BACKGROUND
Excluding patent ductus arteriosus of the premature infant, congenital heart disease is estimated to occur in about 0.8% of all children.1 Fortunately, most of these problems are minor or self-limited and do not require intervention. Congenital heart disease requiring intervention is less common—about 3 to 4/1000.2 For this important group of patients with heart disease, the past 70 years have witnessed a remarkable advance in diagnostic and therapeutic techniques such that many of these children with previously lethal defects may now lead relatively normal lives. Congenital heart disease ranks ninth in cause of death of infants <1 year at 2.1 per 100,000 live births in the United States, and is comparable to the death rate for sepsis and respiratory distress syndrome.3
Children with congenital heart disease may be hospitalized at the time of diagnosis if they present with critical heart disease, after cardiac interventions (surgery or transcatheter), or for acute illness related or unrelated to their heart condition. While some congenital cardiac disease has straightforward anatomy, the hospitalist may care for children with the most complex cardiac anatomy, as these children typically spend the most time in hospital. The pediatric hospitalist frequently plays an important role in the care of children with congenital heart disease—especially when it coexists with other disease processes.
While the anatomic variation of congenital heart disease is vast, the four main elements of pathophysiology in congenital heart disease are desaturation, pulmonary overcirculation, pulmonary vascular disease, and compromised systemic perfusion. The interventions we undertake for patients with congenital heart disease are done to address or prevent one or more of these issues. These pathologies are discussed more fully in the context of the more common examples of congenital heart disease.
CLINICAL PRESENTATION
Congenital heart disease typically presents in one of three time frames: the newborn period, in early infancy, or in childhood. The newborn period usually sees most presentations of critical congenital heart disease. What defines these lesions as “critical” is that survival depends on ductal patency, or emergent surgical or catheter intervention. Typical clues to suggest critical heart disease in the newborn include cyanosis or signs and symptoms of decreased perfusion. Increasingly, newborns with congenital heart disease are born with detailed anatomic diagnoses due to prenatal screening by fetal echocardiography. In some centers, more than half of infants born with serious congenital heart disease have been diagnosed antenatally. Antenatal diagnosis allows for appropriate and anticipatory management of these infants and has led to a measure of improved outcome for complex heart disease.4 One other important presentation for newborns with critical congenital heart disease is the screening program for critical congenital heart disease endorsed by the Centers for Disease Control (CDC) and now adpoted by legislation in over half of the states in the US. This simple screening uses a pulse oximeter to check saturations in the right hand and foot in newborns more than 24 hours old or immediately pre-discharge. Any reading <90%, three readings 90% to 94%, or a more than 3% difference between the hand and the foot triggers the recommendation for a diagnostic echocardiogram. This test will detect most cyanotic congenital heart disease as well as hypoplastic left heart syndrome, and other mixing lesions. A recent meta-analysis of several published studies shows a high sensitivity of this test (76%) for critical congenital heart disease and a low false positive rate (0.05%) when done >24 hours after birth.5
A second group of patients with congenital heart disease presents in the weeks after birth as the pulmonary resistance falls to its nadir at 8 to 12 weeks of life. This group may present with a murmur, or with symptoms of congestive heart failure (tachypnea, hepatomegaly) if there is significant pulmonary overcirculation. Late presentations associated with closure of the ductus arteriosus may also present in this time frame with cyanosis, shock, or decreased femoral pulses.
The third common time frame for diagnosis of congenital heart disease is during childhood, with a less-intense heart murmur. This often happens between age 2 and 5 years when the child finally is cooperative to remain still for a minute or two it takes to get a good quiet listen with a stethoscope.
In addition to the more usual presentations of congenital heart disease, there are infants and children with serious cardiovascular malformations that masquerade as more common illnesses such as lung disease or laryngomalacia (Table 53-1).
Masquerading Disease | Congenital Heart Disease | Hint/Clue | Definitive Diagnostic Test |
---|---|---|---|
Frequent lower respiratory illnesses Asthma / bronchiolitis Failure to thrive |
Ventricular septal defect Patent ductus arteriosus Atrioventricular canal defect Aortopulmonary window with elevated pulmonary vascular resistance |
Loud, single second heart sound Abnormal ECG |
Echocardiogram |
Croup Laryngomalacia Bronchomalacia Feeding difficulties Reflux |
Vascular ring | Often right aortic arch on chest x-ray |
CT scan MRI Bronchoscopy |
Asthma Bronchiolitis Dilated cardiomyopathy |
Anomalous left coronary artery from the pulmonary artery (ALCAPA) |
Cardiomegaly on CXR Abnormal ECG (infarction pattern) |
Echocardiogram (looking specifically at origin of coronary arteries) |
Hypertension | Coarctation of the aorta | Decreased intensity of femoral pulses and/or radio-femoral delay |
Four extremity blood pressures Echocardiogram |
Severe neonatal respiratory distress | Total anomalous pulmonary venous return (with obstruction) | History not suggestive for infant at-risk for pulmonary disease | Echocardiogram (complete, but especially focusing on pulmonary veins) |
DIFFERENTIAL DIAGNOSIS
For infants who are critically ill with low oxygen saturation and/or hypotension, the more common diagnoses of infancy are usually first to be considered. Respiratory distress syndrome, sepsis, meconium aspiration syndrome, pneumonia, and others are usually first considered because their incidence is much higher. Critical heart disease should especially be suspected when cyanosis is present without accompanying respiratory distress, or there are signs of decreased systemic perfusion such as weak or absent femoral pulses and/or metabolic acidosis (Table 53-2). A high index of suspicion for heart disease should be maintained for infants who appear to have severe respiratory disease but who are not improving with conventional management.
PDA Required to Support Pulmonary Flow | PDA Required to Support Systemic Flow | PDA Increases Mixing |
---|---|---|
Critical pulmonary stenosis Pulmonary atresia/intact ventricular septum Tricuspid atresia (normally related great arteries) Tetralogy of Fallot (some) Complex single ventricle with pulmonary stenosis/atresia |
Critical aortic stenosis Critical coarctation/Interrupted aortic arch Tricuspid atresia (transposed great arteries) Hypoplastic left heart syndrome Complex single ventricle with systemic outflow obstruction |
d-Transposition of great arteries (d-TGA) |
When critical heart disease is suspected in the neonate, diagnostic and empiric interventions need to be performed simultaneously. For diagnosis, consultation with a pediatric cardiologist and full transthoracic echocardiogram is indicated. With such evaluation, the diagnosis of virtually all congenital heart disease in the newborn period is possible. Where the index of suspicion for heart disease is lower, a chest radiograph, electrocardiogram, and hyperoxic test should be performed. The hyperoxic test is performed by placing the infant on 100% FiO2 and measuring a post-ductal arterial PO2. A value of >200 Torr almost always excludes cyanotic congenital heart disease. A value lower than that or an infant who has poor perfusion or remains critically ill despite the usual treatment measures for these other conditions should have a complete echocardiogram performed and interpreted by a physician qualified to diagnose complex congenital cardiac disease.
In those infants suspected of having critical heart disease, empiric therapy with intravenous prostaglandin E-1 should be commenced immediately at a dose of between 0.01 μg/kg/min and 0.05 μg/kg/min. Prostaglandin E-1 will reopen a closing ductus arteriosus and restore pulmonary or systemic flow in critical heart disease, temporizing the situation and allowing time for diagnosis and planned intervention. Side effects of prostaglandin include apnea, hypotension, tachycardia, and fever.
Two points are important to make at this juncture:
-
A “normal” fetal ultrasound or even an echocardiogram should not be reason to exclude the consideration of serious congenital heart disease. The fetal diagnosis of congenital heart disease is a very operator-dependent test. There are many instances where infants with hearts diagnosed as “normal” in-utero turn out to have critical congenital heart disease.
-
An echocardiogram done on a critically ill newborn is a very difficult test to perform and interpret accurately. The infant may be ventilated on an oscillator, be very labile with blood pressure or saturation to even brief imaging, and a whole other host of hemodynamic factors may make the acquisition and interpretation difficult. In addition, some of the most difficult diagnoses to make by echocardiogram (such as obstructed total anomalous pulmonary venous return) typically present in this fashion. While more neonatologists, radiologists and hospitalists are acquiring advanced training in pediatric echocardiography, it is imperative that the physician caring for the critically ill infant does not overstep his/her degree of expertise when assessing for potential critical heart disease.6
DIAGNOSTIC EVALUATION FOR CONGENITAL HEART DISEASE
The recognition of congenital heart disease rests on the foundation of a good clinical history and physical examination. The electrocardiogram may add supplemental information to the assessment of cardiac anatomy, and is necessary for diagnosis of rhythm problems. The diagnosis of congenital heart disease has been revolutionized by two-dimensional echocardiography. Echocardiography provides a detailed anatomic and physiologic diagnosis of congenital heart disease, is noninvasive, and is performed at the bedside. Coupled with the Doppler technique, blood velocity may also be assessed, and this can be used to estimate chamber pressures and presence of heart or great vessel obstruction or valve regurgitation. Because almost all the ultrasound waves are reflected at tissue–air interfaces, echocardiography is limited to views where the lungs do not obstruct the path between the ultrasound beam and the heart. Factors that limit the usefulness of transthoracic echocardiography include obesity, obstructive lung disease, and certain chest wall deformities. Three-dimensional echocardiography is in the early stages of clinical application and may provide additional understanding of the anatomic information
Magnetic resonance imaging (MRI) is increasingly being used for diagnostic evaluation of congenital heart disease. Advantages of cardiac MRI include excellent anatomic detail that is not limited by lung tissue, and MRI is therefore an especially good modality for imaging the great vessels. A major disadvantage is the requirement for sedation and the relatively long post-processing and interpretation time. Functional assessment of blood flow by MRI is possible and in many instances may be more accurate than echocardiography. Implanted metal such as surgical clips or embolization coils render bothersome imaging artifacts in many patients when performing cardiac/thoracic MRIs.
MRI is generally contraindicated in patients with pacemakers and defibrillators.
Cardiac catheterization with angiography was once considered the gold standard for anatomic diagnosis. However, echocardiography and MRI have now almost completely supplanted cardiac catheterization for anatomic diagnosis. Cardiac catheterization remains the gold standard for hemodynamic assessment, and increasingly cardiac catheterization is being used as an interventional rather than diagnostic tool.
MANAGEMENT OF CONGENITAL HEART DISEASE
Definitive treatment of congenital heart disease is complex due to the wide variety of anatomy, the physiologic changes that occur over time (e.g. fall in pulmonary vascular resistance), and growth. Straightforward heart lesions are approached with surgical or transcatheter techniques that address a single problem (e.g. atrial septal defect). More complex forms of congenital heart disease are approached by cardiologists and cardiovascular surgeons by first attempting to decide if there are enough required cardiac “parts” to immediately or eventually repair the heart in such a way that it is physiologically, if not anatomically, correct. A “complete” or “two-ventricle” repair entails having separate systemic and pulmonary circuits, each with its own ventricle and atrioventricular valve able to pump deoxygenated blood to the lungs and oxygenated blood to the body. Although this type of repair is referred to as a “complete,” the resulting cardiac anatomy is often very different from normal. Where possible, complete repair is generally the preferred option.
If there are insufficient “parts” to perform a complete repair (e.g. hypoplastic left heart syndrome, tricuspid atresia) then a single ventricle pathway must be pursued. The single ventricle pathway typically follows as a series of palliative surgeries that culminate with a Fontan procedure. This staged cardiac surgery ultimately results in a circulation where the venous blood return is passively routed to the lungs without passing though a ventricle, and then returns to a functionally single ventricle that pumps to the body. For this type of repair to succeed, the patient must have a relatively competent atrioventricular valve, good systolic ventricular function, unobstructed outflow from the heart, widely patent pulmonary arteries, and low pulmonary vascular resistance. The vascular resistance of the neonatal pulmonary vascular bed is too high to accept the passive pulmonary flow of a Fontan, and so a series of operations is necessary to ultimately achieve this type of palliation.
The first stage of the single ventricle pathway provides for adequate pulmonary blood flow and unobstructed systemic flow in the newborn period (Figure 53-1). If the original cardiac anatomy is such that there is insufficient pulmonary flow, a shunt is surgically placed to provide flow from the systemic circulation to the pulmonary circulation taking the place of the ductus arteriosus. One of the more common types of shunt is the modified Blalock-Taussig shunt, which is a synthetic tube, placed from the subclavian artery to the ipsilateral pulmonary artery. There are many types of shunts and modifications of techniques, which depend on the individual anatomy and operator preference.
FIGURE 53-1.
Stage I single ventricle pathway for hypoplastic left heart syndrome (see Figure 53-8 for unrepaired anatomy). 1. Right modified Blalock-Taussig shunt. 2. Large (surgically created) atrial septal defect. 3. Main pulmonary artery has been detached form branch pulmonary arteries. 4. Unobstructed egress of blood from the heart to the body has been achieved by using the main pulmonary artery and native aorta with additional patch material. (Reproduced from Sinclair CM. The Report of the Manitoba Pediatric Cardiac Surgery Inquest: An Inquiry Into Twelve Deaths at the Winnipeg Health Sciences Center in 1994. 2000. Available at http://www.pediatriccardiacinquest.mb.ca/pdf/index.html. Accessed March 21, 2017.)

The infant with an “ideal” physiology following a shunt has a systemic oxygen saturation of about 80%. Empirically this saturation has been found to be the best balance between systemic oxygen delivery and pulmonary overcirculation. Because blood circulating to the lungs is already partially saturated, shunt physiology is inefficient, and even with this “ideal” saturation of 80%, there is still about twice as much blood being circulated to the lungs as to the body.
If the original cardiac anatomy dictates the single ventricle pathway but there is too much pulmonary flow such that the pulmonary pressures are high and/or the infant is in congestive heart failure, then the first stage in palliation is a pulmonary artery band (PAB). A PAB is a surgical “noose” placed around the pulmonary artery that creates an artificial stenosis and limits pulmonary flow.
Of great importance in the single ventricle pathway is the provision for an unobstructed pathway for systemic blood flow. The proximal pulmonary artery and aorta are often used together to recreate a single unobstructed outflow from the heart in this first stage.
At about 4 to 8 months of age, the pulmonary vascular resistance has typically decreased sufficiently to accept a portion of the venous return in a passive manner (Figure 53-2). The second step of cardiac palliation for children proceeding down the single ventricle pathway is the “bidirectional Glenn,” or stage II. This involves connecting the superior vena cava directly to the pulmonary arteries and eliminating any previous shunted source of pulmonary blood flow. The arterial saturations are usually about the same before and after the procedure (~80%); however, this type of circulation is preferable because it is more efficient. In the shunted scenario, the single ventricle pumps blood to both the body and the lungs, and the blood pumped to the lungs is partially saturated—an inefficient type of arrangement. After the stage II repair, the heart is only pumping blood to the body, and only desaturated blood is flowing to the lungs, thereby increasing the efficiency.
FIGURE 53-2.
Stage II single ventricle pathway for hypoplastic left heart syndrome. 1, 2. The right modified Blalock-Taussig shunt that was created at the stage 1 repair has been removed. The superior vena cava is divided and the proximal portion is attached directly to the right pulmonary artery, allowing the blood from the superior vena cava (SVC) to flow to both lungs (“bidirectional Glenn”). “Bidirectional” refers to the SVC blood flowing to both the right and left lungs. (Reproduced from Sinclair CM. The Report of the Manitoba Pediatric Cardiac Surgery Inquest: An Inquiry Into Twelve Deaths at the Winnipeg Health Sciences Center in 1994. 2000. Available at http://www.pediatriccardiacinquest.mb.ca/pdf/index.html. Accessed March 21, 2017.)

The third stage (Fontan completion) consists of redirecting the blood from the inferior vena cava to the lungs, thereby separating the pulmonary and systemic circuits (Figure 53-3). Directing the inferior vena cava blood to the lungs may be accomplished by use of a synthetic tube lateral to the heart (external conduit) or using part of the right atrium as a wall (lateral tunnel). Often a small communication is left in the tube (fenestration) that may later be closed in the cardiac catheterization lab. After closure of the fenestration, these patients have normal oxygen saturations. Stage III is usually performed between ages 2 to 5 years, depending on center preference and technique.
FIGURE 53-3.
Stage III –single ventricle pathway for hypoplastic left heart syndrome. 1. A tube has been placed within the right atrium to direct the blood from the inferior vena cava to the superior vena cava. The superior vena cava is reattached to the undersurface of the pulmonary artery. 2. Fenestration: a small hole is left in the tube to allow some of the venous blood to bypass the lungs. The fenestration keeps the venous pressure from rising too high after the Fontan operation, but results in some desaturation. The fenestration may be optional in some patients, but if desired can be easily closed in the cardiac catheterization laboratory afterward. (Reproduced from Sinclair CM. The Report of the Manitoba Pediatric Cardiac Surgery Inquest: An Inquiry Into Twelve Deaths at the Winnipeg Health Sciences Center in 1994. 2000. Available at http://www.pediatriccardiacinquest.mb.ca/pdf/index.html. Accessed March 21, 2017.)

Postoperative problems encountered by patients with Fontan-type repairs include early problems such as prolonged pleural effusions, and late problems such as increased risk for stroke and thrombus formation in the Fontan, arrhythmia development (especially atrial flutter), and protein-losing enteropathy.
CRITICAL NEWBORN HEART DISEASE
In this set of lesions, a portion of the normal anatomy supporting pulmonary blood flow (tricuspid valve, right ventricle, pulmonary valve, and pulmonary arteries) is small or absent. The ductus arteriosus provides pulmonary blood flow by shunting systemic blood flow to the lungs. While d-transposition of the great arteries (d-TGA) is also included in this section, the physiology of this lesion is somewhat different, as explained below.
The tricuspid valve is atretic in this lesion and the right ventricle is typically severely hypoplastic (Figure 53-4). The pulmonary artery arises from the right ventricle, and there may be a ventricular septal defect. When the ventricular septal defect and/or pulmonary artery are hypoplastic, pulmonary blood flow is inadequate and the ductus arteriosus is required to support pulmonary blood flow. In some instances, there may be adequate pulmonary flow through the ventricular septal defect, and in other cases the ventricular septal defect and pulmonary artery may be so large that the infant has too much pulmonary blood flow.
FIGURE 53-4.
Tricuspid atresia (normal great arteries). 1. Patent foramen ovale with flow right to left. 2. Atretic tricuspid valve. 3. Ventricular septal defect (VSD). Size of VSD and amount of restriction is variable. (Reproduced from Sinclair CM. The Report of the Manitoba Pediatric Cardiac Surgery Inquest: An Inquiry Into Twelve Deaths at the Winnipeg Health Sciences Center in 1994. 2000. Available at http://www.pediatriccardiacinquest.mb.ca/pdf/index.html. Accessed March 21, 2017.)
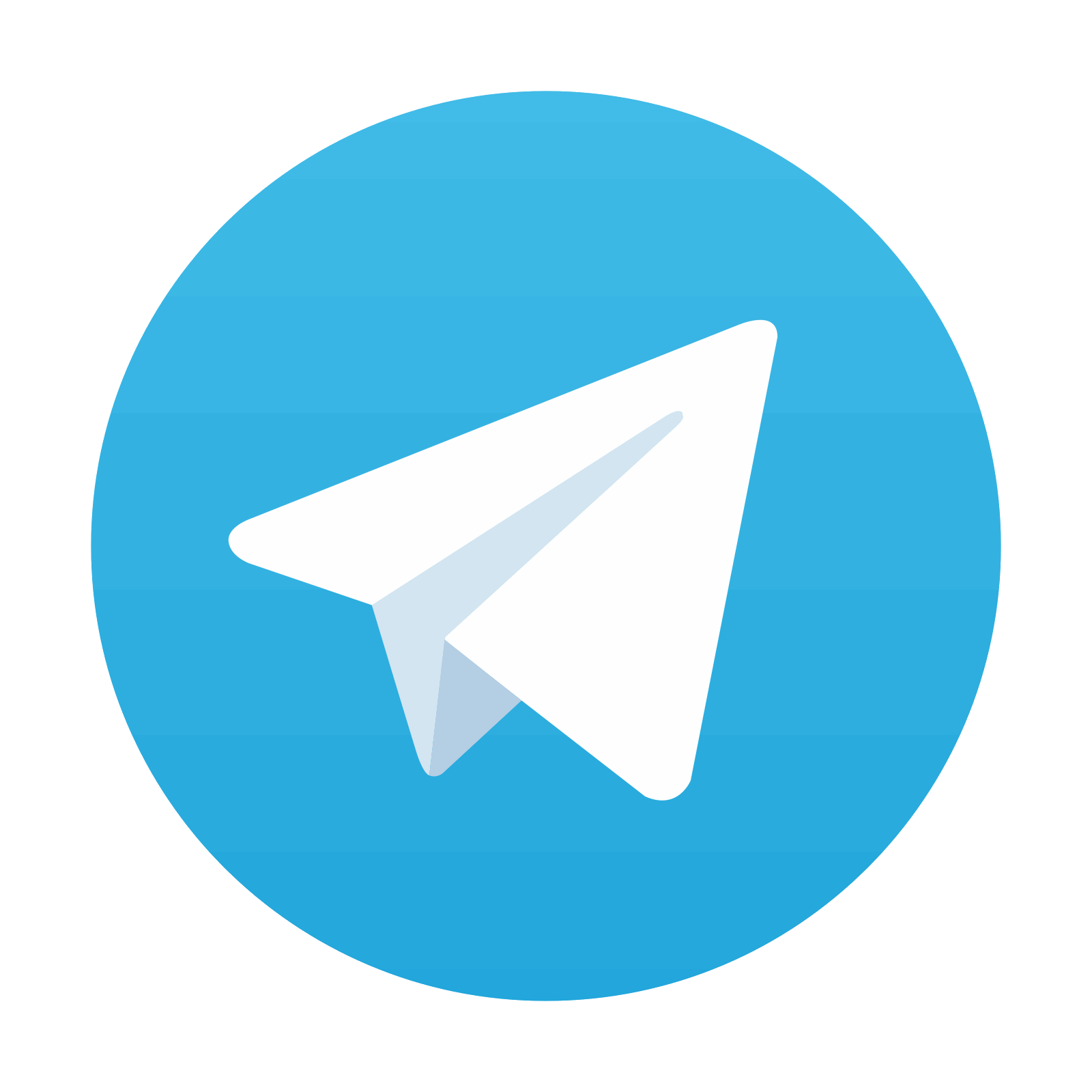
Stay updated, free articles. Join our Telegram channel

Full access? Get Clinical Tree
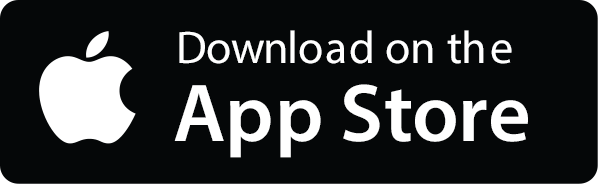
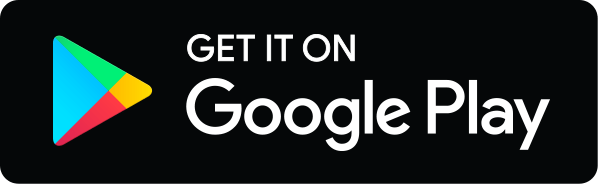