Chapter 76 Cytogenetics
Clinical cytogenetics is the study of chromosomes: their structure, function, inheritance, and abnormalities. Chromosome abnormalities are very common and occur in approximately 1-2% of live births, 5% of stillbirths, and ~50% of early fetal losses in the 1st trimester of pregnancy. Chromosome abnormalities are more common among persons with mental retardation, and they have a significant role in the development of some neoplasias.
Chromosome analyses are indicated in persons presenting with multiple congenital anomalies, dysmorphic features, and/or mental retardation. The specific indications for studies include advanced maternal age (>35 yr) or multiple abnormalities on fetal ultrasound (prenatal testing), multiple congenital anomalies, unexplained growth retardation in the fetus or postnatal problems in growth and development, ambiguous genitalia, unexplained mental retardation with or without associated anatomic abnormalities, primary amenorrhea or infertility, recurrent miscarriages (≥3) or prior history of stillbirths and neonatal deaths, a 1st-degree relative with a known or suspected structural chromosome abnormality, clinical findings consistent with a known anomaly, some malignancies, and chromosome breakage syndromes (Bloom syndrome, Fanconi anemia).
76.1 Methods of Chromosome Analysis
Cytogenetic studies are usually performed on peripheral blood lymphocytes, although cultured fibroblasts may also be used. Prenatal (fetal) chromosome studies are performed with cells obtained from the amniotic fluid, chorionic villus tissue, and fetal blood or, in the case of preimplantation diagnosis, by analysis of a blastomere. Cytogenetic studies of bone marrow have an important role in tumor surveillance, particularly among patients with leukemia. These are useful to determine induction of remission and success of therapy or, in some cases, the occurrence of relapses.
Chromosome anomalies include abnormalities of number and structure and are the result of errors during cell division. There are 2 types of cell division: mitosis, which occurs in most somatic cells, and meiosis, which is limited to the germ cells. In mitosis, 2 genetically identical daughter cells are produced from a single parent cell. DNA duplication has already occurred during interphase in the S phase of the cell cycle (DNA synthesis). Therefore, at the beginning of mitosis the chromosomes consist of 2 double DNA strands joined together at the centromere known as sister chromatids. Mitosis can be divided into 4 stages: prophase, metaphase, anaphase, and telophase. Prophase is characterized by condensation of the DNA. Also during prophase, the nuclear membrane and the nucleolus disappear and the mitotic spindle forms. In metaphase, the chromosomes are maximally compacted and are clearly visible as distinct structures. The chromosomes align at the center of the cell and spindle fibers connect to the centromere of each chromosome and extend to centrioles at the 2 poles of the mitotic figure. In anaphase, the chromosomes divide along their longitudinal axes to form 2 daughter chromatids, which then migrate to opposite poles of the cell. Telophase is characterized by formation of 2 new nuclear membranes and nucleoli, duplication of the centrioles, and cytoplasmic cleavage to form the 2 daughter cells.
Meiosis begins in the female oocyte during fetal life and is completed years to decades later. In males, it begins in a particular spermatogonial cell sometime between adolescence and adult life and is completed in a few days. Meiosis is preceded by DNA replication so that at the outset each of the 46 chromosomes consists of 2 chromatids. In meiosis, a diploid cell (2n = 46 chromosomes) divides to form haploid cells (n = 23 chromosomes). Meiosis consists of 2 major rounds of cell division. In meiosis I, each of the homologous chromosomes pair precisely so that genetic recombination, involving exchange between 2 DNA strands (crossing over), can occur. This results in a reshuffling of the genetic information on the recombined chromosomes and allows further genetic diversity. Each daughter cell then receives 1 of each of the 23 homologous chromosomes. In oogenesis, 1 of the daughter cells receives most of the cytoplasm and becomes the egg, whereas the other smaller cell becomes the 1st polar body. Meiosis II is similar to a mitotic division but without a preceding round of DNA duplication (replication). Each of the 23 chromosomes divides longitudinally, and the homologous chromatids migrate to opposite poles of the cell. This produces 4 spermatogonia in males, or an egg cell and a 2nd polar body in females, each with a haploid (n = 23) set of chromosomes. Consequently, meiosis fulfills 2 crucial roles: It reduces the chromosome number from diploid (46) to haploid (23) so that upon fertilization a diploid number is restored, and it allows genetic recombination.
Two errors of cell division commonly occur during meiosis or mitosis, and either can result in an abnormal number of chromosomes. The 1st is nondisjunction, in which 2 chromosomes fail to separate during meiosis and thus migrate together into 1 of the new cells, producing 1 cell with 2 copies of the chromosome and another with no copy. The 2nd is anaphase lag, in which a chromatid or chromosome is lost during mitosis because it fails to move quickly enough during anaphase to become incorporated into 1 of the new daughter cells (Fig. 76-1).
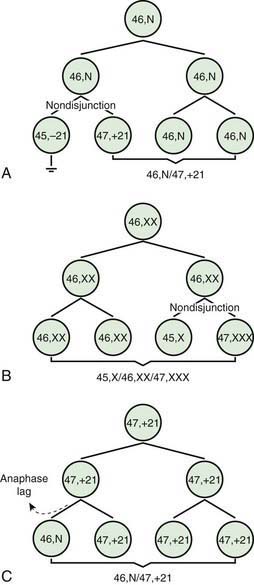
Figure 76-1 Generation of mosaicism. A, Postzygotic nondisjunction in an initially normal conceptus. In this example, 1 cell line (monosomic 21) is subsequently lost, with the final karyotype 46,N/47,+21. B, Postzygotic nondisjunction in an initially 46,XX conceptus, resulting in 45,X/46,XX/47,XXX mosaicism. C, Postzygotic anaphase lag in an initially 47,+21 conceptus.
(From Gardner RJM, Sutherland GR: Chromosome abnormalities and genetic counseling, ed 3, New York, 2003, Oxford University Press, p 33.)
For chromosome analysis, cells are cultured (for varying periods depending on cell type), with or without stimulation, and then artificially arrested in mitosis during metaphase (or prometaphase), later on subjected to a hypotonic solution to allow proper dispersion of the chromosomes for analysis, fixed, banded, and finally stained. The most commonly used banding and staining method is the GTG banding (G-bands trypsin Giemsa) also known as G banding, which produces a unique combination of dark (G-positive) and light (G-negative) bands that permits recognition of all individual 23 chromosome pairs for analysis.
Other banding techniques such as Q-banding using quinacrine, reverse (R-banding) using acridine orange, and C-banding (constitutive heterchromatin) using barium hydroxide are available for use in certain circumstances but are losing ground to molecular technologies. Metaphase chromosome spreads are 1st evaluated microscopically, and then their images are photographed or captured by a video camera and stored on a computer to be later analyzed. Humans have 46 chromosomes or 23 pairs, which are classified as autosomes for chromosomes 1 to 22, and the sex chromosomes, often referred as sex complement: XX for females and XY for males. The homologous chromosomes from a metaphase spread can then be paired and arranged systematically to assemble a karyotype according to well-defined standard conventions like those established by International System for Human Cytogenetic Nomenclature (ISCN), with chromosome 1 being the largest and 22 the smallest. According to nomenclature, the description of the karyotype includes the total number of chromosomes followed by the sex chromosome constitution. A normal karyotype is 46,XX for females and 46,XY for males (Fig. 76-2). Abnormalities are noted after the sex chromosome complement.
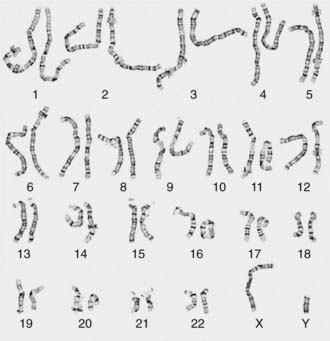
Figure 76-2 Karyotype of a normal male at the 550-600 band level. The longer the chromosomes are captured at metaphase or sometimes prometaphase, the more bands that can be visualized.
Although the internationally accepted system for human chromosome classification relies largely on the length and banding pattern of each chromosome, the position of the centromere relative to the ends of the chromosome also is a useful distinguishing feature (Fig. 76-3). The centromere divides the chromosome in 2, with the short arm designated as the p arm and the long arm designated as the q arm. A plus or minus sign before the number of a chromosome indicate that there is an extra or missing chromosome, respectively. Table 76-1 lists some of the abbreviations used for the descriptions of chromosomes and their abnormalities. A metaphase chromosome spread usually shows 450-550 bands. Prophase and prometaphase chromosomes are longer, are less condensed, and often show 550-850 bands. High-resolution analysis is useful for detecting subtle chromosome abnormalities that might otherwise go unrecognized.
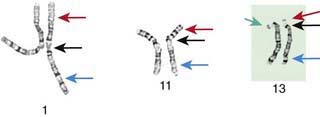
Figure 76-3 Example of different chromosome types according to the position of the centromere. On the left is a chromosome 1 pair with the centromere equidistant from the short and long arm (also known as metacentric). In the center is a chromosome 11 pair that is submetacentric. On the right is a chromosome 13 pair that is an example of an acrocentric chromosome. Acrocentric chromosomes contain a very small short arm, stalks, and satellite DNA. The black arrow indicates the position of the centromere. The blue arrow shows the long arm of a chromosome. The red arrow shows the short arm of a chromosome. The green arrow highlights the satellite region, which is made of DNA repeats. The light area between the short arm and the satellite is known as the stalk.
Molecular techniques such as fluorescence in situ hybridization (FISH) and comparative array genomic hybridization studies (conventional CGH and array CGH [aCGH]) have filled a significant void for the diagnosing cryptic chromosomal abnormalities. These techniques identify subtle abnormalities that are often below the resolution of standard cytogenetic studies. FISH is used to identify the presence, absence, or rearrangement of specific DNA segments and is performed with gene- or region-specific DNA probes. Several FISH probes are used in the clinical setting: unique sequence or single-copy probes, repetitive-sequence probes (alpha satellites in the pericentromeric regions), and multiple-copy probes (chromosome specific or painting). FISH involves using a unique known DNA sequence or probe labeled with a fluorescent dye that is complementary to the studied region of disease interest. The labeled probe is exposed to the DNA on a microscope slide, typically metaphase or interphase chromosomal DNA, that has been previously treated (denatured) to allow the DNA to become single stranded and to permit hybridization. When the probe pairs with its complementary DNA sequence, it can be then visualized by fluorescence microscopy (Fig. 76-4). In metaphase chromosome spreads, the exact chromosomal location of each probe copy can be documented and often the number of copies (deletions, duplications) of the DNA sequence as well, if they are not too close to each other; whereas in interphase cells, only the number of copies of a particular DNA segment can be determined. When the interrogated segments (as in genomic duplications) are close together, only interphase cells can accurately determine the presence of 2 or more copies or signals. In metaphase cells, some duplications might falsely appear as a single signal.
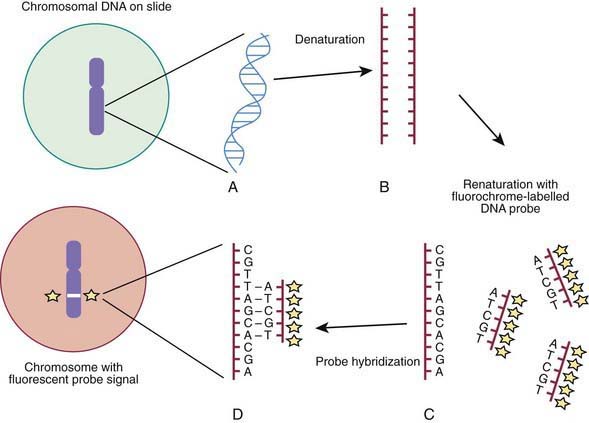
Figure 76-4 Fluorescence in situ hybridization (FISH) involves denaturation of double-stranded DNA as present in metaphase chromosomes or interphase nuclei on cytogenetic slide preparations (A) into single-stranded DNA (B). The slide-bound (in situ) DNA is then renatured or reannealed in the presence of excess copies of a single-stranded, fluorochrome-labeled DNA base-pair sequence or probe (C). The probe anneals or “hybridizes” to sites of complementary DNA sequence (D) within the chromosomal genome. Probe signal is visualized and imaged on the chromosome by fluorescent microscopy.
(From Lin RL, Cherry AM, Bangs CD, et al: FISHing for answers: the use of molecular cytogenetic techniques in adolescent medicine practice. In Hyme HE, Greydanus D, editors: Genetic disorders in adolescents: state of the art reviews. Adolescent medicine, Philadelphia, 2002, Hanley and Belfus, pp 305–313.)
Metaphase and interphase FISH are particularly useful for detecting very small deletions that might escape notice with G-band analysis. In most cases the probe used for identification is used in conjunction with a control probe with a known location nearby the region studied. This allows correct identification of the hybridized signal to the right chromosome, and in some cases identification to the rearranged chromosome. With high-resolution chromosome analysis it is very difficult to recognize deletions of <5 million bp (5 Mb); FISH can reliably detect deletions as small as 50 to 200 kb of DNA. This has allowed the clinical characterization of a number of microdeletion syndromes. In addition to gene- or locus-specific probes, complex mixtures of DNA from a chromosome arm or an entire chromosome are available for fluorescence staining of large chromosome sections or entire chromosomes. The probe mixtures are referred to as chromosome paints (Fig. 76-5A and B). Other probes hybridize to repetitive sequences located to the pericentromeric regions. These probes are useful for the rapid identification of certain trisomies in interphase cells of blood smears, or even in the rapid analysis of prenatal samples from cells obtained through amniocentesis. Such probes are available for chromosomes 13, 18, and 21 and for the sex pair X and Y (Fig. 76-5C and D).
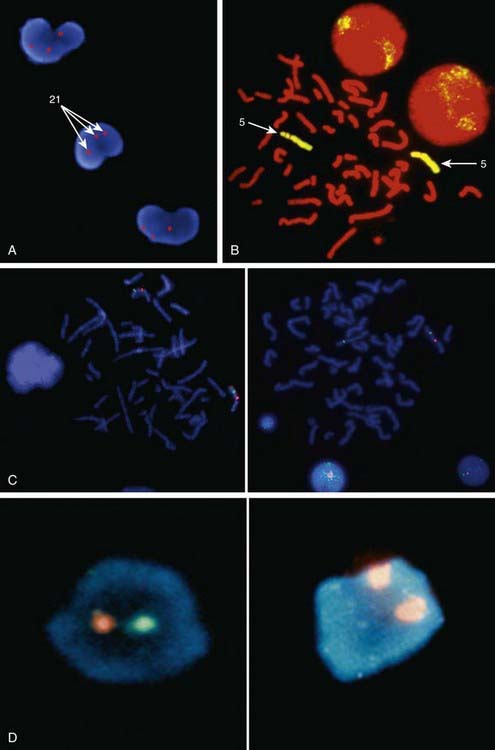
Figure 76-5 A, Fluorescence in situ hybridization (FISH) analysis of interphase peripheral blood cells from a patient with Down syndrome using a chromosome 21-specific probe. The 3 red signals mark the presence of 3 chromosomes 21. B, FISH analysis of a metaphase chromosome spread from a clinically normal individual using a whole-chromosome paint specific for chromosome 5. Both chromosomes 5 are completely labeled (yellow) along their entire length. C, Fluorescence in situ hybridization studies (FISH) on metaphase cells using a unique sequence probe that hybridizes to the elastin gene on chromosome 7q11.23, inside the Williams syndrome critical region. The elastin probe is labeled in red, and a control probe on chromosome 7 is labeled in green. The left image shows normal hybridization to chromosome 7, with 2 signals for the elastin region and 2 for the control probe. The right image shows a normal chromosome on the right with control and elastin signals, and a deleted chromosome 7 on the left, evidenced by a single signal for the control probe. This image corresponds to a patient with a Williams syndrome region deletion. D, FISH in interphase cells using DNA probes that hybridize to repetitive α-satellite sequences in the pericentromeric region for the sex chromosomes. Left, interphase cells with 2 signals, 1 labeled in red for the X chromosome and green for the Y chromosome, consistent with a normal male chromosome complement. Right, interphase cell showing 2 red signals for the X chromosome, compatible with a normal female chromosome complement.
Spectral karyotyping (SKY) and multicolor FISH (M-FISH) are similar molecular cytogenetic techniques that use 24 different chromosome painting probes and 5 fluorochromes to simultaneously visualize every chromosome in a metaphase spread. Each of the 24 different chromosome paints is labeled with a different combination of the 5 fluorescent dyes, which emit at different wavelengths. Each of the 22 autosomes and the X and Y chromosomes has its own unique spectrum of wavelengths of fluorescence. Special filters, cameras, and image-processing software are required to identify each chromosome. SKY and M-FISH are especially useful for identifying the complex chromosome rearrangements found in many tumors. This technique requires very special and costly equipment and is being displaced by comparative aCGH.
Comparative genomic hybridization (CGH) is a molecular-based technique that involves differentially labeling the patient’s DNA with a fluorescent dye (green) and a normal reference DNA with another fluorescent dye (red) (Fig. 76-6). Equal amounts of the two-label DNA samples are mixed and then used as a painting probe for FISH with normal metaphase chromosomes. The ratio of green : red fluorescence is measured along each chromosome. Regions of amplification of the patient’s DNA display an excess of green fluorescence, and regions of loss show excess red fluorescence. If the patient’s and the control DNA are equally represented, the green : red ratio is 1 : 1 and the chromosomes appear yellow.
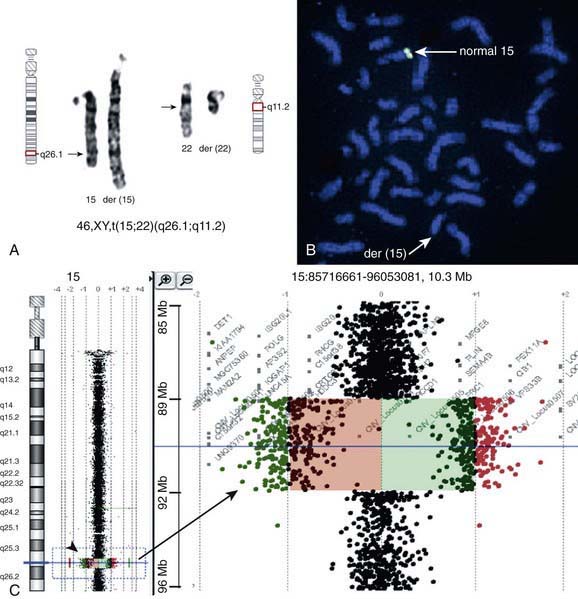
Figure 76-6 An example of a cryptic microdeletion at a translocation breakpoint of an apparently balanced translocation in a patient with DD and growth defect (with permission from the author and the publisher). A, Partial karyotype shows t(15;22)(q26.1;q11.2). B, FISH with clones 2O19 (green) and 354M14 (red) at 15q26.1; arrows indicate signals only present on the normal chromosome 15, suggesting a deletion on the der(15). C, Two-color aCGH with dye swap with 244 K oligo probes; arrowhead indicates a 3.3-Mb deletion at chromosome 15q26.1-q26.2, arrow points to the close-up view of the deletion.
(From Li MM, Andersson HC: Clinical application of microarray-based molecular cytogenetics: an emerging new era of genomic medicine, J Pediatr 155:311–317, 2009.)
A modified version of this technology, aCGH, uses DNA spotted onto a slide or microarray grid. In this case, instead of metaphase chromosomes, segments of DNA are represented by BACs (bacterial artificial chromosomes) and PACs (P1 artificial chromosomes) or oligonucleotides (short DNA segments of varied size) distributed in a microarray that resembles the chromosomes in a metaphase. The detection could reach the size of the BACs, PACs, and oligonucleotides and ranges in resolution from approximately 50 to 200 kb (even smaller if using cosmids or shorter polymerase chain reaction (PCR) products used as probes).
There are many advantages of aCGH. They can test all critical disease causing regions in the genome at once; FISH requires the clinical knowledge and tests only 1 area at a time. aCGH can detect duplications and deletions not currently recognized as recurrent disease-causing regions probed by FISH. aCGH can detect single and contiguous gene deletion syndromes. aCGH does not always require cell culture to generate sufficient DNA, something that may be important in the context of prenatal testing because of timing. There are disadvantages to aCGH: It does not detect balanced translocations, inversions, or very low-levels of mosaicism.
There are 2 different types of aCGH, targeted and whole-genome arrays. aCGH is the equivalent of doing thousands of FISH experiments in a single sample at once. Targeted aCGH is an effective and efficient technique for detecting clinically known cryptic chromosomal aberrations, which are typically associated with known disease phenotypes; many of these arrays have expanded detection to areas potentially susceptible to recurring deletion or duplication. Whole-genome array targets the entire genome. The advantage of this latter technique is that it allows better and denser coverage of the entire genome in evenly spaced portions; its disadvantage is that interpretation of deletions or duplications may be difficult if it involves areas not previously known to be involved in disease.
There are many copy number variations (CNVs) causing deletion or duplication in the human genome. Thus, most detected genetic abnormalities, unless associated with very well known clinical phenotypes, require parental investigations because a detected CNV that is inherited might turn out to be an incidental polymorphic variant. A de novo abnormality (i.e., 1 found only in the child and not the parents) is often more significant if it is associated with an abnormal phenotype and if it involves genes with important functions. aCGH is a very valuable technology alone or when combined with FISH and conventional chromosome studies (Fig. 76-7).
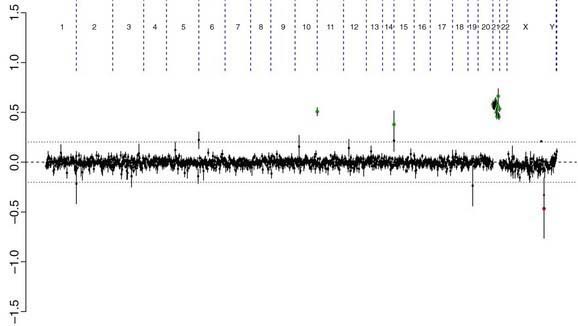
Figure 76-7 Array CGH in a female patient with Down syndrome. Each black dot represents a piece of DNA segment specific for different chromosome location. Most of the dots displayed between the 0.0 and 0.2 axis are considered within normal range. Exceptions are often due to polymorphic variations. A group of dots colored in green clusters on chromosome 21 and above 0.5. Those represent a gain in copy number of DNA segments for chromosome 21 as seen in Down syndrome and consistent with trisomy 21.
ACOG Practice Bulletin. Screening for fetal chromosomal abnormalities. Obstet Gynecol. 2007;109:217-227.
Bejjani BA, Saleki R, Ballif BC, et al. Use of targeted array-based CGH for the clinical diagnosis of chromosomal imbalance: is less more? Am J Med Genet. 2005;134A:259-267.
Li MM, Andersson HC. Clinical application of microarray-based molecular cytogenetics: an emerging new era of genomic medicine. J Pediatr. 2009;155:311-317.
Saugier-Veber P, Girard-Lemaire F, Rudolf G, et al. Genetic compensation in a human genomic disorder. N Engl J Med. 2009;360:1211-1216.
76.2 Down Syndrome and Other Abnormalities of Chromosome Number
Aneuploidy and Polyploidy
Human cells contain a multiple of 23 chromosomes (n = 23). A haploid cell (n) has 23 chromosomes (typically in the ovum or sperm). If a cell’s chromosomes are an exact multiple of 23 (46, 69, 92 in humans), those cells are referred to as euploid. Polyploid cells are euploid cells with more than the normal diploid number of 46 (2n) chromosomes: 3n, 4n. Polyploid conceptions are usually not viable, but the presence of mosaicism with a karyotypically normal line can allow survival. Mosaicism is an abnormality defined as the presence of 2 or more cell lines in a single individual. Polyploidy is a common abnormality seen in 1st-trimester pregnancy losses. Triploid cells are those with 3 haploid sets of chromosomes (3n) and are only viable in a mosaic form. Triploid infants can be liveborn but do not survive long. Triploidy is often the result of fertilization of an egg by 2 sperm (dispermy). Failure of 1 of the meiotic divisions, resulting in a diploid egg or sperm, can also result in triploidy. The phenotype of a triploid conception depends on the origin of the extra chromosome set. If the extra set is of paternal origin, it results in a partial hydatidiform mole with poor embryonic development, but triploid conceptions that have an extra set of maternal chromosomes results in severe embryonic retardation with a small fibrotic placenta that is typically spontaneously aborted.
Abnormal cells that do not contain a multiple of haploid number of chromosomes are termed aneuploid cells. Aneuploidy is the most common and clinically significant type of human chromosome abnormality, occurring in at least 3-4% of all clinically recognized pregnancies. Monosomies occur when only 1, instead of the normal 2, of a given chromosome is present in an otherwise diploid cell. In humans, most autosomal monosomies appear to be lethal early in development, and survival is possible in mosaic forms or by means of chromosome rescue (restoration of the normal number by duplication of single monosomic chromosome). An exception to this rule is monosomy for the X chromosome (45,X), seen in Turner syndrome; it has been estimated that the majority of 45,X conceptuses are lost early in pregnancy for as yet unexplained reasons.
The most common cause of aneuploidy is nondisjunction, the failure of chromosomes to disjoin normally during meiosis (see Fig. 76-1). Nondisjunction can occur during meiosis I or II or during mitosis. After meiotic nondisjunction, the resulting gamete either lacks a chromosome or has 2 copies instead of 1 normal copy, resulting in a monosomic or trisomic zygote, respectively.
FISH is a technique that can be used for rapid diagnosis in the prenatal detection of common fetal aneuploidies including chromosomes 13, 18, and 21 as well as sex chromosomes (see Fig. 76-5C and D). The most common numerical abnormalities in liveborn children include trisomy 21 (Down syndrome), trisomy 18 (Edwards syndrome), trisomy 13 (Patau syndrome), and sex chromosomal aneuploidies: Turner syndrome (usually 45,X), Klinefelter syndrome (47,XXY), 47,XXX, and 47,XYY, By far the most common type of trisomy in liveborn infants is trisomy 21 (47,XX,+21 or 47,XY,+21). Trisomy 18 and trisomy 13 are relatively less common and are associated with a characteristic set of congenital anomalies and severe mental retardation (Table 76-2). The occurrence of trisomy 21 and other trisomies increases with advanced maternal age (≥35 yr). Owing to this increased risk, women who are ≥35 yr at the time of delivery should be offered genetic counseling and prenatal diagnosis (including serum screening, ultrasonography, and amniocentesis or chorionic villus sampling; Chapter 90).
Table 76-2 CHROMOSOMAL TRISOMIES AND THEIR CLINICAL FINDINGS
Down Syndrome
Trisomy 21 is the most common genetic cause of moderate mental retardation. The incidence of Down syndrome in live births is approximately 1 in 733; the incidence at conception is more than twice that rate; the difference is accounted by early pregnancy losses. In addition to cognitive impairment, Down syndrome is associated with congenital anomalies and characteristic dysmorphic features (Figs. 76-8 and 76-9; Table 76-3). Although there is variability in the clinical features, the constellation of phenotypic features is fairly consistent and permits clinical recognition of trisomy 21. Affected individuals are more prone to congenital heart defects (50%) such as atrioventricular septal defects, ventricular septal defects, isolated secundum atrial septal defects, patent ductus arteriosus, and tetralogy of Fallot. Congenital and acquired gastrointestinal anomalies and hypothyroidism are common (Table 76-4). Other abnormalities include megakaryoblastic leukemia, immune dysfunction, diabetes mellitus, and problems with hearing and vision (see Table 76-4). Alzheimer disease–like dementia is a known complication that occurs as early as the 4th decade and has an incidence 2-3 times higher than sporadic Alzheimer disease. Most males with Down syndrome are sterile, but some females have been able to reproduce, with a 50% chance of having trisomy 21 pregnancies. Two genes (DYRK1A, DSCR1) in the putative critical region of chromosome 21 may be targets for therapy.
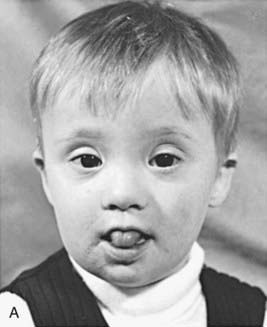
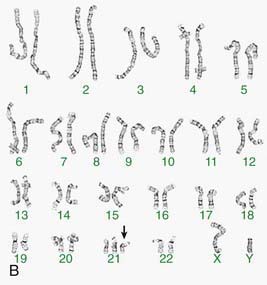
Figure 76-8 A, Face of a child with Down syndrome. B, Karyotype of a male with trisomy 21 as seen in Down syndrome. This karyotype reveals 47 chromosomes instead of 46, with an extra chromosome in pair 21.
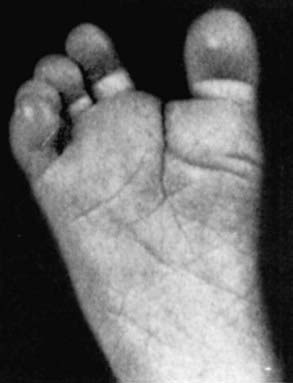
Figure 76-9 Prehensile foot in a 1-mo-old child.
(From Wiedemann HR, Kunze J, Dibbern H: Atlas of clinical syndromes: a visual guide to diagnosis, ed 3, St Louis, 1989, Mosby.)
Table 76-3 CLINICAL FEATURES OF DOWN SYNDROME IN THE NEONATAL PERIOD
CENTRAL NERVOUS SYSTEM
CRANIOFACIAL
CARDIOVASCULAR
MUSCULOSKELETAL
GASTROINTESTINAL
CUTANEOUS
Cutis marmorta
Table 76-4 ADDITIONAL FEATURES OF DOWN SYNDROME THAT CAN DEVELOP OR BECOME SYMPTOMATIC WITH TIME
NEUROPSYCHIATRIC
SENSORY
CARDIOVASCULAR
MUSCULOSKELETAL
ENDOCRINE
HEMATOLOGIC
GASTROINTESTINAL
CUTANEOUS
Developmental delay is universal (Tables 76-5 and 76-6; Fig. 76-10). Cognitive impairment does not uniformly affect all areas of development. Social development is relatively spared, but children with Down syndrome have considerable difficulty using expressive language. Understanding these individual developmental strengths will maximize the educational process for children with Down syndrome. Persons with Down syndrome often benefit from programs aimed at stimulation, development, and education. These programs are most effective in addressing social skills that often appear advanced for the intellectual delay. Children with Down syndrome also benefit from anticipatory guidance, which establishes the protocol for screening, evaluation, and care for patients with genetic syndromes and chronic disorders (Table 76-7).

Full access? Get Clinical Tree
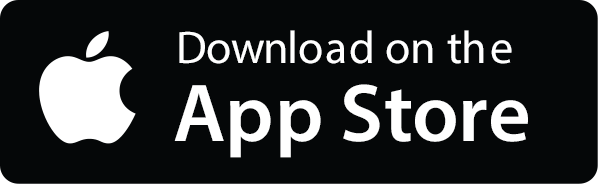
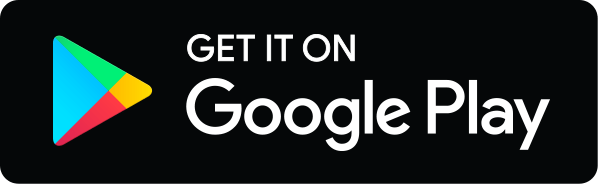