Fig. 1
(a) Illustration showing the ossification centers and synchondroses of the atlas. The neural arch ossification centers form during the seventh gestational week, whereas the ossification center within the body of C1 becomes visible during the first year of life. The posterior midline synchondrosis fuses at about the third year of life, while the neurocentral synchondroses about the atlas body fuse around the age of 7 years. (b) Correlative axial CT from a 24-week-old child (Used with permission from Barrow Neurological Institute)
The axis is unique because two additional ossification centers are present and fuse in the midline to form the odontoid process. The body of C2 fuses to the odontoid between ages 3 and 6 years. The fusion line is often visible until the age of 11 years and remains visible throughout life in one-third of the population [27]. The C2 posterior arches fuse in the midline by age 2–3 years and fuse with the vertebral body by age 3–6 years. Secondary ossification centers appear at the apex of the odontoid, fusing with the dens around age 12 years, and at the inferior end plate of C2, fusing with the vertebral body by age 25 years (Fig. 2) [56].
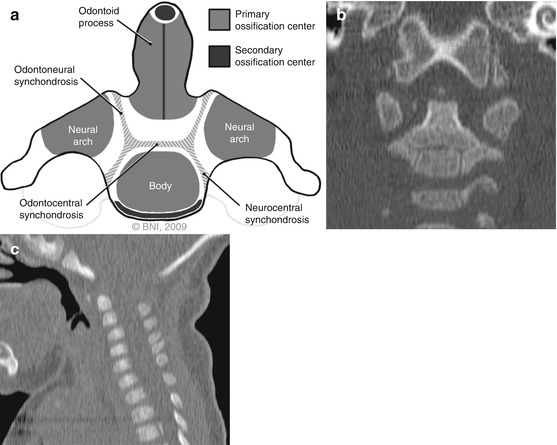
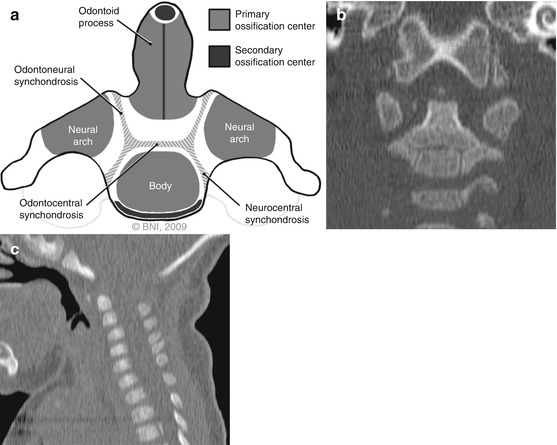
Fig. 2
(a) Illustration showing the ossification centers and synchondroses of the axis. Two ossification centers fuse in the midline to form the odontoid process by the seventh gestational month. The body of C2 fuses to the odontoid between ages 3 and 6 years. The neurocentral synchondroses also fuse between ages 3 and 6 years. The secondary ossification center at the apex of the odontoid appears between ages 6 and 8 years and fuses with the dens around the age of 12 years. (b) Correlative coronal CT from a 6-month-old child shows the synchondrosis between the odontoid and body of C2, the neurocentral synchondroses, and an early apical ossification center for the odontoid. (c) Correlative sagittal CT from a 24-week-old child shows the synchondrosis between the odontoid and body of C2. Note the absence of an apical ossification center (Used with permission from Barrow Neurological Institute)
Imaging Characteristics of the Immature Craniovertebral Junction
Imaging findings of the immature craniovertebral junction can be confused for pathologic conditions, secondary to incomplete ossification and physiologic hypermobility in this region. The atlantodens interval (ADI), for example, should be <5 mm in the pediatric cervical spine [96], compared with the <3 mm guideline for the adult population. This exaggeration in the ADI may reflect incomplete ossification of the dens and laxity of the transverse ligament. Overriding of the arch of C1 on the dens during extension can also be mistaken for atlantoaxial instability, but this finding is normal in 20 % of children under 8 years old [11].
Physiologic C1 lateral mass displacement in the pediatric population may also be exaggerated. Up to 6 mm of C1 lateral mass displacement is common in children <4 years of age, and it may be present until the age of 7 years [47, 83]. A C1 lateral mass displacement >6.9 mm on open-mouth views may indicate transverse ligament disruption in adults, although magnetic resonance imaging (MRI) has demonstrated that the sensitivity of this technique is low [16, 79].
Synchondroses in the pediatric population can be mistaken for fracture lines, whereas fractures through synchondroses can be misinterpreted as within normal limits. The dens-C2 body synchondrosis can be mistaken for a type II dens fracture in children, but this synchondrosis is well corticated and lies below the level of the superior facets of C2. Conversely, a fracture through the dens-C2 body synchondrosis can be missed in pediatric patients [75], despite it being the most common injury to involve the odontoid process in children <7 years old [4, 73]. C1 synchondroses also can be misinterpreted as fractures or sites of abnormal separation [41].
Biomechanical Considerations in the Developing Craniovertebral Junction
The pediatric craniovertebral junction demonstrates an age-dependent hypermobility resulting from underdeveloped bony anatomy, ligaments, and musculature. Furthermore, a child’s proportionally larger head enables a larger moment arm to act on the underdeveloped spine. Biomechanical studies of the pediatric craniovertebral junction and upper cervical spine, however, are rare in comparison with the extensive published studies on biomechanics in the adult population.
Large series demonstrate that the occipitoatlantoaxial complex appears especially vulnerable in the young-age group (0–8 years), with a tendency toward pure ligamentous injuries rather than fractures [23, 65]. The craniovertebral junction is vulnerable in young children for several reasons:
1.
The occipital condyles are smaller.
2.
3.
4.
The odontoid synchondrosis is susceptible to translational forces.
Because of these unique characteristics of the child’s craniovertebral junction, care for the pediatric trauma patient must be provided with a high index of suspicion for injury to this region.
Patient Presentation and Evaluation
Presentation and Prehospital Immobilization
In pediatric patients with potential craniovertebral junction injury, immediate goals in the field are to establish an airway, obtain adequate ventilation, and provide cardiovascular support. Because considerable force is required to cause significant craniovertebral junction injury, patients may present with head, spinal cord, or multisystem traumatic injuries. Vertebral artery injury or cranial nerve deficits may be present. However, some patients with craniovertebral injury may have no neurologic deficits [38].
The primary goal of immediate spinal immobilization is to prevent further injury. Immobilization should be accomplished with the child’s cervical spine in the neutral position. In children less than 8 years of age, consideration must be given to the relatively large head compared to the torso, which forces the neck into flexion when the child is placed supine on a flat surface. Of 40 children less than 8 years of age assessed by Nypaver and Treloar, all required elevation of the torso to achieve neutral alignment [54]. A cervical collar did not prevent the induced flexion when a flat spinal board was used [86]. Authors have therefore recommended either elevating the torso or placing the head in an occipital recess to achieve neutral position during immobilization of a pediatric patient [37, 54]. If occipitoatlantal dislocation is suspected, sandbags and tape should be used for initial immobilization of the head. Rigid cervical collars can lead to further distraction of the occipitoatlantal joint [18].
Clinical Clearance of the Cervical Spine
After the pediatric trauma victim has been immobilized and transported to an acute care facility, and following the initial clinical assessment and provision of cardiorespiratory support, evaluation of the cervical spine may begin. After a cervical collar has been placed on a child, formal clearance must be obtained before it can be removed. Certain low-risk children may not need imaging to exclude cervical spine injury [44, 90]. In 268 children with isolated head injuries, Laham et al. investigated the role of cervical 3-view (anteroposterior, lateral, and open mouth) spine x-rays [44]. Patients with isolated head injuries who were able to communicate and who had no neck pain or neurologic deficits were retrospectively classified as low risk (n = 135). High-risk patients were those incapable of verbal communication, because of either age (<2 years) or head injury, and those with neck pain (n = 133). After radiographic evaluation, no injuries were found in the low-risk group, but 10 injuries were discovered in the high-risk group. The authors concluded that cervical spine radiographs are not necessary in children with isolated head injuries who can communicate and have no neck pain or neurologic deficit [44].
Consistent with these results, Vicellio et al. prospectively evaluated the cervical spines of 3,065 children less than 18 years of age [90]. They identified 603 low-risk children, defined as those with the absence of each of the following criteria: midline cervical tenderness, evidence of intoxication, altered level of alertness or intubation, focal neurologic deficits, and painful distracting injury. After undergoing a three-view radiographic evaluation, none of these low-risk children had a documented cervical spine injury. The authors concluded that the collar may be clinically cleared without further imaging in patients meeting these low-risk criteria.
Imaging
Plain radiography has been used as the first imaging modality for the upper cervical spine, and pediatric patients who do not meet the above low-risk criteria should undergo at least anteroposterior and lateral x-rays. The utility of open-mouth odontoid views in pediatric trauma victims has been questioned [8, 84]. In young children, Swischuk et al. identified missed fractures on lateral views that were detected on open-mouth views at a rate of 0.007 per year per radiologist. They concluded that open-mouth view radiographs may not be needed routinely in young children (age <5 years) [84]. Buhs et al. found that open-mouth radiographs did not provide the diagnosis in any of 15 children less than 9 years of age who sustained cervical spinal injuries [8]. They concluded that open-mouth view radiographs are not necessary in children <9 years old.
Computed tomography (CT) provides superior detail of bony anatomy in comparison with plain radiography, but the role of CT in evaluating the cervical spines of pediatric trauma patients has not been well studied. Hutchings et al. retrospectively reviewed cervical spine clearance methods in 115 consecutive obtunded trauma patients <16 years old [40]. Both the sensitivity and specificity of CT for injury detection were 100 %, although a low incidence of injury in this population makes interpretation of these results difficult. When obtaining a CT, it is important to consider that most children younger than 10 years old with cervical spine injuries will have ligamentous injuries without a fracture [19, 34]. In older children with cervical spine injuries, the incidence of a ligamentous injury without fracture (20 %) is still significant, although less than the incidence of fracture (80 %) in this population [25, 90]. Therefore, normal osseous anatomy on CT imaging alone should not be used to exclude injury to the pediatric cervical spine.
As proposed by Pang, the condyle-C1 interval (CCI) as determined on CT is a sensitive diagnostic measurement of occipitoatlantal dislocation [60, 61]. Pang et al. analyzed the CCI from sagittal and coronal CT images of the craniovertebral junction from 89 children without occipitoatlantal dislocation and 16 children with occipitoatlantal dislocation. The abnormal CCI varied from 5 to 34 mm, and eight patients showed left-right joint asymmetry. Compared to “standard” tests on plain films with a sensitivity that ranged between 25 and 50 % and a specificity that ranged between 10 and 60 %, the CCI of 4 mm or greater had a sensitivity and specificity of 100 %. They concluded that the CCI criterion has the highest diagnostic sensitivity and specificity for occipitoatlantal dislocation among all radiographic methods [60].
MRI is superior to CT in delineating nonosseous anatomy. It can provide important information about ligamentous injury and influence surgical management. Keiper et al. reviewed their experience with MRI in the evaluation of children with clinical evidence of cervical spine trauma and no evidence of fracture on plain radiographs or CT, but who had persistent or delayed symptoms or instability [42]. In 52 children, 16 MRI examinations were abnormal. Of these 52 children, 4 underwent surgical treatment, and in each of these surgical cases, the MRI findings influenced the surgeon to stabilize more levels than would have otherwise been addressed. MRI can also provide prognostic information regarding existing neurological deficits. Davis et al. used MRI to evaluate pediatric spinal cord injury in 15 patients [15]. They found that MRI findings correlated with neurological outcome; hematomyelia was associated with permanent neurologic deficit [15].
We use MRI whenever a neurologic deficit is present and to assess the extent of ligamentous involvement of craniovertebral junction injuries. We have a low threshold for obtaining MRIs of the cervical spine in obtunded children with mechanisms of injury that are high risk for injury to the craniovertebral junction. However, MRI is a static test and does not necessarily predict cervical spine instability [39]. The extent of injury demonstrated on MRI can be used to guide management (Fig. 3).
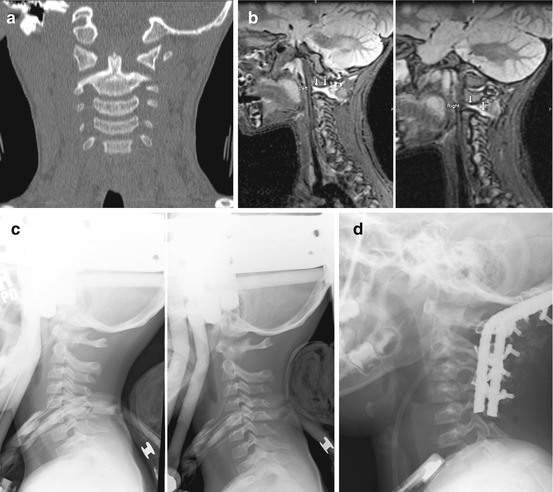
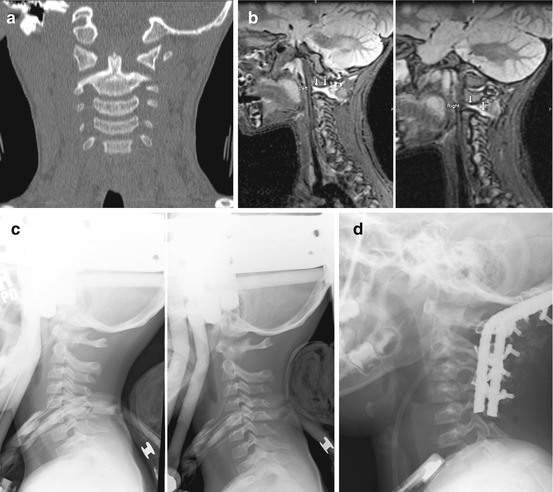
Fig. 3
(a) Coronal CT from a 4-year-old girl who was an unrestrained passenger in a motor vehicle accident, demonstrating widening of the bilateral atlantoaxial joints. (b) Sagittal MRI short T1-weighted inversion recovery (STIR) images show signal changes within the bilateral atlantoaxial joints and within the right occipitoatlantal joint. (c) Halo immobilization was unsuccessful as evidenced by persistent vertical translocation at C1–C2 between upright (left) and supine (right) films. (d) Surgical stabilization was achieved with occiput-to-C4 titanium rod fixation and autograft fusion (Used with permission from Barrow Neurological Institute)
Pediatric Spinal Cord Injury
The upper cervical spinal cord is particularly vulnerable to injury in children <8 years old, secondary to ligamentous injury often with no overt bony fractures [23, 65]. A thorough neurologic examination should be performed to identify the level of injury and to determine whether the injury is complete or incomplete. Sacral sparing should be tested, because it indicates continuity of long tracts and is associated with an improved likelihood for a return of neurologic function [50]. Upper cervical cord injuries may be fatal, and patients who do survive these injuries may be dependent on a respirator.
An acute nonoperative management consideration for pediatric spinal cord injury is maintenance of adequate mean arterial pressure. Hypotension should be avoided, and the mean arterial blood pressure should be elevated to protect spinal cord blood perfusion and to prevent ischemia [87]. Unlike blood pressure management, however, the use of high-dose steroids to treat spinal cord injury is controversial. There are few data on the use of methylprednisolone specific to pediatric patients. The National Acute Spinal Cord Injury study excluded all children younger than 13 years old, and based on the experience in adults, the use of methylprednisolone cannot be recommended [6, 33].
Spinal Cord Injury Without Radiographic Abnormality
The biomechanical properties of the craniovertebral junction in children <8 years old place them at risk for spinal cord injury without radiographic abnormality (SCIWORA) [23]. This syndrome was described by Pang and Wilberger in 1982 [63]. A meta-analysis conducted by Pang found the incidence of SCIWORA in pediatric patients with spinal cord injury to be about 35 % [58]. With more widespread use of MRI and with improvements in imaging quality, the incidence has been reported to be as low as 3 % [2]. The physiologic basis of SCIWORA is the hypermobility of the pediatric cervical spine. Traumatic hyperflexion, hyperextension, or distraction will cause the spine to recoil to its physiologic state, while the spinal cord sustains injury from temporary compression and deformation [58]. Children <8 years old are considerably more likely to sustain more rostral and severe SCIWORA than older children [20].
MRI is the modality of choice for patients with suspected SCIWORA. In the clinical setting of SCIWORA, injuries to extraneural structures as well as to the spinal cord have been documented on MRI. The patient’s MRI examination may also be completely normal. Pang divided MRI findings within the spinal cord of 50 patients with clinical SCIWORA into the following categories: major hemorrhage, minor hemorrhage, edema, and no abnormality [58]. The severity of MRI findings correlated positively with severity of neurologic deficits. Pang also proposed the concept of “occult” instability of the spine in SCIWORA patients [58], which has been postulated to be a cause of delayed neurologic deterioration in these patients [57, 58]. Occult instability is also a possible cause of recurrent SCIWORA, in which minor trauma after an initial SCIWORA episode causes recurrent symptoms [67]. Presumably, weakened extraneural structures may facilitate recurrence, and the injured spinal cord is more vulnerable to repeat trauma.
Therapeutic immobilization of patients with SCIWORA is controversial. Pang and Pollack advocated 12 weeks of immobilization to allow ligamentous injuries to heal and to prevent recurrent SCIWORA [62]. Bosch et al., however, found that immobilization did not prevent SCIWORA recurrence [5]. A meta-analysis by Launay et al. showed that patients immobilized for 8 weeks had a 17 % chance of developing recurrent SCIWORA, but no patients immobilized for 12 weeks demonstrated SCIWORA recurrence [45]. These data suggest that 12 weeks of immobilization is appropriate for most cases of SCIWORA, followed by dynamic films to detect instability.
Neonatal Spinal Cord Injury
Birth injuries of the spinal cord occur in approximately 1 per 60,000 births [90]. The upper cervical spine is the most common level of injury, and neonates present with apnea and flaccid quadriplegia [48, 52]. Menticoglou et al. reported 15 neonates with birth-related upper cervical spinal cord injuries [52]. All were associated with cephalic presentation requiring rotational maneuvers with forceps, and all but one child was apneic at birth with quadriplegia. In another report, Mackinnon et al. described 22 neonates with birth-related spinal cord injuries; 14 of whom had upper cervical injuries [48]. Of the seven infants alive at last follow-up, six were dependent on mechanical ventilation. A thermoplastic molded device spanning the occiput to the thorax has been used for spinal immobilization in these difficult patients [35].
Osseoligamentous Injuries of the Pediatric Craniovertebral Junction
Occipitoatlantal and Atlantoaxial Dislocation
Traumatic occipitoatlantoaxial dislocations (OAAD) were once thought to be rare and predominantly fatal entities [53, 82]. Improvements in on-site resuscitation and transportation have increased the number of patients surviving these injuries [53, 64]. Often seen in young children involved in high-speed motor vehicle accidents, complete neurologic injury at this level usually results in rapid death. Therefore, neurologic deficits associated with OAAD are partial or absent.
In terms of distraction injuries, the occipitoatlantoaxial complex can be regarded as one unit [51]. Because the occipitoatlantoaxial ligaments span the occipital-C1 and the C1–C2 segments, instability should affect both segments. Neither the C0–C1 and C1–C2 joint capsules nor the atlantooccipital and atlantoaxial membranes contribute significantly to the vertical stability of the craniovertebral junction [96]. Rather, the tectorial ligament, alar ligaments, and surrounding musculature appear to have the largest roles in stabilizing this segment [21, 96]. As discussed earlier, younger children are uniquely predisposed to OAAD because their occipital articulation with the lateral masses of C1 is more planar than cuplike and because a young child has a relatively larger head and greater hypermobility of the upper cervical spine compared to older patients [27, 95].
Traynelis et al. classically divided occipitoatlantal dislocation into three types (Fig. 4) [85]. The direction of dislocation of the occiput with respect to the atlas determines the type of injury. Type I injury describes an anterior displacement of the occiput with respect to the atlas. Type II injury is a distraction injury with vertical displacement. Type III injury involves posterior displacement of the occiput.
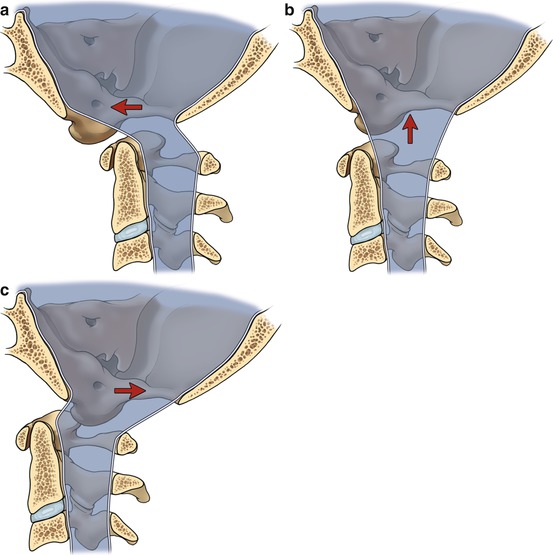
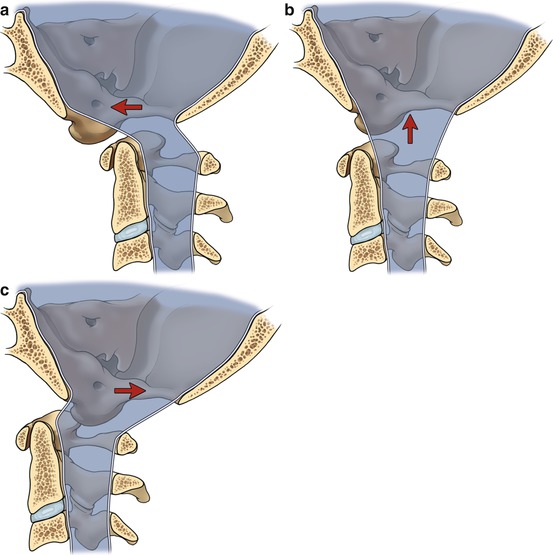
Fig. 4
Drawings representing the classification for occipitoatlantal dislocation. (a) Type I: anterior displacement (arrow) of the occiput with respect to the atlas. (b) Type II: distraction and vertical displacement (arrow). (c) Type III: posterior displacement (arrow) (Used with permission from Barrow Neurological Institute)
The diagnosis of OAAD requires a high index of suspicion, especially in a child involved in a high-speed motor vehicle accident or auto-pedestrian accident. None of the criteria for diagnosis of OAAD are failproof. Classic measurements on plain radiography to assess OAAD include Power’s ratio [68], the basion-dens interval [97], basion-axial interval [36], and atlantodens interval [46]. The accuracy of these traditional radiographic criteria has been questioned. Reconstructed coronal and sagittal CT images can be used to assess the joint-space separation and symmetry at C0–C1 and C1–C2. A condyle-C1 interval (CCI) >4 mm as measured on CT may indicate occipitoatlantal dislocation [30, 60]. MRI can further define abnormalities of joints, ligaments, and soft tissues at C0–C1 and C1–C2. The integrity of the tectorial membrane on MRI may be a critical factor in determining severity of both occipitoatlantal and atlantoaxial joint injuries [82]. MRI also accurately depicts the anatomical integrity of the transverse ligament, which has been proposed as a criterion for early fusion for atlantoaxial instability [17].
Initial management of OAAD involves placement of the child into a halo vest. Traction and a cervical collar should be avoided, because these can lead to overdistraction and worsening of neurologic injury [18]. Definitive treatment of OAAD requires surgical fusion. Threaded contoured rods and wiring can be used from the occiput to C1 or C2, depending on the injury [74]. We have successfully used the rod-and-wire technique in children as young as 11 months [69]. Transarticular screws of C1–C2 or C2 pedicle screws, coupled with plate or rod constructs, have also been used in pediatric patients with excellent success [31, 74]. We have used occipital keel screws coupled with C1 lateral mass screws and C2 pars interarticularis screws to achieve occipitoatlantoaxial stabilization in young pediatric patients (Fig. 5).
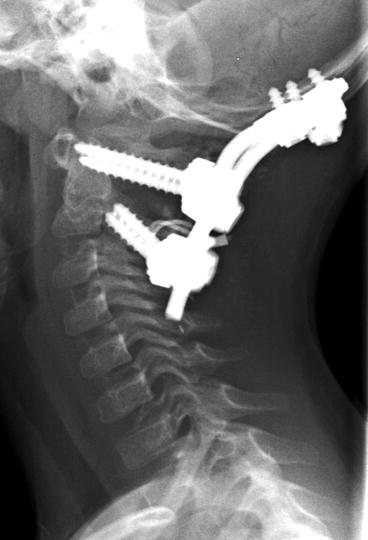
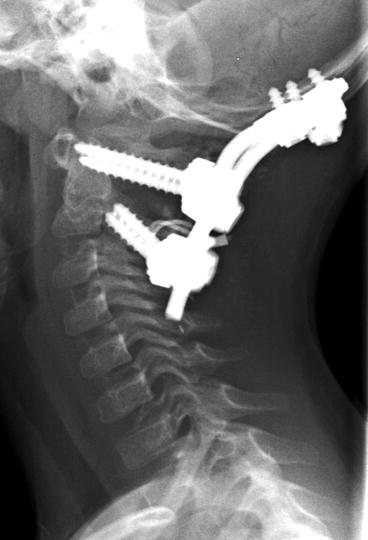
Fig. 5
Postoperative lateral radiograph of a 3-year-old girl with occipitoatlantoaxial dislocation, fixated with occipital keel screws, C1 lateral mass screws, and C2 pars interarticularis screws (Used with permission from Barrow Neurological Institute)
Atlantoaxial Rotatory Subluxation
Atlantoaxial rotatory subluxation is a frequent cause of torticollis in children. If it has persisted for more than 3 months, it is also known as atlantoaxial rotatory fixation. Trauma and upper respiratory infections are the main causes of this disorder, but iatrogenic and congenital causes also exist. The mechanism of injury is often trivial trauma resulting in subluxation of C1 on C2. Children present with a “cock-robin” appearance with the head tilted toward and rotated away from the side of the dislocation. With acute subluxation, a child presents with accompanying neck pain. If fixed persistent deformities are present, the pain often subsides.
Fielding and Hawkins presented the most commonly used classification scheme for these injuries (Fig. 6) [28]. Type I atlantoaxial rotatory injury is defined by an intact transverse ligament. Types II and III are defined by disruption of the transverse ligament alone and by the transverse and alar ligaments, respectively. These injuries are associated with a progressively widened atlantodens interval (ADI). Type IV injury is a posterior rotatory displacement of the atlas on C2 in the setting of odontoid hypoplasia.
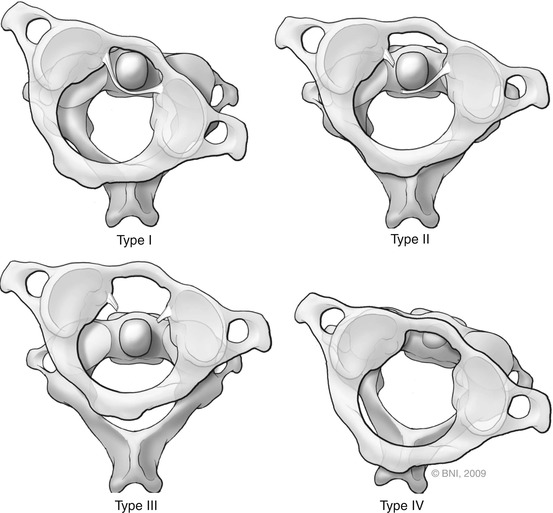
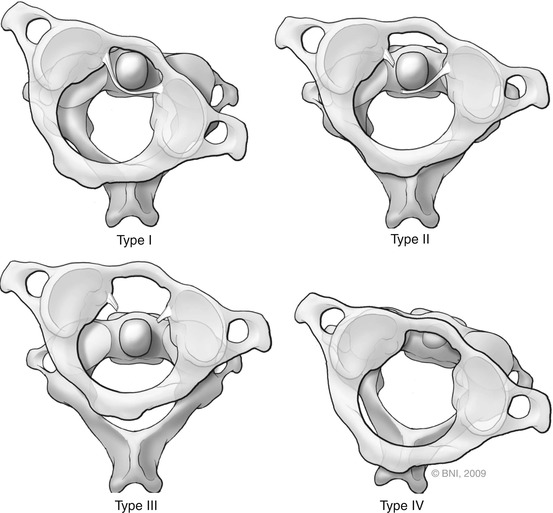
Fig. 6
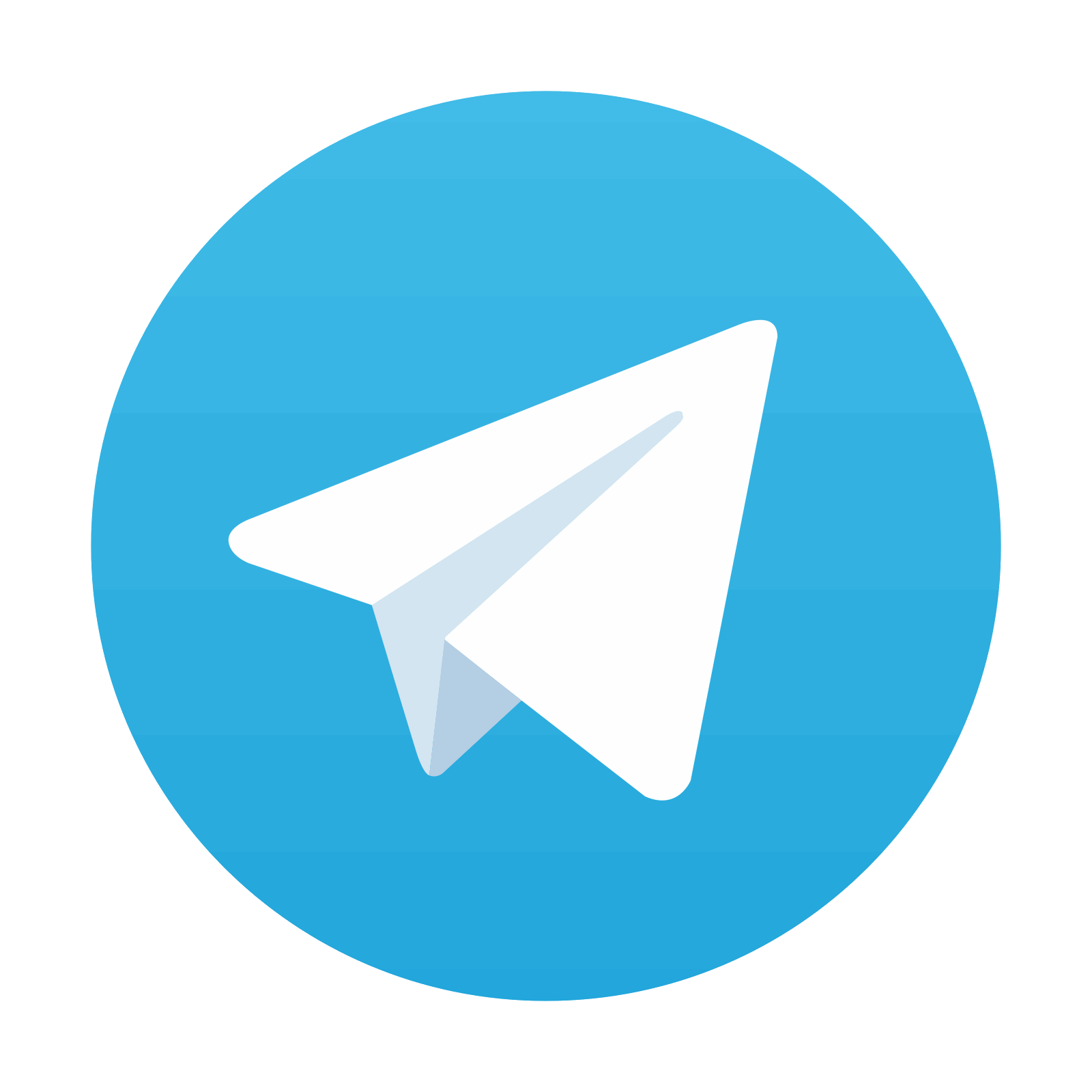
Fielding and Hawkins classification scheme for atlantoaxial rotatory injuries. Type I is defined by an intact transverse ligament. Types II and III are defined by disruption of the transverse ligament alone and the transverse and alar ligaments, respectively. These injuries are associated with a progressively widened atlantodens interval. Type IV injury is a posterior rotatory displacement of the atlas on C2 in the setting of odontoid hypoplasia (Used with permission from Barrow Neurological Institute)
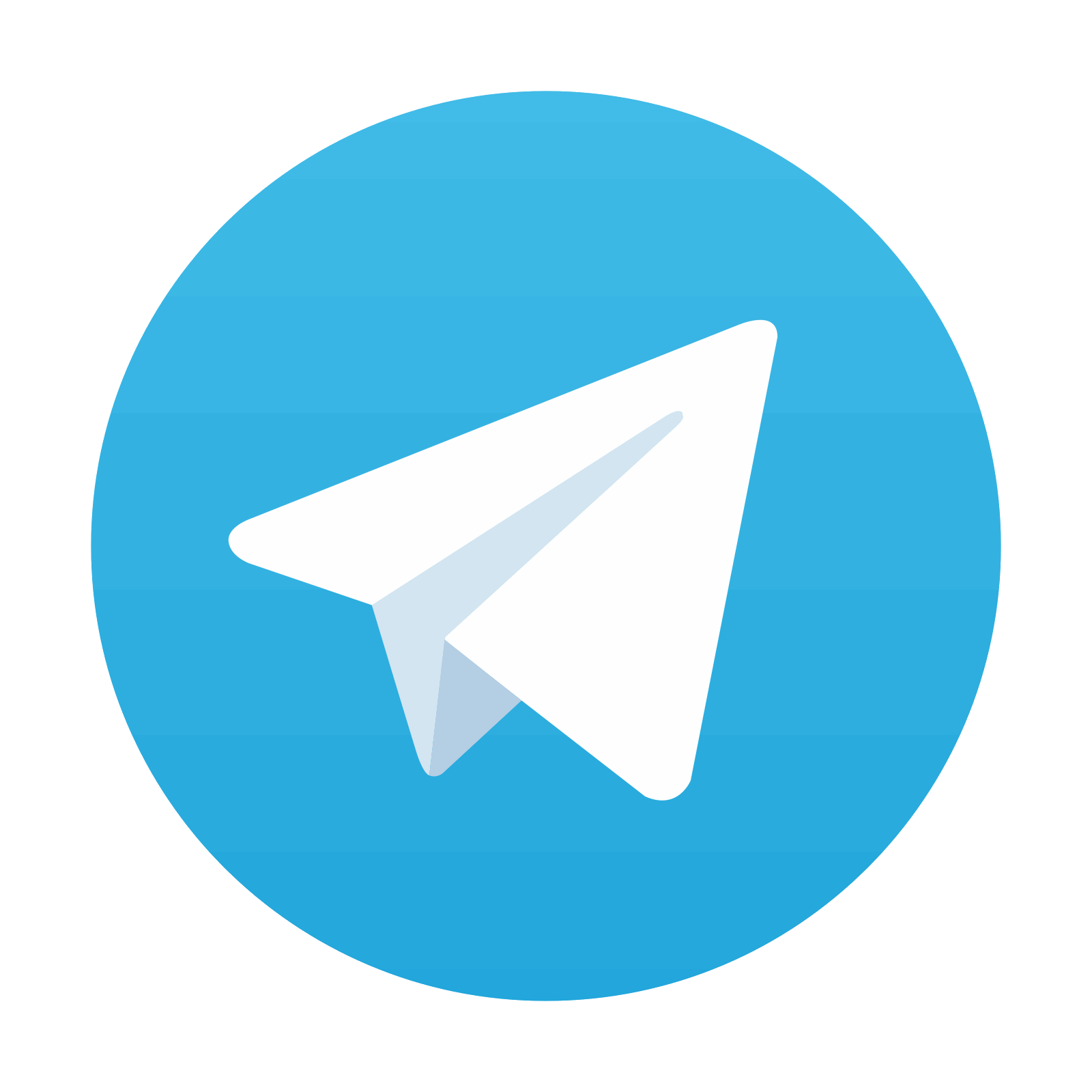
Stay updated, free articles. Join our Telegram channel

Full access? Get Clinical Tree
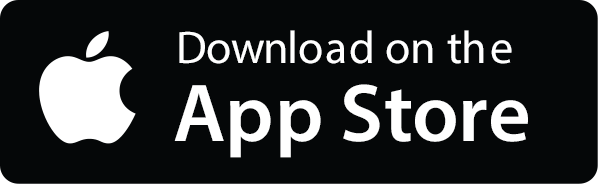
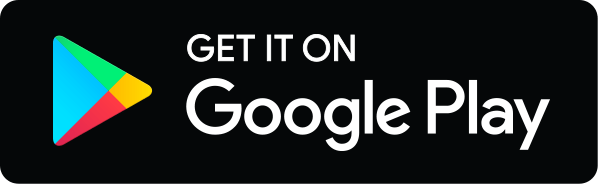
