Critical illness in pregnancy is relatively rare. Current studies estimate the incidence of intensive care unit (ICU) admissions in pregnancy and the puerperium to range between 0.7 and 13.5 events per 1000 deliveries (Pollock, 2010). Most of these admissions are postpartum, and obstetric complications account for between 55 and 90 percent. The most common indications are hypertensive disorders of pregnancy, hemorrhage, and sepsis (Baskett, 2008; Chantry, 2008; Orsini, 2012; Pollock, 2010). Nonobstetric indications for ICU admission include maternal cardiovascular disease, pulmonary disease, cerebrovascular accidents, trauma, and anesthetic complications (Wanderer, 2013; Zwart, 2010).
This chapter provides an overview of the most commonly seen conditions in the critically ill pregnant and postpartum woman. Moreover, some less common disorders that an obstetrician would be expected to be familiar with will also be briefly presented.
To understand maternal mortality rates, an understanding of the terms used to report maternal deaths is essential. The International Code of Diseases (ICD-10) and the World Health Organization (WHO) (2010) define maternal death as “the death of a woman while pregnant or within 42 days of termination of pregnancy, irrespective of the duration and site of the pregnancy, from any cause related to or aggravated by pregnancy or its management, but not from incidental or accidental causes.” A pregnancy-related death is defined as “the death of a woman while pregnant or within 1 year of termination of pregnancy irrespective of the duration or site of the pregnancy from complications of pregnancy, a chain of events initiated by pregnancy, or aggravation of an unrelated event or condition by the physiologic effects of pregnancy.” Of other terms, the maternal mortality ratio (MMR) is the number of maternal deaths per 100,000 live births. The pregnancy-related mortality ratio is defined as the number of pregnancy-related deaths per 100,000 live births.
Globally, maternal mortality rates have been decreasing by 1.3 percent per year since 1990 (Kassebaum, 2014). In 2013, the global MMR was 209 deaths per 100,000 live births. This number was lowest—12.1—in the developed world. The highest ratio was seen in Western sub-Saharan Africa, where the MMR was 468.9. Globally, obstetric causes such as hemorrhage, hypertension, and sepsis were responsible for 72 percent of maternal deaths. Other indirect causes such as human immunodeficiency virus (HIV) and other preexisting conditions were responsible for 28 percent (Say, 2014).
In the United States, the pregnancy-related mortality ratio in 2013 was 18.5 deaths per 100,000 live births (Kassebaum, 2014). Despite a significant decline in the maternal mortality rate during the 20th century, the pregnancy-related mortality ratio has climbed since 1987, when the ratio was 7.2 (Berg, 1996; Creanga, 2015). It is unclear if this is a true increase in pregnancy-related mortality rates or whether this rise reflects improved ascertainment of cases. Examples include changes to death certificates and to insurance coding. Likely, it is from a combination of factors.
Unfortunately, a large racial disparity persists in pregnancy-related mortality rates in the United States. The pregnancy-related mortality ratio for black women is more than three times greater than that for white women—38.9 versus 12.0 deaths per 100,000 live births, respectively (Creanga, 2015).
Of specific etiologies, the leading causes of maternal mortality in 2010 were cardiovascular disease (14.6 percent), infection (13.6 percent), noncardiovascular medical conditions (12.7 percent), cardiomyopathy (11.8 percent), and hemorrhage (11.4 percent) (Creanga, 2015). As can be seen in Figure 7-1, maternal mortality rates from hemorrhage and hypertensive disorders of pregnancy have significantly declined. In contrast, deaths from cardiovascular disease have steadily risen.
Several other parameters are noteworthy. First, pregnancy-related mortality ratios increase with increasing maternal age. Second, according to Creanga and colleagues (2015), most deaths occur on the day of delivery or pregnancy termination (16 percent), within 1 to 6 days (21 percent), or from 17 to 42 days (26 percent). Only 23 percent of deaths occurred antepartum and the remaining 14 percent after 42 days. Last, pregnancy-related deaths may complicate ectopic pregnancy, spontaneous abortion, or induced abortion. Between 2006 and 2010, approximately 6 percent of deaths were attributed to these failed pregnancies.
ICU admission rates appear to be similar between the developing world and the developed world. However, the maternal mortality rate associated with these admissions is significantly higher in the developing world—median 14 versus 3.4 percent (Pollock, 2010).
To predict survival, many different scoring systems have been created for individuals admitted to the ICU. These systems include the Acute Physiology and Chronic Health Evaluation (APACHE) and the Simplified Acute Physiology Score (SAPS). These grading schemes were developed in nonpregnant ICU patients. Thus, they do not account for the physiologic changes of pregnancy or the self-limited nature of many obstetric complications such as preeclampsia. When the APACHE and SAPS scores are applied to a pregnant ICU population, the risk of mortality and the severity of illness are significantly overestimated (Gilbert, 2003; Stevens, 2006; Vasquez, 2007).
In critically ill pregnant women with severe sepsis or septic shock, other scoring classifications such as the Modified Early Warning Score (MEWS) and Systemic Inflammatory Response Syndrome (SIRS) criteria have been applied in an attempt to predict disease severity. However, the normal physiologic parameters of pregnancy overlap significantly with these scores, making them unreliable for predicting adverse events in a critically ill pregnant cohort (Bauer, 2014; Edwards, 2015). Thus, additional data are needed to accurately predict risk of ICU admission, disease severity, and mortality risk in the obstetric population.
Recently, a scoring system specific to critically ill gravidas has been proposed to predict illness severity. The Sepsis in Obstetrics Score (SOS), shown in Table 7-1, collects data such as maternal temperature, pulse, and blood pressure to generate a score. Patients with a score ≥6—out of a possible 28—have been reliably identified as being at high risk for ICU admission (Albright, 2014). Although promising, the SOS score requires future prospective validation.
Variable | High Abnormal Range | Normal | Low Abnormal Range | ||||||
---|---|---|---|---|---|---|---|---|---|
Score | +4 | +3 | +2 | +1 | 0 | +1 | +2 | +3 | +4 |
Temperature (°C) | >40.0 | 39–40.9 | 38.5–38.9 | 36–38.4 | 34–35.9 | 32–33.9 | 30–31.9 | <30 | |
SBP (mm Hg) | >90 | 70–90 | <70 | ||||||
Heart rate (bpm) | >179 | 150–179 | 130–149 | 120–129 | ≤119 | ||||
Respiratory ratea | >49 | 35–49 | 25–34 | 12–24 | 10–11 | 6–9 | ≤5 | ||
Spo2 (%) | ≥92 | 90–91 | 85–89 | <85 | |||||
WBC (per μL) | >39.9 | 25–39.9 | 17–24.9 | 5.7–16.9 | 3–5.6 | 1–2.9 | <1 | ||
Immature neutrophils (%) | ≥10 | <10 | |||||||
Lactic acid (mmol/L) | ≥4 | <4 |
Sepsis develops in approximately 1 of every 3500 hospitalizations for delivery in the United States. It is the leading cause of direct maternal deaths in the United Kingdom (Bauer, 2013; Cantwell, 2011). Unlike in the general population, infections in pregnant and postpartum women tend to be polymicrobial from organisms that compose the normal vaginal flora (Barton, 2012). Chorioamnionitis, endometritis, pneumonia, and pyelonephritis are the most frequent serious infections (Bauer, 2013). The most common organisms include Escherichia coli; group A and B streptococci; Staphylococcus aureus—both methicillin-sensitive and methicillin-resistant; Streptococcus pneumoniae; and various gram-negative rods (Acosta, 2014; Bauer, 2013; Knowles, 2015). Infections resulting from Group A β-hemolytic streptococcus and E coli are among those most commonly associated with maternal death (Acosta, 2014; Kramer, 2009; Mabie, 1997).
In 1992, the American College of Chest Physicians and the Society of Critical Care Medicine (ACCP-SCCM) introduced definitions for sepsis-related disorders. These have been generally adopted and used in practice by clinicians and investigators (Bone, 1992; Levy, 2003). SIRS describes an inflammatory response that may be due to several etiologies including infection, trauma, or burns.
The criteria for SIRS are shown in Table 7-2. A woman meets criteria for sepsis if she has two or more SIRS criteria and a known or suspected infection. True sepsis is a continuum that progresses to severe sepsis with the development of organ dysfunction, hypoperfusion abnormality, or sepsis-induced hypotension. Septic shock is a subset of severe sepsis defined as sepsis-induced hypotension that persists despite adequate fluid resuscitation and that is accompanied by hypoperfusion abnormalities or by organ dysfunction. Hypoperfusion abnormalities include, but are not limited to, lactic acidosis, oliguria, or an acutely altered mental status (Barton, 2012; Bone, 1992).
Two or more of the following: Body temperature >38°C or <36°C Heart rate >90 beats per minute Tachypnea, manifested by a respiratory rate >20 breaths/minute or by a Paco2 <32 mm Hg Alteration in the WBC, such as a count >12,000/mm3, or a count <4000/mm3, or the presence of more than 10% immature neutrophils. |
The Surviving Sepsis Campaign has established guidelines for sepsis management in nonpregnant patients. In 2012, updated guidelines for diagnosis and management of severe sepsis and septic shock for the general population were issued (Dellinger, 2013). These guidelines were formed by a consensus committee of 68 experts representing 30 international organizations. This chapter selectively addresses some of the important considerations in management of sepsis in pregnancy.
Since the introduction of this definition of sepsis, the Surviving Sepsis Campaign has set forth extended specifications for the diagnosis of sepsis to improve diagnostic accuracy. Table 7-3 shows these extended diagnostic measures. The Campaign has also set forth criteria for the diagnosis of severe sepsis, which are shown in Table 7-4.
General variables Fever (>38.3°C) Hypothermia (core temperature <36°C) Heart rate >90/min or more than two SD above the normal value for age Tachypnea Altered mental status Significant edema or positive fluid balance (>20 mL/kg over 24 hr) Hyperglycemia (plasma glucose >140 mg/dL or 7.7 mmol/L) in the absence of diabetes |
Inflammatory variables Leukocytosis (WBC count >12,000/μL) Leukopenia (WBC count <4000/μL) Normal WBC count with >10 percent immature forms Plasma C-reactive protein >2 SD above the normal value Plasma procalcitonin >2 SD above the normal value |
Hemodynamic variables Arterial hypotension (SBP <90 mm Hg, MAP <70 mm Hg, or an SBP decrease >40 mm Hg in adults or <2 SD below normal for age) |
Organ dysfunction variables Arterial hypoxemia (Pao2/Fio2<300) Acute oliguria (urine output <0.5 mL/kg/hr for at least 2 hr despite adequate fluid resuscitation) Creatinine increase >0.5 mg/dL or 44.2 μmol/L Coagulation abnormalities (INR >1.5 or aPTT >60 sec) Ileus (absent bowel sounds) Thrombocytopenia (platelet count <100,000/μL) Hyperbilirubinemia (plasma total bilirubin >4 mg/dL or 70 μmol/L) |
Tissue perfusion variables Hyperlactatemia (>1 mmol/L) Decreased capillary refill or mottling |
Any of the following thought to be due to infection: Sepsis-induced hypotension Lactate above upper limits of laboratory normal Urine output <0.5 mL/kg/hr for more than 2 hr despite adequate fluid resuscitation Acute lung injury with Pao2/Fio2 <250 in the absence of pneumonia as infection source Acute lung injury with Pao2/Fio2 <200 in the presence of pneumonia as infection source Creatinine >2.0 mg/dL (176.8 μmol/L) Bilirubin >2 mg/dL (34.2 μmol/L) Platelet count <100,000/μL Coagulopathy (international normalized ratio >1.5) |
The Surviving Sepsis Campaign has developed bundles to be performed within 3 and 6 hours of admission. These bundles focus on timely antibiotic administration and volume status reassessment. Table 7-5 shows the 3-hour and 6-hour sepsis bundles.
Within 3 hr of presentation: Measure lactate level Obtain blood cultures prior to antibiotics Give broad-spectrum antibiotics Give 30 mL/kg fluid bolus of crystalloid for hypotension or lactate ≥4 mmol/L |
Within 6 hr of presentation: If MAP <65 mm Hg after initial fluid resuscitation: Vasopressors to maintain a MAP of ≥65 mm Hg Reassess volume status and tissue perfusion If lactate elevated at 3 hr: Repeat lactate level If initial lactate ≥4 mmol/L reassess volume status and tissue perfusion. |
After initial fluid resuscitation, focused examination: Vital signs Cardiopulmonary status Capillary refill Pulse Skin findings or |
Two or more of the following: Measure CVP Measure Scvo2 Bedside cardiovascular ultrasound Dynamic assessment of fluid responsiveness with passive leg raise or fluid challenge |
This is an immediate concern with severe sepsis. Initial resuscitation begins with crystalloid in a volume of 30 mL/kg. Albumin is used for patients who require substantial amounts of crystalloids. Hetastarch is not recommended (Dellinger, 2015). Vasopressors are implemented for patients who fail to respond to volume resuscitation.
The goals of fluid resuscitation include the following: (1) central venous pressure of 8 to 12 mm Hg; (2) mean arterial pressure >65 mm Hg; (3) urine output of >0.5 mL/kg/hr; and (4) central venous oxygen saturation (Scvo2) or mixed venous oxygen saturation (Svo2) of 70 or 65 percent, respectively.
Intravenous antibiotic therapy is initiated promptly and within the first hour after recognition of septic shock and severe sepsis. In septic shock, each hour delay in administering effective antibiotics is associated with a 7.6-percent rise in the mortality rate (Dellinger, 2013; Kumar, 2006). Blood cultures and site-specific cultures are obtained before antimicrobial therapy is begun as long as doing so does not delay treatment longer than 45 minutes. The patient is prescribed a broad-spectrum antibiotic until agent selection can be refined once cultures are available (Barton, 2012; Pacheco, 2014). In pregnant women, the most likely bacteria are gram-negative rods and group A or group B streptococci. Therefore, antibiotic coverage should include formulations to treat these organisms.
In the nonpregnant population, norepinephrine is recommended as the first-line agent in patients with septic shock not responsive to adequate fluid resuscitation (Barton, 2012; Dellinger, 2013). If norepinephrine and fluid therapy fail to correct hypotension, second-line vasopressors are used. Second-line agents include epinephrine and vasopressin.
Respiratory failure is a rare complication of pregnancy and develops in less than 0.1 percent of births (Chen, 2003). Causes of respiratory failure include pulmonary edema (cardiogenic and noncardiogenic), pneumonia, embolism (pulmonary, amnionic fluid, or venous air), asthma, and acute respiratory distress syndrome (ARDS). Pregnancy-specific causes include amnionic fluid embolism and pulmonary edema due to tocolytics or preeclampsia (Abdel-Razeq, 2011; Mighty, 2010).
Respiratory failure can be categorized as hypoxemic or as hypercapnic. In obstetric patients, hypoxemic respiratory failure is more frequently encountered. It develops when the arterial partial pressure of oxygen is low but the partial pressure of carbon dioxide is normal. This stems from an inability of the lungs to oxygenate blood. The most common cause is a ventilation-perfusion mismatch, which when taken to its most extreme manifestation is called a shunt. Ventilation-perfusion mismatch can develop if some portion of oxygenated blood from the heart does not communicate effectively with the alveoli. Shunts, on the other hand, occur when blood either bypasses the lungs—as in Eisenmenger syndrome—or flows past alveoli that are not ventilated—as with atelectasis. Ventilation-perfusion mismatch often responds to oxygen therapy, whereas a shunt does not. Common causes of hypoxemic respiratory failure include pulmonary embolism, pulmonary edema, aspiration, pneumonia, ARDS, and pneumothorax.
In contrast, hypercapnic respiratory failure results from a failure of ventilation (CO2 exchange) due to airflow obstruction, decreased respiratory drive, or respiratory muscle weakness. This can be seen in patients with severe asthma, drug overdose including magnesium sulfate, and neuromuscular disorders such as myasthenia gravis.
During pregnancy, several physiologic changes involve the respiratory system and can affect management of respiratory failure. First, an increase in the subcostal angle and elevation of the diaphragm leads to decreased chest compliance. Additionally, the rise in intraabdominal pressure and decrease in esophageal sphincter tone results in a greater risk of aspiration. Also, the normal decline in functional residual capacity promotes alveolar collapse, and the physiologic increase in minute ventilation creates a lower partial pressure of carbon dioxide (Pco2). The net sum is respiratory alkalosis with a normal pH, low Pco2, and low HCO3– level. The Pao2 tends to be higher than in a nonpregnant individual, but studies have found that this varies based on altitude (Hankins, 1996). Because the Pco2 is lower in gravidas than in nonpregnant individuals, a “normal” Pco2 can be a sign of inadequate ventilation and should not be considered reassuring (Mighty, 2010). Table 7-6 compares arterial blood gas measurements in pregnant and nonpregnant women.
This syndrome describes severe acute hypoxemic respiratory failure resulting from various pulmonary injuries. Importantly, in women with suspected ARDS, the diagnosis of cardiogenic pulmonary edema should first be excluded, as the treatment approach is very different. In 2012, the diagnostic specifications for ARDS were changed based on recommendations from a consensus conference. The primary conference objectives were to improve the reliability, validity, and feasibility of the ARDS diagnostic parameters. Known as the Berlin criteria, three mutually exclusive categories were created that classified ARDS as mild, moderate, or severe based on the degree of hypoxemia. They define ARDS as respiratory failure “within one week of a known clinical insult or new/worsening respiratory symptoms with bilateral opacities not fully explained by effusions, lobular/lung collapse or nodules. The respiratory failure must not be fully explained by cardiac failure or fluid overload.” An echocardiogram is considered to exclude a cardiogenic cause if the source of ARDS is not clear (ARDS Definition Task Force, 2012; Ferguson, 2012; Mehta, 2015). Definitions of mild, moderate, and severe ARDS are shown in Table 7-7.
Factor | Acute Respiratory Distress Syndrome | ||
---|---|---|---|
Timing | Within 1 week of a known clinical insult or new/worsening respiratory symptoms | ||
Chest imaginga | Bilateral opacities—not fully explained by effusions, lobar/lung collapse, or nodules | ||
Origin of edema | Respiratory failure not fully explained by cardiac failure or fluid overload; need objective assessment (e.g., echocardiography) to exclude hydrostatic edema if no risk factor present | ||
Mild | Moderate | Severe | |
Oxygenationb | 200 <PAO2/Fio2 ≤300</PAO with PEEP or CPAP ≥5 cm H2O | 100 <PAO2/Fio2 ≤200</PAO with PEEP ≥5 cm H2O | Pao2/Fio2 ≤100 with PEEP ≥5 cm H2O |
Antepartum or postpartum acute respiratory failure is ideally managed in conjunction with an intensive care expert and maternal-fetal medicine specialist. Treatment goals strive to restore ventilation and oxygenation. In a woman who is able to protect her airway and has only mild respiratory distress, supplemental oxygen may be the sole requirement. As shown in Table 7-8, options for noninvasive oxygen supplementation include nasal cannula, various types of face masks, continuous positive airway pressure (CPAP), and noninvasive positive-pressure ventilation (NPPV). NPPV differs from CPAP in that it assists with ventilation as well as provides intermittent positive airway pressure (Gregoretti, 2015).
Intubation is indicated in those with hypercapnic or hypoxemic respiratory failure that does not respond adequately to supplemental oxygen, as well as in those who cannot protect their airway. The indications are generally the same as those in nonpregnant individuals. Importantly, as noted earlier, normal Pco2 values are lower in gravidas, and thus intubation is indicated at a lower Pco2.
Tools and technique for intubation in the gravida are described in Chapter 19 (p. 311). Failed intubation occurs approximately eight times more often in a pregnant woman because of anatomic changes to the airway. Because of this, intubation is best performed by the most skilled person (Guntupalli, 2015). Moreover, those with potentially difficult intubations are ideally identified early. As discussed and illustrated in Chapter 18 (p. 293), preparations can be made to accommodate the woman with a challenging airway.
Several different ventilator modes can be used for mechanical ventilation. These settings can be categorized as volume-limited modes, pressure-limited modes, or a combination of the two. In volume-limited modes, the tidal volume is preset, and the ventilator provides a predetermined tidal volume with each breath. In this mode, the airway pressures are allowed to vary, and this guarantees a minimum minute ventilation. As a reminder, minute ventilation = tidal volume × respiratory rate.
In pressure-limited modes, a preset positive-pressure level is given with each breath, and the tidal volume is allowed to vary. In pressure-targeted modes, the peak airway pressures are limited to reduce the risk for barotrauma. An example of a pressure-targeted mode is pressure support ventilation, which requires the patient to initiate spontaneous breaths. Another is pressure-control mode, in which a respiratory rate is preset (Grossbach, 2011).
In addition, the patient’s ability to take spontaneous breaths can be accommodated. Listed here in order of increasing patient autonomy, modes are controlled mechanical ventilation (CMV), assist control (AC), and intermittent mandatory ventilation (IMV). All of these can be set as either volume limited or pressure limited. With CMV, the patient does not perform any of the work of breathing. Respiratory rate and tidal volume or peak airway pressure are set by the provider. With AC, a minimum respiratory rate is set, but the patient is able to trigger additional ventilator-assisted breaths. Last, with IMV, a minimum respiratory rate is once again predetermined, and the patient is able to initiate additional breaths. However, unlike AC, these breaths are not assisted by the ventilator. Synchronized intermittent mechanical ventilation (SIMV) is a variation of IMV, and breaths are synchronized with patient effort (Grossbach, 2011).
Traditionally, the goal of mechanical ventilation has been to achieve a tidal volume of 10 to 15 mL/kg of ideal body weight. In 2000, the Acute Respiratory Distress Syndrome Network (ARDSNet) published the results of a randomized controlled trial in which patients were randomized to different tidal volume groups. In one cohort, a traditional tidal volume (12 mL/kg) with a peak pressure of 50 cm H2O was compared against a low tidal volume (6 mL/kg) with a peak pressure of 30 cm H2O. Mean peak pressures were 33 ± 8 cm of water in the traditional tidal volume group versus 25 ± 6 cm in the low tidal volume group. Of results, the low tidal volume group had a lower mortality rate—31 versus 40 percent—and more days off the ventilator in the first 28 hospital days—12 versus 10 days.
For women with severe ARDS refractory to mechanical ventilation, options are limited. Of these, extracorporeal membrane oxygenation (ECMO) in this population has shown some success. As an overview, ECMO functions by removing blood, adding O2, and removing CO2 before returning it to the circulation. For patients with ARDS, this is often accomplished by venovenous bypass, in which blood is removed from the inferior vena cava (IVC) and returned to the superior vena cava. For this, the patient must be fully anticoagulated. This method does not provide any hemodynamic support. In patients with cardiac failure, venoarterial bypass can be used. In this form of ECMO, blood is removed from the venous system, oxygenated, and then returned to the arterial system, bypassing the heart and lungs. This form of ECMO is associated with a much higher complication rate.
Several studies have found that referral to an ECMO center for patients with severe ARDS results in lower mortality rates and improved outcomes (Duarte, 2014). However, data on the use of ECMO in pregnancy are limited. In one series of 12 pregnant or postpartum women in Australia and New Zealand treated with ECMO during the H1N1 influenza outbreak, 66 percent of patients survived. Of the four deaths, three were from bleeding, which was intracranial, pulmonary, or generalized (Nair, 2011).
Amnionic fluid embolism is a rare but often catastrophic complication of pregnancy. Accurate data on prognosis after this event are difficult to determine due to the varying methods of case ascertainment. Maternal mortality rate estimates currently range from 20 to 60 percent (Clark, 2014). Between 2006 and 2010, amnionic fluid embolism was responsible for 5.3 percent of pregnancy-related deaths in the United States (Creanga, 2015).
Clinical characteristics include acute hypoxia, hypotension, and coagulopathy during labor, delivery, or within 30 minutes of delivery. Risk factors include induction of labor, cervical laceration, placenta previa, placental abruption, advanced maternal age, and cesarean delivery (Ballinger, 2015; Clark, 2014; Knight, 2012).
The syndrome is a clinical diagnosis and assigned once other potential causes have been excluded. The presence of fetal squamous cells and debris in the pulmonary circulation is nonspecific and is not pathognomonic for amnionic fluid embolism. Studies of women with pulmonary artery catheters suggest that squamous cells may be a normal finding in the maternal pulmonary circulation (Clark, 1986; Lee, 1986).
The pathophysiology of amnionic fluid embolism is poorly understood. Current theories implicate an immunologic response to an unknown factor in amnionic fluid, fetal cells, or placental cells that enters the maternal circulation. The clinical response resembles the response observed with septic shock and anaphylactic shock, which supports a potential immunologic basis (Clark, 1995).
There is no definitive treatment, and management is supportive. Ventilatory support and oxygenation, volume resuscitation, vasopressor support, and correction of coagulopathy with transfusion of red cells and clotting factors remain the primary approach. If the woman remains undelivered, then perimortem cesarean delivery may be indicated if cardiac arrest occurs (p. 106).
Obstetric hemorrhage accounted for 11.4 percent of maternal deaths in the United States from 2006 to 2010 and is responsible for a significant portion of ICU admissions in obstetric patients (Creanga, 2015; Wanderer, 2013; Zwart, 2010). Importantly, as many as 90 percent of these deaths may be preventable (Berg, 2005). As described in Chapter 29 (p. 466), obstetric hemorrhage can be defined by several parameters. Three that are often used include blood loss >500 mL after a vaginal delivery and >1000 mL after cesarean delivery; need for blood transfusion; or >10 percent drop in hematocrit (American College of Obstetricians and Gynecologists, 2015b). Due to the physiologic changes of pregnancy, hemodynamic response to hemorrhage may be subtle until 25 to 30 percent of the circulating blood volume has been lost (~1500 to 1800 mL).
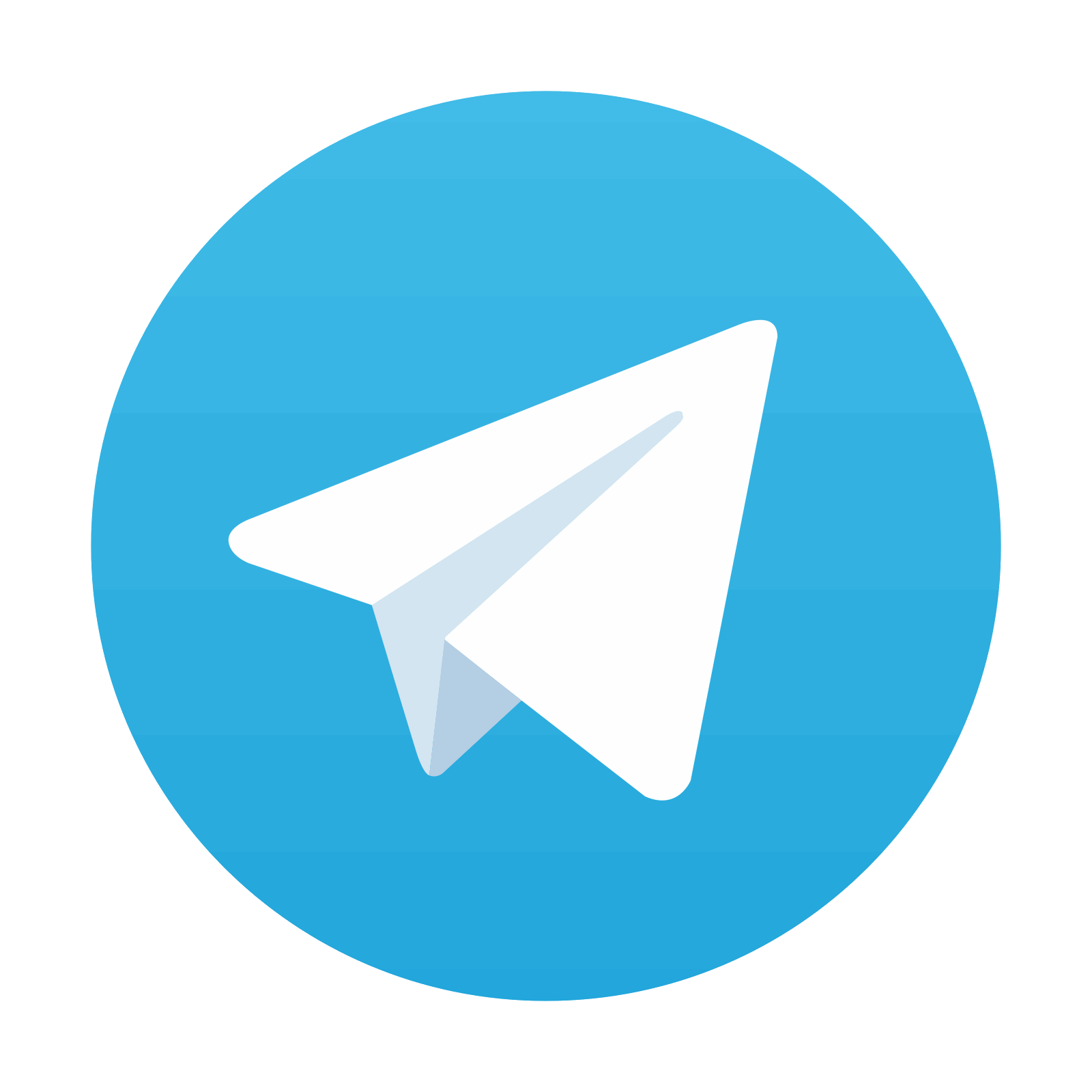
Stay updated, free articles. Join our Telegram channel

Full access? Get Clinical Tree
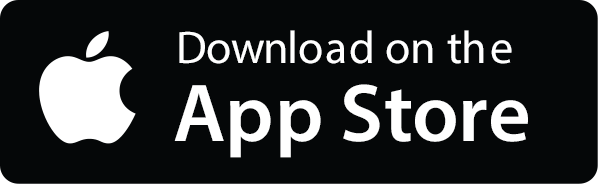
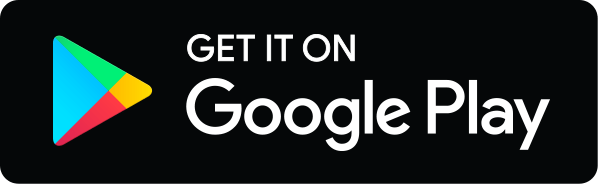