Continuous Blood Gas Monitoring
M. Kabir Abubakar
Adequate monitoring of oxygenation and acid base status is an important and necessary component in the management of critically ill neonates in the intensive care unit. This provides information that is fundamental to the diagnosis of respiratory and metabolic disorders and to assess the effect of therapeutic interventions. Traditionally, arterial blood gas analysis has been used as the gold standard because it provides data on hydrogen ion content of the blood (pH), arterial oxygen tension (PaO2), partial pressure of carbon dioxide (PaCO2), a calculation of the bicarbonate ion (HCO3), and base deficit. However, arterial blood gas sampling requires invasive procedures and provides only intermittent data. There are now noninvasive methods including pulse oximetry, transcutaneous PO2 and CO2 monitoring, and interesting developments in continuous intra-arterial blood gas analysis (in those patients who already have an indwelling arterial catheter) that supplement traditional intermittent blood gas analysis. These provide real-time continuous dynamic data on the patient’s respiratory status, thus can be used to provide early warning and immediately evaluate the efficacy of clinical interventions in the NICU.
PULSE OXIMETRY
Pulse oximetry has remained the most common method of continuous oxygen monitoring in clinical care. It is noninvasive, easy to use, readily available, and able to provide continuous monitoring of arterial oxygen saturations and pulse rate.
A. Definitions
1. Arterial oxyhemoglobin saturation measured by arterial blood gas analysis is referred to as SaO2.
2. Arterial oxyhemoglobin saturation measured noninvasively by transcutaneous pulse oximetry is referred to as SpO2.
B. Background
1. Principles of oxygen transport
a. Approximately 98% of the oxygen in the blood is bound to hemoglobin.
The amount of oxygen content in the blood is related directly to the amount of hemoglobin in the blood, amount of oxygen bound to the hemoglobin, and to the partial pressure of unbound, dissolved oxygen in the blood (PaO2) (1). The relationship of arterial PO2, in near-term infants, to percent saturation measured by pulse oximetry is shown in Figure 11.1.
b. The relationship between blood PaO2 and the amount of oxygen bound to hemoglobin is presented graphically as an oxygen-hemoglobin affinity curve (Fig. 11.2). Percent oxygen saturation is calculated using the formula

2. Principles of pulse oximetry
a. Based on the principles of spectrophotometric oximetry and plethysmography (2).
b. Arterial saturation and pulse rate are determined by measuring the absorption of selected wavelengths of light.
Oxygenated hemoglobin (oxyhemoglobin) and reduced hemoglobin (deoxyhemoglobin) absorb light as known functions of wavelengths. By measuring the absorption levels at different wavelengths of light, the relative percentages of these two constituents and SpO2 are calculated.
c. A sensor composed of two light-emitting diodes (LEDs) as light sources and one photodetector as a light receiver is employed. The photodetector is an electronic device that produces a current proportional to the incident light intensity (2, 3).
There are two methods of sending light through the measuring site: transmission and reflectance. In the transmission method, the emitter and photodetector are opposite of each other with the measuring site in between. In the reflectance method, the emitter and photodetector are next to each other on the measuring site. The light bounces from the emitter to the detector across the measuring site. The transmission method is the most common type used and is discussed here.
(1) One LED emits red light with an approximate wavelength of 660 nm.
Red light is absorbed selectively by deoxyhemoglobin.
(2) The other LED emits infrared light with an approximate wavelength of 925 nm.
Infrared light is absorbed selectively by oxyhemoglobin.
d. The different absorption of the wavelengths when transmitted through tissue, pulsatile blood, and nonpulsatile blood are utilized (Fig. 11.3).
(1) The photodetector measures the level of light that passes through without being absorbed.
(2) During the absence of pulse (diastole), the detector establishes baseline levels for the wavelength absorption of tissue and nonpulsatile blood.
(3) With each heartbeat, a pulse of oxygenated blood flows to the sensor site.
(4) Absorption during systole of both the red and the infrared light is measured to determine the percentage of oxyhemoglobin.
(5) Because the measurements of the change in absorption are made during a pulse (systole), these pulses are counted and displayed as pulse rate. In addition, the pulse oximeter also displays a plethysmographic waveform which can help clinicians distinguish an artifactual signal from the true signal.
C. Indications
1. Noninvasive continuous or intermittent arterial oxygen saturation and heart rate monitoring
2. To monitor oxygenation in infants suffering from conditions associated with
a. Hypoxia
b. Apnea/hypoventilation
c. Cardiorespiratory disease
d. Bronchopulmonary dysplasia
3. To monitor response to therapy
a. Resuscitation
Pulse oximetry is a necessary adjunct to monitoring in the delivery room. With the use of pulse oximetry, SpO2 values can be obtained within 1 minute after birth (Fig. 11.4) (4, 5, 6, 7, 8)
b. Monitoring effectiveness of positive pressure ventilation or during placement of an endotracheal tube
4. To monitor side effects of other therapy
a. Endotracheal tube suctioning
b. Positioning for laryngoscopy, spinal tap, and other procedures
It is optimal to use pulse oximetry for oxygen monitoring in the very low-birth-weight infant because of its noninvasiveness. Pulse oximetry can be used reliably in very low-birth-weight infants with acute as well as chronic lung disease (9, 10, 11).
6. Pulse oximetry also offers an advantage for precise fraction of inspired oxygen (FiO2) control during neonatal anesthesia because of the short response time to changes in SpO2 (10).
7. Screening tool for critical congenital heart disease and infant car seat testing in the newborn
D. Limitations
1. Decreased accuracy when arterial saturation is <65%.
Pulse oximetry will overestimate SpO2 at this level; therefore, blood gas confirmation is imperative (9, 10, 11).
2. Not a sensitive indicator for hyperoxemia (10).
Pulse oximeter accuracy does not allow for precise estimation of PaO2 at saturations >90%. Small changes in O2 saturation (1% to 2%) may be associated with large changes in PaO2 (6 to 12 mm Hg) (10).
3. Because pulse oximeters rely on pulsatile fluctuations in transmitted light intensity to estimate SpO2, they are all adversely affected by movement (9, 10, 11).
In some cases, the pulse oximeter may calculate an SpO2 value for signals caused by movement, or it may reject the signal and not update the display. Usually, pulse rate output from the oximeter will reflect the detection of nonarterial pulsations, indicating either “0” saturation or “low-quality signal” (3). Advances in microprocessor technology have led to improved signal processing, which makes it possible to minimize motion artifact and monitor saturation more accurately during motion or low-perfusion states (10).
4. Significant levels of carboxyhemoglobin or methemoglobin can yield erroneous readings (carboxyhemoglobin absorbs light at the 660-nm wavelength). However, carboxyhemoglobin levels of <3% will not affect the accuracy of the instrument.
5. SpO2 may be overestimated in darkly pigmented infants although this is not always consistent (10, 11, 12, 13).
6. Erroneous readings can occur in the presence of high fetal hemoglobin (14).
A smaller effect on accuracy is noted when fetal hemoglobin levels are <50% (14). With a predominance of fetal hemoglobin, an SpO2 of >92% may be associated with hyperoxemia (14). However, whereas saturations may appear adequate, PaO2 may be low enough to produce increased pulmonary vascular resistance (SpO2/PaO2 curve shift to the left).
Infants with chronic lung disease and prolonged oxygen dependence are older and have less fetal hemoglobin; therefore, SpO2 readings obtained from these patients may be more accurate than those obtained from neonates with acute respiratory disorders at an earlier age (14). The same situation exists in infants who have undergone exchange transfusion because of decreased levels of fetal hemoglobin.
7. Light sources that can affect performance include surgical lights, xenon lights, bilirubin lamps, fluorescent lights, infrared heating lamps, and direct sunlight.
Although jaundice does not account for variability in pulse oximeter accuracy (15), phototherapy can interfere with accurate monitoring. Therefore, appropriate precautions should be taken, such as covering the probe with a relatively opaque material (1).
8. Do not correlate SpO2 values with laboratory hemoximeters (15).
Most laboratory oximeters measure fractional oxygen saturation (all hemoglobin including dysfunctional
hemoglobin) as opposed to functional oxygen saturation (oxyhemoglobin and deoxyhemoglobin excluding all dysfunctional hemoglobin).
hemoglobin) as opposed to functional oxygen saturation (oxyhemoglobin and deoxyhemoglobin excluding all dysfunctional hemoglobin).
Use of normal adult values for hemoglobin, 2,3-diphosphoglycerate, and, in some cases, PaCO2 can lead to errors in the algorithm used to calculate SaO2 with some blood gas analysis instruments (15).
9. Although pulse oximeters can detect hyperoxemia, it is important that type-specific alarm limits are set to avoid hypo or hyperoxemia (2, 16).
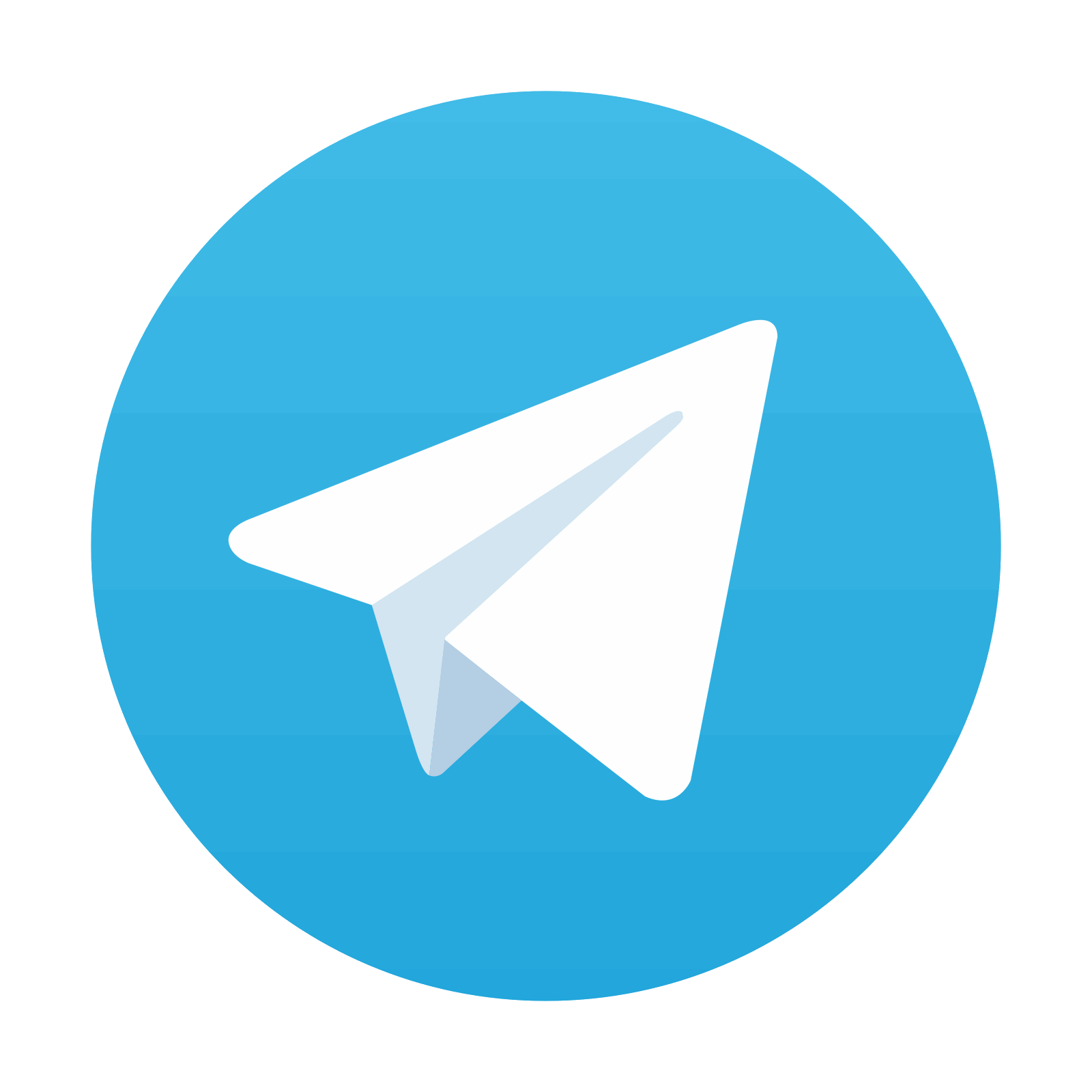
Stay updated, free articles. Join our Telegram channel

Full access? Get Clinical Tree
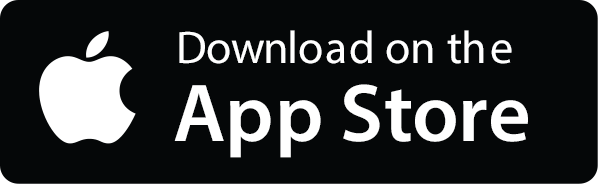
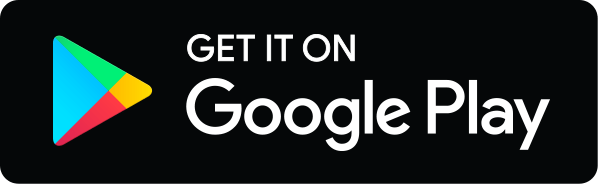