18-1. There is hypertension, indicating that the myocardium has not yet failed. Effective ventilation of the lungs with a high oxygen concentration may correct acidosis by lowering the PaCO2, oxygenating the blood, and adequately dilating the pulmonary vascular bed. If significant acidosis persists after alleviation of the hypercarbia, alkali might be needed to correct the metabolic component of the acidosis, relieve pulmonary vasoconstriction, and establish good pulmonary perfusion. Generally, raising pH to 7.25 is sufficient for this purpose, but there are some important exceptions (discussed below) in which a higher pH is needed to dilate the pulmonary vascular bed.
When the effects of asphyxia are alleviated, spontaneous respiratory efforts return. The duration between the onset of resuscitation and reappearance of spontaneous respiratory efforts is directly proportional to the amount of brain injury that has occurred (13).
Onset of spontaneous respiratory efforts is not necessarily an indication to withdraw assisted ventilation. Often, there is residual atelectasis and the infant does not have strong, regular respiratory efforts. PaCO2 may be normal, and PaO2 may rise to a high level with assisted ventilation. But when assisted ventilation is withdrawn, effective ventilation may decrease and the whole process of asphyxia recurs. Therefore, assisted ventilation and supplemental oxygen should be only gradually withdrawn.
The blood volume of the asphyxiated infant may be abnormal. Asphyxia during labor usually shifts blood from the placenta to the fetus. There are certain situations, however, in which the infant’s blood volume may be reduced. The most obvious of these is hemorrhage from the fetoplacental unit, which is manifested by vaginal bleeding. Three other conditions that shift blood volume from the fetus to the placenta are compression of the umbilical cord, in which umbilical venous flow is reduced selectively more than arterial flow; severe hypotension in the mother; and asphyxia occurring only at the end of labor (28).
Initially, it may be difficult to determine whether or not blood volume is adequate in the asphyxiated newborn. There are two reasons for this. First, many of the circulatory responses to asphyxia are similar to those associated with loss of blood volume. Either asphyxia or hypovolemia may cause bradycardia, metabolic acidosis, poor peripheral perfusion indicated by pallor and slow capillary filling, and a large difference between core and skin temperature. A low aortic pressure could be a result of either the end stage of asphyxia or to shock. Only changes in central venous pressure are in the opposite direction, and even here the coexistence of the two processes can have offsetting effects. Second, the circulatory changes during asphyxia and resuscitation may determine the adequacy or inadequacy of the circulating blood volume. If an infant is moderately asphyxiated and has systemic and pulmonary vasoconstriction (see Fig. 18-1, center) and a small blood volume, aortic and central venous pressures will be nearly normal. Administration of a blood volume expander at this point would only overload the circulation. The effects of volume expansion would be even worse if the asphyxia were more severe and myocardial failure were present. Correction of asphyxia (see Fig. 18-1, going from right to left) relieves the vasoconstriction of resistance and capacitance vessels, and the small blood volume now becomes inadequate to support the circulation. Reperfusion of asphyxic and ischemic tissues also increases loss of intravascular water from these capillary beds, leading to edema and reduced plasma volume.
During recovery from asphyxia, several metabolic abnormalities appear. There may be hypoglycemia caused by depletion of carbohydrate reserves during the asphyxia. Hypoglycemia must be prevented because it can cause myocardial failure in a heart recently subjected to asphyxia (29). Hyperglycemia caused by excessive glucose administration similarly is dangerous during asphyxia because it worsens the acidosis by increasing lactic acid production (30). Hypocalcemia also develops, possibly as a result of increased calcitonin release during asphyxia (31), and can lead to myocardial failure.
Hyperkalemia occurs during asphyxia, when, in the process of buffering acidosis, H+ enters the erythrocytes and K+ is displaced from them. Although this increases plasma K+ while the patient is asphyxiated, total body K+ decreases as some of the K+ is excreted by the kidney. On relief of asphyxia, the buffering processes are reversed and K+ leaves the plasma and reenters the erythrocytes, leading to hypokalemia.
HIGH-RISK PREGNANCIES
Certain situations during pregnancy, labor, or delivery carry an increased risk of intrapartum asphyxia. If these high-risk deliveries are identified before birth, their progress during labor and delivery should be closely monitored and resuscitation can be initiated at birth. Tables 18-1 and 18-2 list some of the factors that alert the physician to a high-risk delivery. Optimal management of these cases requires good communication between the obstetrician, anesthesiologist, and pediatrician.
RESUSCITATION OF THE ASPHYXIATED INFANT
If a severely asphyxiated infant is expected, a resuscitation team must be present at delivery. In the majority of instances, good communication between the obstetrician and pediatrician will provide timely notice of the impending delivery of an asphyxiated infant. The actual extent of resuscitation needed can be determined only after someone with considerable clinical experience evaluates the infant’s condition. It is helpful to assign the responsibility of each member of the resuscitation team before delivery.
The following is an example of outlining the responsibilities of each member of the resuscitation team.
The following is an example of outlining the responsibilities of each member of the resuscitation team.
TABLE 18-1 SOME FACTORS THAT PLACE THE NEWBORN AT HIGH RISK FOR ASPHYXIA | ||||||||||||||||||||||||||||||
---|---|---|---|---|---|---|---|---|---|---|---|---|---|---|---|---|---|---|---|---|---|---|---|---|---|---|---|---|---|---|
|
Member A:
Assess infant.
Manage airway and intubate the trachea, if needed.
Provide positive-pressure ventilation.
Secure endotracheal tube (ET).
Member B:
Listen for heart rate and, if needed, give cardiac massage.
Auscultate chest to be sure ET is in proper position and gas exchange is good.
Catheterize umbilical vessel or vessels and maintain patency of catheters.
Measure intravascular pressures, assess perfusion, sample blood for pH, PO2, and PCO2, and draw blood cultures.
Administer fluids and drugs.
Continue assessment of infant.
TABLE 18-2 FETAL HEART RATE PATTERNS ASSOCIATED WITH FETAL AND NEONATAL DISTRESS | ||||||||||||
---|---|---|---|---|---|---|---|---|---|---|---|---|
|
Member C:
Blot baby dry; apply electrocardiograph (ECG) monitor leads, radiant monitor servocontrol, and transcutaneous oxygen sensor.
Keep timed, written record of resuscitation and vital signs and assign the Apgar scores at 1 and 5 minutes and every 5 minutes thereafter until the score is 7 or greater; time and record the rate and volume of infusions such as alkali and blood volume expanders.
Assist member A by providing ET suction, adjusting the fraction of oxygen inspired (FiO2), and helping to secure ET.
Help member B by providing medications and blood volume expanders in sterile syringes; B is working in a sterile field early in resuscitation.
Monitor baby’s temperature and capillary blood glucose.
Resuscitation Equipment and Supplies for Optimal Resuscitation (Modified from Reference 1)
All resuscitation equipment (listed below) should be checked before each delivery and should be fully operational.
Resuscitation table with heat source
Suction equipment
Bulb syringe
Mechanical suction and tubing
Suction catheters: 5 Fr, 6 Fr, 8 Fr, 10 Fr, and 12 Fr
8 Fr feeding tube and 20 mL syringe
Meconium aspirator
Bag and mask equipment
Neonatal resuscitation bag with a pressure-release valve or pressure manometer (the bag must be capable of delivering 90% to 100% oxygen)
Face masks, newborn and premature sizes (cushioned-rim masks preferred)
Oxygen source with flowmeter (flow rate up to 10 L/min) and tubing
Intubation equipment
Laryngoscope with straight blades: No. 00 (very-low-birth-weight infant); No. 0 (preterm infant); and No. 1 (term infant)
Endotracheal tubes, 2.5-, 3.0-, 3.5-, 4.0-mm internal diameter (ID)
Stylet (optional)
Scissors
Tape or securing device for ET
Alcohol sponges
CO2 detector (optional)
Laryngeal mask airway (optional)
Medications
Epinephrine 1:10,000 (0.1 mg/mL)-3-mL or 10-mL ampules
Isotonic crystalloid (normal saline or Ringer lactate) for volume expansion: 100 or 250 mL
Sodium bicarbonate 4.2% (5 mEq/10 mL)-10-mL ampules
Naloxone hydrochloride 0.4 mg/mL-1-mL ampules or 1.0 mg/mL-2-mL ampules
Dextrose 10%, 250 mL
Normal saline for flushes
Feeding tube, 5 Fr (optional)
Umbilical vessel catheterization supplies
Sterile gloves
Scalpel or scissors
Povidone-iodine solution
Umbilical tape
Umbilical catheters, 3.5 Fr, 5 Fr
Three-way stopcock
Syringes: 1, 3, 5, 10, 20, and 50 mL
Needles: 25, 21, and 18 gauge, or puncture device for needleless system
Miscellaneous
Gloves and appropriate personal protection
Radiant warmer or other heat source
Firm, padded resuscitation surface
Clock (timer optional)
Warmed linens
Stethoscope (neonatal head preferred)
Tape: ½ or ¾ inch
Cardiac monitor and electrode or pulse oximeter and probe
Oropharyngeal airways (0, 00, 000 sizes or 30-, 40-, and 50-mm lengths)
Arterial and venous pressure monitor with waveform displays; transducers can be connected to the catheters beforehand so that aortic pressure is displayed as soon as the umbilical artery catheter is inserted, and the venous waveform can be used to localize the catheter tip in the thoracic inferior vena cava (32)
Indirect blood pressure monitor
Tube thoracostomy tray with instruments and catheters from 10 to 14 Fr
Blood gas electrodes with a trained operator of the blood gas machines close enough to the resuscitation area so that results are available in less than 5 minutes
In selected situations (see the section “Special Problems”), it is useful to have present in the delivery room a unit of whole blood or packed erythrocytes that have been cross-matched against the mother; this blood can be kept in a cold pack and returned to the blood bank if not used.
Clinical Assessment of Severity of Asphyxia
The Apgar score was the first attempt at a systematic assessment of birth asphyxia (6,33). There is a loose correlation between low Apgar scores and umbilical cord blood gases. However, some infants with severe acidosis have normal Apgar scores, and some with normal blood gases and pH have very low scores (34,35). Maternal anesthetics, sedatives, maternal drugs, fetal sepsis, and central nervous system pathologic conditions can lower the Apgar score; extremely premature infants often have low scores without any other evidence of asphyxia (36,37). Regard-less of the cause, an Apgar score that remains low calls for action. The clinical significance of the Apgar score increases with time. Scoring should continue every 5 minutes until the score increases to 7 or above. The length of time it takes to reach a score of 7 is a rough indication of severity of asphyxia. Umbilical cord blood gases, discussed previously, are useful measures of fetal asphyxia, but this information will not be available until a few minutes after birth, and resuscitation must be started before that. Thus, their main value is in guiding subsequent management of the infant.
It is important to point out that the term perinatal asphyxia should be used with caution because an inaccurate use of this term may have medicolegal ramifications. It should be reserved in the clinical context of damaging hypoxemia and metabolic acidosis. A neonate who suffers from hypoxemia proximate to delivery that is severe enough to result in hypoxic encephalopathy will show other evidence of hypoxic damage, including severe metabolic or mixed acidemia (pH <7.00) on an umbilical cord arterial sample; persistence of an Apgar score of 0 to 3 for longer than 5 minutes; neonatal neurologic sequelae, for example, seizures, coma, hypotonia; and evidence of multiorgan system dysfunction. However, because neonatal neurologic problems may be attributed to factors other
than those involving labor and delivery, the term neonatal depression should be used when cause is uncertain.
than those involving labor and delivery, the term neonatal depression should be used when cause is uncertain.
The following resuscitation approach is based mostly on the recommendations of the American Academy of Pediatrics and the American Heart Association for neonatal resuscitation (1). However, it must be pointed out that most of these recommendations are based on anecdotal experience and a review of the available literature, but have not been rigorously tested. It is expected that in the future some of these recommendations will undergo close scrutiny and possible change.
Initial Steps in Resuscitation
Initial assessment, performed within a few seconds of delivery, dictates the extent of resuscitation needed. Initial assessment includes determining whether or not the infant is breathing, if there is good muscle tone, if the color is pink, and whether the infant looks term or preterm. If the infant is term, vigorous, without any known risk factors, and born through clear amniotic fluid, the infant need not be separated from the mother to receive initial care. Thermal care can be given by putting the infant on the mother’s chest (direct skin-to-skin contact), drying the infant, and covering the infant with dry linen. If the infant is apneic, gasping, has decreased muscle tone, or is cyanotic, immediate resuscitation is needed. Place the infant under a radiant warmer; quickly towel dry the baby; open the airway by laying the infant in the sniffing position; suction the mouth first and then the nose; provide tactile stimulation (by gently slapping or flicking the soles of the feet or by gently rubbing the back); and, if necessary, give oxygen. In the majority of instances, with these initial steps, the infant will start breathing adequately and demonstrate a color change to pinkish. If the infant starts breathing adequately, but continues to have central cyanosis, provide free-flow 100% oxygen. However, if the infant does not start breathing adequately or has a heart rate of less than 100 beats per minute, positive pressure ventilation (PPV) should be instituted immediately. The entire process up to this point should not take more than 20 to 30 seconds.
Positive Pressure Ventilation
Ensuring adequate ventilation is the single most important step in the cardiopulmonary resuscitation of the compromised newborn infant (38). Indications for initiating PPV include (a) apnea or gasping breaths; (b) heart rate of less than 100 beats per minute (bpm); and (c) persistent central cyanosis despite the provision of free-flow 100% oxygen. Initial PPV can be provided by either a flow-inflating bag (also called an anesthesia bag) or a self-inflating bag. Each type has its own advantages and disadvantages, and the resuscitator should be familiar with the type of bag used in his or her institution. If PPV is needed for a term infant, provide bag-and-mask ventilation at a rate of 40 to 60 breaths per minute with initial pressures of as high as 30 to 40 cm H2O. Subsequently, however, one should ventilate with the lowest pressures required to produce a gentle chest rise. Begin ventilation by slowly applying a pressure of 20 to 30 cm H2O. Maintain this inflating pressure for 1 to 2 seconds, then ventilate at a rate of 40 to 60 breaths per minute, using an inflation time of 0.3 to 0.5 seconds and enough pressure to provide a visible rise of the upper portion of the chest. Repeat the application of the initial inflating pressure pattern three to four times over the first 2 minutes. These prolonged breaths inflate regions of the lungs that were gasless and create necessary FRC (39). Figure 18-2 illustrates this process. Care must be taken to avoid rapid inflation or overdistension of the lungs.
It is important to realize that infants respond to initial lung inflation by eliciting a variety of physiologic responses. The infant may respond by the “rejection response,” in which the infant responds to PPV with a positive intraesophageal pressure to resist the inflation, that is, the infant actively resists attempts to inflate the lungs by generating an active exhalation. This response acts to not only reduce lung inflation, but also may cause high transient inflation pressures. Another response is “Head’s paradoxical response” in which the neonate responds to PPV with an inspiratory effort, causing a negative intraesophageal pressure. This inspiratory effort, with the resultant negative pressure produces a fall in inflation pressures but results in a transient increase in tidal volume. Of course, the neonate may demonstrate no response to the inflation attempt, that is, not generating any change in intraesophageal pressure during the positive pressure inflation, and passive inflation subsequently results. It is important to recognize that these physiologic responses to positive pressure inflation in the delivery room may cause large variability in the tidal volume and intrapulmonary pressures, despite constant delivery of inflation pressure.
The very premature infant with a very small lung volume and a surfactant-deficient lung presents special problems for initial ventilation that are discussed later (see section “Special Problem”). Whether low tidal volume ventilation starting in the delivery room, in an attempt to avoid lung injury and hypocapnia in premature infants, can be safely delivered, and whether it is as efficacious as normal tidal volume ventilation remains to be seen.
In the majority of instances, provision of appropriate PPV is followed by an increase in heart rate, improvement in color, and spontaneous breathing. Rate and pressures of PPV should be gradually reduced before deciding to see if the infant will tolerate its discontinuation. Free-flow oxygen may be continued as long as necessary to keep the infant pink. Some of the factors determining the success of PPV using a bag and mask include choosing the correct size mask, proper positioning of the infant, achieving tight seal between the face and the mask, and using adequate inspiratory pressure. If despite correct bag-and-mask PPV the infant fails to improve or continues to deteriorate, consider beginning chest compressions and bag-and-endotracheal-tube ventilation.
Chest Compressions
Chest compressions are infrequently needed during neonatal resuscitation. However, infants who have a heart rate of less than 60 bpm, despite 30 seconds of effective PPV, need immediate chest compressions (Fig. 18-3) Chest compressions can be provided by either the thumb technique or the two-finger technique, the thumb technique being preferred. Chest compressions are performed by placing thumbs or finger on the sternum immediately above the xiphoid and compressing at a rate of 90 per minute with an accompanying breath rate of 30 per minute (chest-compressions-to-ventilation ratio = 3:1). To provide effective chest compressions, one should ensure that the depth of compressions is one-third the depth of the chest, thumbs or fingers remain in contact with the chest at all times, the duration of the downward stroke of the compression is shorter than that of the release, compressions are well coordinated with ventilation, and there is adequate chest movement during ventilation. Continue chest compressions until the heart rate is greater than 60 bpm
and ventilate until heart rate is greater than 100 bpm, at which point the infant’s own respiratory effort is assessed for adequacy. Ventilation can then be discontinued if heart rate remains above 100 bpm and the infant continues to breathe spontaneously. However, if the heart rate remains below 60 bpm despite effective chest compressions and ventilation, ET intubation (if it has not been already done) and administration of medications may be needed. However, it is important to realize that the most important reason for continued bradycardia during resuscitation is failure to establish effective postnatal ventilation rather than perinatal asphyxia. Therefore, it important to make every effort to optimize ventilation before chest compressions and medication administration are considered.
and ventilate until heart rate is greater than 100 bpm, at which point the infant’s own respiratory effort is assessed for adequacy. Ventilation can then be discontinued if heart rate remains above 100 bpm and the infant continues to breathe spontaneously. However, if the heart rate remains below 60 bpm despite effective chest compressions and ventilation, ET intubation (if it has not been already done) and administration of medications may be needed. However, it is important to realize that the most important reason for continued bradycardia during resuscitation is failure to establish effective postnatal ventilation rather than perinatal asphyxia. Therefore, it important to make every effort to optimize ventilation before chest compressions and medication administration are considered.
Endotracheal Intubation
Endotracheal intubation is probably the most difficult step in neonatal resuscitation and the one that requires constant practice to maintain sharp skills in this technique. Someone experienced in endotracheal intubation should be available to assist at every delivery. Therefore, if the resuscitator is not comfortable with endotracheal intubation, he/she should focus on providing effective ventilation via bag and mask until somebody experienced in endotracheal intubation arrives. Indications for endotracheal intubation include (a) to suction meconium; (b) to improve ventilation when bag-and-mask ventilation is ineffective; (c) to coordinate ventilation and chest compressions; (d) to administer medications such as epinephrine; (e) when prolonged ventilation is needed, for example, extreme prematurity; (f) to administer surfactant; and (g) when congenital diaphragmatic hernia is suspected (probably the only absolute indication for endotracheal intubation).
The correct size laryngoscope blade is No. 1 for a term and No. 0 for a preterm infant. For a very-low-birth-weight (VLBW) infant, a size 00 blade may be used. The ET should be of uniform internal diameter as tubes with shoulders may obstruct the line of vision during insertion and are more likely to cause trauma to the vocal cords. The choice of ET is based on the infant’s weight or gestational age: for infants weighing less than 1000 g (<28 weeks’ gestational age), use a 2.5-mm internal diameter (ID) tube; for infants weighing 1000 to 2000 g (28 to 34 weeks’ gestational age), use a 3.0-mm ID tube; for infants weighing 2000 to 3000 g (34 to 38 weeks’ gestational age), use a 3.5-mm ID; and for larger infants, a 3.5- to 4.0-mm ID tube may be used. To minimize the risk of vocal cord trauma and the subsequent development of laryngeal stenosis, one should make sure that the inserted ET is not too tight a fit in the larynx. In general, gas should leak from the space between the ET and the trachea when 15 to 30 cm H2O pressure is applied to the airway. Cutting the tube at the 13- to 15-cm mark prior to insertion makes it easier to handle during the procedure, decreases the chances of inserting the tube too far, and decreases the resistance to airflow. The use of a stylet during ET insertion is optional. If a stylet is used to stiffen the ET, it should be ensured that its tip is approximately 0.5 cm above from the tip of the tube and does not protrude from the end or the side hole of the ET. In addition, the stylet should be well secured so that it does not advance farther into the ET during intubation. If the stylet extends beyond the tip of the ET, it could traumatize the airway.
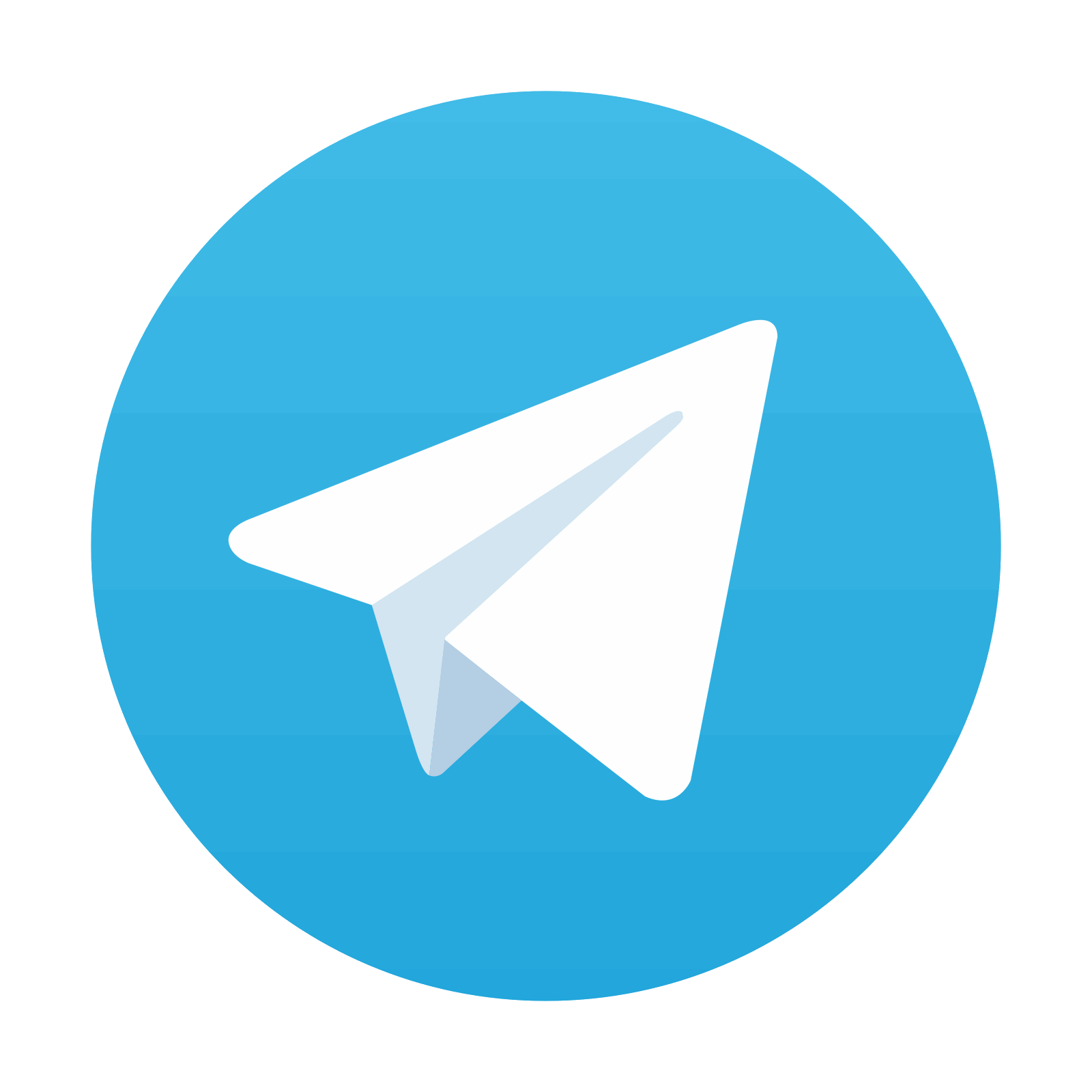
Stay updated, free articles. Join our Telegram channel

Full access? Get Clinical Tree
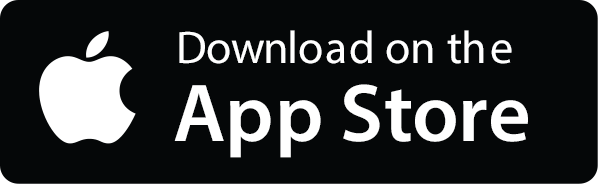
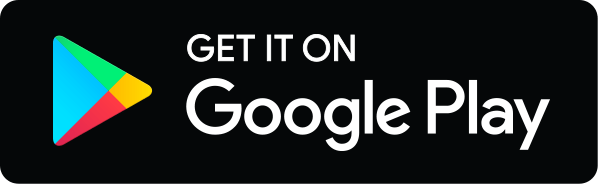
