The spectrum of congenital brain malformations is complex and classification schemes are imperfect. Neuroimaging evaluations provide extensive information concerning abnormal brain morphology and cellular organization. Correlation of this information with well-established concepts of normal embryological and fetal neural development provides a conceptual framework for a greater understanding of brain anomalies. The most frequently utilized neuroimaging classification scheme for congenital malformations of the brain recognizes 4 major types that correlate to stages of development: (1) dorsal induction (primary neurulation), (2) ventral induction (telencephalization), (3) neuronal proliferation, differentiation, and histogenesis, and (4) neuronal migration. It is important to recognize, however, that this system is an oversimplification of a heterogeneous and complex group of disorders.
At about 16 days’ gestational age, the embryo consists of a 3-layered disc. Cells from the primitive node migrate between the endoderm and ectoderm cranially and form the notochordal process, the structure around which the vertebral column forms. The notochordal process fuses with endoderm and reaches the oropharyngeal membrane by day 18. There is concomitant thickening of ectodermal cells that overlie the notochord, thereby forming the neural plate. On day 18, neural plate cells along the dorsal aspect of the notochordal process invaginate, forming the neural groove. Cells lateral to the groove thicken and give rise to the neural folds. The neural folds move medially and close over the neural groove, forming the neural tube. Closure of the neural tube begins in the cervical region and proceeds toward both ends of the embryo. The brain ventricular system and the spinal cord central canal develop from the lumen of this tube. The walls of the neural tube eventually thicken to form the brain and the spinal cord. Dorsal induction refers to the sequence of embryological events in the dorsal aspect of the embryo that culminate in formation of the neural tube.
Neurulation is the process of neural tube closure. Primary neurulation encompasses initial closure of the neural tube from the anterior neuropore to the upper lumbar segments. Secondary neurulation refers to the events that produce closure of the more caudal segments. Various congenital anomalies result from failure of appropriate neural tube closure during the third and fourth weeks of development. Deficient neurulation encompasses a spectrum of malformations that includes anencephaly, exencephaly, encephalocele, and Chiari II malformation. Craniorachischisis is total failure of neurulation: central nervous system (CNS) tissue exists only as a neural plate that is devoid of dorsal osseous or dermal covering.
Ventral induction refers to the series of events after neurulation that lead to the formation of 2 separate cerebral hemispheres (i.e., diverticulation). Luminal epithelial cells secrete a watery liquid into the closed neural tube. The cephalic end becomes somewhat distended with fluid and forms the primary brain vesicles: the prosencephalon, mesencephalon, and rhombencephalon. The prosencephalon (forebrain) consists of 2 secondary vesicles: the telencephalon and the diencephalon. The telencephalon gives rise to the cerebral hemispheres, putamina, caudate nuclei, retinae, and optic nerves. The diencephalon gives rise to the thalami, hypothalami, globi pallidi, pineal gland, and neurohypophysis. The rhombencephalon (hindbrain) partially divides into the metencephalon, which forms the cerebellum, and the myelencephalon, which forms the pons and medulla.
Developmental disturbances during the ventral induction stage can lead to the various forms of holoprosencephaly, septooptic dysplasia, pituitary anomalies, absence of the septum pellucidum, and olfactory aplasia. The prechordal mesenchyme that induces diverticulation of the primitive forebrain into paired cerebral vesicles also stimulates formation of the premaxillary facial structures from overlying ectoderm. Therefore, craniofacial anomalies often occur in association with intracranial disturbances of diverticulation.
Embryogenesis of the major posterior fossa structures occurs simultaneous to the ventral induction stage of cerebral development. The 2 major divisions of the rhombencephalon are the metencephalon and the more caudally located myelencephalon. The walls of the metencephalon form the pons and the cerebellum; the fluid-filled cavity forms the superior portion of the fourth ventricle. The myelencephalon gives rise to the medulla and the inferior portion of the fourth ventricle. Anomalies of posterior fossa development include Dandy-Walker malformation, Joubert syndrome, rhombencephalosynapsis, cerebellar hypoplasia, vermian hypoplasia, and mega cisterna magna.
Development of the cerebral cortex occurs in 3 overlapping stages. During the first stage (approximately weeks 5 to 18), stem cells proliferate into neuroblasts and glial cells in the ventricular and subventricular germinal zones that line the cerebral cavity of the forebrain; this is the proliferation stage. After the initial mitotic division of these neurons, neuronal migration from the germinal matrix occurs during the second, or migration, phase (weeks 6 to 22). This neuronal migration occurs in a radial fashion toward the pial surface. Successive generations of neurons pass one another within the cortical plate. The third phase, or organization stage, represents organization of cells in the cortex into 6 layers. Synaptogenesis and apoptosis occur during this phase. Sulcation refers to the process by which the surface of the brain develops a convolutional pattern of gyri and sulci. Sulcation involves neuronal migration, glial cell proliferation, neuronal growth and differentiation, and influx of afferent fibers.1
Anomalies that predominantly involve disordered neuronal proliferation, histogenesis, and differentiation include primary megalencephaly, hemimegalencephaly, primary micrencephaly, neurocutaneous syndromes, and primary aqueductal stenosis. Abnormalities of neuronal migration and sulcation include schizencephaly, lissencephaly, and gray matter heterotopias. Abnormal cortical organization can lead to polymicrogyria or schizencephaly. Although not pure migrational anomalies, disorders of the corpus callosum apparently occur at a similar time during development. Commissural fibers that form the corpus callosum develop between weeks 8 and 17 of gestation; alterations in this process result in hypoplasia or aplasia of the corpus callosum.
Brain myelination begins during the fourth and fifth months of gestation and continues throughout childhood. The period of most active myelin synthesis is during the first 8 postnatal months. The temporal sequences of myelination vary in different brain regions and structures. In general, the pattern of myelination reflects the evolving functional capacity of the CNS. The spinal cord, brainstem, central cerebellar white matter, and posterior limbs of the internal capsules are the initial areas of myelination. Peripheral cerebral white matter is the last area to become myelinated. There are myriad congenital and acquired abnormalities of brain myelin.
Sonography and MRI provide accurate depiction of brain development in the fetus and infant. MRI is the superior technique for assessing brain composition and structure, particularly with the implementation of diffusion-tensor techniques.2 The brain of the fetus and premature infant is lissencephalic, with a smooth surface and lack of white matter interdigitation (Figures 14-1 and 14-2). At 26 weeks’ gestation, the white matter is hypointense to gray matter on T1-weighted images and hyperintense on T2-weighted images. Decrease in water content because of myelin formation results in conversion to hyperintensity on T1-weighted images and hypointensity on T2-weighted images (Figure 14-3). MRI provides accurate assessment of this orderly process (Table 14-1 and Figure 14-4). Most normal children have MR evidence of near-complete myelination by approximately 2 years of age. Persistence of high T2 signal intensity because of incomplete myelination until the fourth birthday is common in the peritrigonal white matter (the terminal zone) and the frontotemporal subcortical white matter (Figure 14-5). Occasionally, mild T2 hyperintensity in the terminal zone persists into early adolescence.3,4
Figure 14–3
Normal brain development.
Midline T1-weighted MR images of 4 infants at different ages. A. Six days. The corpus callosum is thin and unmyelinated. The early stage of myelination in the cord and brainstem (except the ventral pons) results in subtle hyperintensity. Note hyperintensity of both the anterior and posterior lobes of the pituitary. B. Three months. There is increased myelination of the ventral pons, cerebellar vermis, and dorsal aspect of the corpus callosum. C. Eight months. The brainstem and vermis now have an adult pattern. The corpus callosum is myelinated but is still somewhat thin. D. Eighteen months. The corpus callosum has mature morphology and myelination.

Age in monthsa | ||
---|---|---|
Structure | T1 | T2 |
Superior cerebellar peduncles | (28 GA) | (28 GA) |
Posterior limb internal capsule, posterior aspect | (36 GA) | (40 GA) |
Middle cerebellar peduncles | 0 | 0–2 |
Posterior limb internal capsule, anterior aspect | 0–1 | 4–7 |
Cerebellum | 0–3 | 3–5 |
Anterior limb internal capsule | 1–3 | 5–11 |
Splenium of corpus callosum | 3–4 | 4–6 |
Genu of corpus callosum | 5–6 | 7–8 |
Occipital white matter | 4–7 | 12–14 |
Frontal white matter | 5–11 | 16–18 |
Figure 14–4
Normal brain myelination.
T2-weighted images in children of different ages. A. Six days. Beginning myelination in the posterior limbs of the internal capsules (arrow) is hypointense. The adjacent thalamic nuclei are also hypointense. B. Two months. There is additional myelination of the posterior aspects of the posterior limbs. The basal ganglia and thalami are hypointense relative to the unmyelinated cerebral white matter. C. Seven months. The entire posterior limbs are myelinated. There is faint hypointensity in the anterior limbs. D. Twelve months. There is myelination of the anterior and posterior limbs of the internal capsules (arrows) and the genu and splenium of the corpus callosum. Hyperintensity of the cerebral white matter is less pronounced.

As described above, the ventricular system develops from the lumen of the primitive neural tube. Early during brain development, a vascular layer of the pia matter is located outside of the ependymal roof of the fourth ventricle. This fuses with the ependyma to form the tela choroidea, which subsequently invaginates into the fourth ventricle and develops into the choroid plexus of the fourth ventricle. A similar process occurs within the roof of the third ventricle and in the medial walls of the lateral ventricles. Thereby, there is formation of four choroid plexuses. These secrete cerebrospinal fluid into the embryonic ventricular system. The thin roof of the fourth ventricle ruptures during the second trimester to give rise to the foramina of Luschka and the foramen of Magendie.
Normal developmental variations of the ventricular system include the cavum septum pellucidum, cavum vergae, and cavum velum interpositum. The cavum septum pellucidum is a fluid filled space within the membrane that separates the lateral ventricles (Figure 14-6). A cavum septum pellucidum is present in nearly all newborns; subsequent complete fusion occurs in approximately 80% of individuals. Fusion of the septal lamellae occurs from posterior to anterior. A cavum vergae represents extension of a cavum septum pellucidum posterior to the columns of the fornix. This normal variation occurs in approximately 33% of newborns and spontaneously disappears in approximately 99% of individuals. Rarely, a cavum septum pellucidum or cavum vergae serves as a site of origin of an intraventricular arachnoid cyst. Because there is posterior-to-anterior fusion of the septal lamellae, a cavum septum pellucidum invariably accompanies a cavum vergae; that is, a cavum septum pellucidum et vergae (Figure 14-7). The cavum veli interpositi (cavum velum interpositum) is an anterior extension of pia-arachnoid from the quadrigeminal plate cistern. This fluid-filled structure is inferior to the body of the fornix, contains the internal cerebral veins, and can extend as far forward as the columns of the fornix (Figure 14-8). In contradistinction to an arachnoid cyst, cavum veli interpositi does not cause substantial mass effect.5
Anencephaly is a severe fatal defect of neuraxis development. Major portions of the cranium and CNS fail to develop or are destroyed during the first trimester. The calvarial defect involves the frontal, parietal, and occipital bones, often with extension into the cervical spine. The forebrain is absent. Most often, the entire cerebrum consists of a small nonfunctioning fibrotic mass of neural tissue. Concomitant malformations of the cerebellum, brainstem, optic nerves, and spinal cord are often present. Anomalies of other organ systems are also common. Anencephaly leads to spontaneous abortion, stillbirth, or neonatal death. The prevalence is 1.2 per 10,000 births and up to 1 per 1000 pregnancies.6
There are 3 types of anencephaly: meroanencephaly, holoanencephaly, and craniorachischisis. Meroanencephaly is characterized by rudimentary brain tissue and partial formation of the cranium. Abnormal tissue, called the area cerebrovasculosa, protrudes through a median cranial defect. The area cerebrovasculosa consists of abnormal spongy vascular tissue admixed with glial tissue. It ranges from a thin membrane to a large pseudoencephalic mass that simulates cerebral tissue. Holoanencephaly is the most common type of anencephaly. The brain is completely absent. Craniorachischisis is the most severe type of anencephaly, with defects in the head and spine. The area cerebrovasculosa and area medullovasculosa (vascular mass of rudimentary spinal cord at the site of a bony defect) fill cranial and spinal column defects.
Standard radiographs of the newborn infant with anencephaly demonstrate severe microcephaly and absence of large portions of the membranous calvaria. Facial anomalies may also be present. Prenatal ultrasound shows absence of the normal fetal skull and brain above the level of the orbits (Figure 14-9). Occasionally, there is echogenic angiomatous stroma (area cerebrovasculosa) at the expected site of the cerebrum. During the first trimester, sonography may demonstrate relatively intact cerebral hemispheres with absent membranous calvaria. Echogenic particles of neural tissue are sometimes visible floating in the amniotic fluid. Polyhydramnios is present in about half of these pregnancies. α-Fetoprotein levels are elevated in maternal serum and amniotic fluid.7–9
Figure 14–9
Anencephaly.
A. There is lack of normal cranial development (C) on this longitudinal prenatal ultrasound image. The arrows mark the spine and the arrowheads indicate the base of the head. B. An anteroposterior radiograph of the stillborn infant shows lack of the membranous calvaria. Facial bones are present.

Exencephaly is a rare neural tube defect in which a large portion of the brain is located outside of the skull. Early fetal manifestations of exencephaly on sonography sometimes progress to those of anencephaly later in gestation, a result of necrosis of neural tissue that is exposed to amniotic fluid. This anomaly usually results in fetal death. Skull radiographs of the neonate with exencephaly demonstrate absence of the membranous portions of the bony calvaria. There is also variable deficiency of the skull base. There is a disorganized mass of cerebral tissue.10,11
The Chiari malformations are a group of congenital hind-brain anomalies that involve the posterior fossa and craniocervical junction. The various types of Chiari malformation are clinically and pathophysiologically distinct (Table 14-2). Each likely involves failure of normal dorsal induction. These anomalies are named after Hans Chiari, a pathologist at the University of Prague, who described types I to III of these malformations in 1891. Chiari malformation type II is also termed Arnold-Chiari malformation, in recognition of the German pathologist Julius Arnold. The type IV and type 0 malformations are later additions.
Chiari malformations | Major features |
---|---|
I | Caudal extension of the cerebellar tonsils |
Occipital/craniocervical deformities | |
Syringohydromyelia is common | |
Scoliosis is common | |
II | Caudal descent of vermis and medulla |
Spinal dysraphism | |
Hydrocephalus | |
Associated CNS anomalies are common | |
III | Herniation of portions of the cerebellum and brainstem into a sac at the craniocervical junction |
IV | Aplasia or marked hypoplasia of cerebellum and tentorium |
0 | “Crowded” posterior fossa; no tonsillar herniation |
Syringomyelia is common |
Chiari I malformation consists of downward herniation of the cerebellar tonsils into the cervical spinal canal. This type of Chiari malformation clinically occult at birth in nearly all affected patients. Chiari II malformation occurs in association with myelodysplasia. There are numerous developmental abnormalities of the brain and skull in these patients, including caudal extension of vermis and medulla into the cervical spinal canal. Chiari III malformation refers to a low occipital or high cervical encephalocele in combination with anomalies of the brainstem and cerebellum. Chiari IV malformation refers to hypoplasia or aplasia of the cerebellum and tentorium. Most investigators exclude this anomaly from the Chiari malformation spectrum since there is no hindbrain herniation. Some clinicians apply the term Chiari 0 to patients with symptoms of Chiari I malformation and a “crowded” posterior fossa, but no caudal displacement of the cerebellar tonsils.12–14
Chiari I malformation consists of downward displacement of the cerebellar tonsils through the foramen magnum into the cervical spinal canal, unrelated to acquired intracranial pathology. There also is variable inferior displacement of the brainstem. The cerebellar tonsils are elongated and often have a peg-like or pointed shape. The tonsillar morphology is often asymmetric. Symptoms in these patients can result from alteration in cerebrospinal fluid flow dynamics in the region of the foramen magnum or from compression of neural structures. Fibrous adhesions that develop between the dura, arachnoid, and tonsils can obstruct the fourth ventricular outlet foramina. A constricting band of dura at the level of the foramen magnum and arch of the atlas is common in patients with Chiari I malformation. Occasionally, there is flattening or buckling of the medulla. Congenital malformations of the supratentorial structures are rare in patients with Chiari I malformation.15
Osseous deformities of the occipital region and craniocervical junction occur in approximately half of patients with Chiari I malformation. The volume of the posterior fossa tends to be somewhat small; this may be the primary etiological factor for the tonsillar ectopia in many individuals (i.e., a developmental disorder of the paraxial mesoderm). Flattening and elevation of the squamous portion of the occipital bone are often present, resulting in a shallow posterior fossa. The tentorium may have a steep orientation (Figure 14-10). Other potential findings include platybasia, basilar impression, Klippel-Feil deformity, a concave clivus, atlantoaxial assimilation, and atlanto-occipital fusion. Syringohydromyelia occurs in 50% to 75% of individuals with Chiari I malformation; the cervical region is the most common location. Scoliosis is present in nearly 90% of individuals with Chiari I malformation. Single curves and convex left curves are more common in these patients than in those with idiopathic scoliosis.16–18
Figure 14–10
Chiari I malformation.
A sagittal T2-weighted MR image of a 1-year-old child shows herniation of a thin tongue of the cerebellum (arrow) into the cervical spinal canal. The posterior fossa volume is small. There is a vertical configuration of the tentorium. The superior aspect of the odontoid process is somewhat small.

The estimated prevalence of Chiari I malformation in the general population is approximately 0.5%. Many individuals with this anomaly are asymptomatic, however. Potential clinical manifestations include headaches, nystagmus, motor deficits, sensory deficits, and manifestations of syringohydromyelia (Figure 14-11). Scoliosis may result in clinically evident deformity and back pain. There is considerable variation in the nature and severity of the clinical consequences of Chiari I malformation; the severity of tonsillar ectopia is a weak indicator of symptomatology. In general, herniation of greater than 12 mm is usually symptomatic. Approximately 30% of individuals with displacements between 5 and 10 mm are asymptomatic. Patients with a small posterior fossa volume are prone to develop symptoms. Prominent retroflexion of the dens is often associated with syringohydromyelia. There is a moderate female predilection of Chiari I malformation. Occasional cases are familial.19
Figure 14–11
Chiari I malformation.
This 14-year-old girl presented with headaches precipitated by coughing. She also noted increasing clumsiness over the past several months. There is caudal extension of the cerebellar tonsils below the neural arch of C1. The foramen magnum (arrows) is enlarged. There is slight impression on the medulla by the odontoid (artifact from orthodontic hardware distorts the anterior spinal structures). Septated syringohydromyelia is present in the cervical cord.

MR is the imaging study of choice for the diagnosis and characterization of Chiari I malformation. The major findings are tonsillar herniation, obliteration of the retrocerebellar subarachnoid spaces, and cranial base dysplasia (Figure 14-12). The generally accepted imaging criterion for the diagnosis of Chiari I malformation is herniation of 1 or both cerebellar tonsils 5 mm or more below the foramen magnum (measuring from the opisthion-basion line) in a patient without a predisposing factor such as myelodysplasia, hydrocephalus, intracranial mass, or prior craniocervical junction surgery. Also included in the diagnosis are patients with herniation of both tonsils 3 to 5 mm inferior to the foramen magnum if there are accompanying features such as syringohydromyelia or cervicomedullary kinking. In most patients with Chiari I malformation, there is a short and flat clivus, sometimes associated with C1 assimilation (Figure 14-13). There is often retroflexion of the odontoid process, which can cause compression of the medulla (Figure 14-14). Occasionally, there is compression of neural structures by an elongated clivus; the superior aspect of the odontoid process is often absent in these patients. Substantial compression can lead to abnormal signal elevation in the cord, medulla, or herniated cerebellar tonsils because of edema or gliosis. In those patients with fourth ventricular outlet obstruction, there is ventriculomegaly (Figure 14-15).18,20,21
Figure 14–12
Chiari I malformation.
A sagittal T2-weighted image of a 14-year-old patient with scoliosis shows caudal extension of the cerebellar tonsils below the C1 neural arch. There is associated distortion of the upper cervical cord. The medulla extends inferior to the enlarged foramen magnum and is compressed by a slightly retroflexed odontoid process.

Figure 14–13
Chiari I malformation.
There is marked caudal extension of the cerebellar tonsils as viewed on this T1-weighted sagittal MR image. The clivus is short and the inferior aspect of the occipital bone is flattened (arrow). The posterior fossa volume is small. There is retroflexion of the odontoid.

Tonsillar ectopia and the small size of the posterior fossa in patients with Chiari I malformation alter the cerebrospinal fluid flow patterns at the foramen magnum and in the superior aspect of the cervical subarachnoid space, leading to diminished cerebrospinal fluid (CSF) flow volume and elevated CSF flow velocity. Phase-contrast flow sequences with MR are useful in selected patients to assess these flow patterns. The potential findings include lack of detectable flow at the craniocervical junction, flow jets, regions with predominantly unidirectional flow, synchronous bidirectional flow, and pulsatile systolic tonsillar descent. Abnormal CSF flow patterns in a patient with Chiari I malformation correlate with the likelihood of symptomatic disease and syringohydromyelia. MR flow evaluation, therefore, can be helpful in selecting patients who will likely benefit from surgical therapy.22–24
Chiari II malformation is a complex CNS deformity that occurs in association with myelodysplasia. Essentially all patients with myelomeningocele have Chiari II malformation. There is variable involvement of the posterior fossa structures, spine, skull, and spinal column. The most consistent features are a small posterior fossa, tentorial dysplasia, and hydrocephalus. The primary pathophysiological event in Chiari II malformation is localized failure of neural tube closure because of deficient expression of crucial neuronal surface molecules in the developing neural tube. Cerebrospinal fluid escapes through the neural tube defect, resulting in reduced pressure in the brain vesicles, which in turn interferes with normal intracranial mesenchymal development; for example, a small posterior fossa and dysgenesis of the falx. As the cerebellum and brainstem grow in the abnormally small posterior fossa, alterations occur in hindbrain morphology, including inferior extension through the foramen magnum into the cervical spinal canal. The prevalence of Chiari II malformation is approximately 0.44 per 1000 livebirths.25
The major clinical symptoms of Chiari II malformation relate to hydrocephalus and myelodysplasia. Manifestations of brainstem or lower cranial nerve dysfunction can also occur; for example, nystagmus, dysphagia, vocal cord paralysis, or apneic spells. Clinically significant hydrocephalus is present at the time of birth in more than half of infants with Chiari II malformation. Most others develop hydrocephalus over the next several days or weeks. However, signs and symptoms of hydrocephalus are often absent in the perinatal period. Macrocephaly is often lacking, and head circumference measurements are unreliable for predicting the development of hydrocephalus. The early diagnosis of hydrocephalus in infants with Chiari II malformation frequently requires direct imaging, usually with sonography.26
The membranous potion of the skull in young infants with Chiari II malformation sometimes has a mesodermal dysplasia termed Lückenschädel, craniolacunia, or lacunar skull. Radiography and CT show a “soap bubble” pattern of focal calvarial thinning, with oval areas of lucency surrounded by sclerotic bands (Figure 14-16). The findings are most prominent in the parietal and frontal bones. Craniolacunia is a developmental abnormality that is unrelated to increased intracranial pressure or hydrocephalus. Spontaneous resolution occurs by the end of the first year of life.27
Flattening or scalloping of the posteromedial portions of the petrous pyramids is common in infants with Chiari II malformation. Scalloping of the clivus occasionally occurs. These bony changes apparently result from compression of osseous structures by the cerebellum and brainstem. Pressure effects also produce enlargement of the foramen magnum.28
Dural abnormalities in patients with Chiari II malformation include an abnormally low attachment of the tentorium, enlargement of the tentorial incisura, and fenestration or hypoplasia of the falx cerebri. Attachment of the tentorium in these patients ranges from a near-normal position to a location adjacent to the foramen magnum. A low attachment contributes to the small volume of the posterior fossa. The transverse sinuses and torcula help to define the position of the tentorial attachment on imaging studies (Figure 14-17). On axial cross-sectional imaging studies, the enlarged incisura often appears heart-shaped.
Figure 14–17
Chiari II malformation.
A. An axial T1-weighted image of a 16-year-old boy shows the cerebellum bulging through the splayed tentorial incisura (arrows). There is anterior extension of the cerebellum adjacent to the brainstem. B. A sagittal image at the midline demonstrates a vertical orientation of the tentorium (arrow). The medulla and cerebellar vermis extend from the small posterior fossa into the cervical spinal canal. The rostrum of the corpus callosum is deficient. C. A contrast-enhanced image to the right of the midline shows extension of the superior sagittal sinus (arrow) to the inferior occipital region where it merges with the vertically oriented straight sinus.


Involvement of the cerebellum in patients with Chiari II malformation is important clinically and radiographically. The volume of the posterior fossa is small and the cerebellum is variably hypoplastic. The cerebellar folia are sometimes absent. There is displacement of the inferior aspect of the cerebellar vermis through the foramen magnum into the cervical spinal canal (Figure 14-18). This “tail” or “peg” of cerebellar tissue extends a variable distance along the dorsal aspect of the cervical spinal canal. Occasionally, thin cerebellar tissue reaches the inferior aspect of the cervical spine or the upper aspect of the thoracic spine. The descended vermian tissue is usually gliotic and poorly vascularized. Caudal descent of the tonsils occasionally occurs. Upward protrusion of the cerebellar hemispheres and vermis through the enlarged incisura produces the appearance of a “pseudotumor” of the tentorium or a “towering” cerebellum on cross-sectional imaging. The cerebellum sometimes grows anteriorly and partially surrounds the brainstem (Figure 14-19). Occasionally, there is inferior extension of the posteromedial aspect of the cerebrum through the enlarged incisura.29–31
Figure 14–18
Chiari II malformation.
A sagittal T2-weighted image of a 15-day-old infant shows a small posterior fossa, marked enlargement of the foramen magnum, and inferior displacement of the medulla and cerebellar vermis into the cervical spinal canal. There is heterogenous signal in the dysplastic cerebellar tissue. The inferior tentorial attachment is near the foramen magnum. There is syringohydromyelia in the thoracic spine.

Figure 14–19
Chiari II malformation.
A. A sagittal T2-weighted MR image of a 33-day-old infant with a myelomeningocele and shunted hydrocephalus demonstrates extension of the medulla and dysplastic cerebellar tissue into the cervical spinal canal. The fourth ventricle is narrow. The inferior aspect of the tectum (arrow) is beaked posteriorly. The corpus callosum is thin. There is scalloping of the clivus. B. Superior protrusion of the cerebellum through the tentorial incisura (arrows) is visible on this coronal image. C. The compressed cerebellum bulges anteriorly and partially surrounds the brainstem.


In addition to caudal extension of the inferior cerebellar vermis, Chiari II malformation usually includes inferior displacement of the brainstem into the cervical spinal canal. The brainstem has an elongated configuration and the pontomedullary junction is less distinct than normal. The medulla buckles backward, creating a characteristic “cervicomedullary kink” on sagittal MR images; this is present in approximately 70% of patients with Chiari II malformation. Stretching of the inferior cranial nerves is common. The pons occasionally has a flattened appearance, usually in association with concavity of the clivus. The mesencephalic tectum has a beak-shaped configuration on axial CT and MR images (Figure 14-20). On sagittal images, there is posteroinferior stretching of the quadrigeminal plate. Syringobulbia is an occasional complication of Chiari II malformation.32
The fourth ventricle has an elongated and narrowed configuration in individuals with Chiari II malformation. Occasionally, a trapped (isolated) fourth ventricle occurs as a consequence of aqueductal obstruction in conjunction with obstruction of the outflow foramina or basilar cisterns. Another potential complication is posteroinferior herniation of the fourth ventricle dorsal to the medulla and inferior to the vermis (“encysted fourth ventricle”). Deformities of the cerebral aqueduct are common; aqueductal stenosis or atresia is present in 50% to 75% of patients. A variable degree of enlargement of the lateral ventricles occurs in nearly all patients with Chiari II malformation, often with a colpocephalic pattern. The frontal horns point anteroinferiorly. Substantial enlargement of the third ventricle is uncommon. The massa intermedia is prominent. The septum pellucidum is absent in approximately 40% of individuals with Chiari II malformation; there is a fenestrated appearance in 35% (Figure 14-21).
The cerebral tissue in patients with Chiari II malformation is histologically normal. However, there is a contracted, closely folded pattern of the gyri. After shunting, the medial aspects of the occipital lobes often have multiple small gyri with normal gray matter thickness; this is termed stenogyria. The interhemispheric fissure of patients with Chiari II malformation has an irregular, serrated appearance. Gyri cross the midline and interdigitate through a hypoplastic or fenestrated falx (Figure 14-22). Abnormal development of the corpus callosum occurs in at least 75% of patients with Chiari II malformation. The most common pattern is absence of the rostrum and absence or hypoplasia of the splenium. Enlargement of the caudate heads and massa intermedia is common (Figure 14-23). Gray matter heterotopias occasionally occur.33
Figure 14–23
Chiari II malformation.
A. An axial T1-weighted image of a 10-year-old child shows a markedly enlarged massa intermedia (arrows) extending between the thalami. B. The massa intermedia (arrow) appears as a round structure in the third ventricle on this T2-weighted sagittal image. Additional findings include a fourth ventricle cyst, caudal descent of the cerebellum and brainstem, and scalloping of the clivus.

The Chiari III malformation is exceedingly rare. This term describes a low occipital or high cervical encephalocele in combination with multiple anomalies of the brainstem and cerebellum. Portions of the cerebellum and occipital lobes extend through the calvarial defect, sometimes in conjunction with part of the brainstem. The herniated tissue is gliotic and necrotic; heterotopias may be present. Other potential findings include petrous and clivus scalloping, dysgenesis of the corpus callosum, cerebellar tonsillar herniation, hydrocephalus, and syringohydromyelia.34–36
Holoprosencephaly is a rare disorder of organogenesis, in which there is failure of normal lateral cleavage into distinct cerebral hemispheres, as well as deficient transverse cleavage into a diencephalon and telencephalon. The developmental pathogenesis likely involves defective development of the notochordal plate early in embryogenesis, thereby causing a lack of normal lateral migration of the telencephalic and optic vesicles. There is failure of normal division of the forebrain (i.e., the prosencephalon) between weeks 5 and 6 of embryogenesis, resulting in a complex facial and craniocerebral anomaly of variable severity. The term holoprosencephaly derives from this lack of division of the prosencephalon.
The estimated prevalence of holoprosencephaly is between 1 in 5000 and 1 in 16,000 livebirths. Because many pregnancies with a holoprosencephalic fetus end with spontaneous or elective abortion, the fetal prevalence may be as high as 1 in 250. Holoprosencephaly accounts for at least half of all instances of ventriculomegaly detected on prenatal sonography. Although often an isolated anomaly, holoprosencephaly sometimes occurs in association with various chromosomal syndromes, including trisomy 13, trisomy 15, and trisomy 18. The clinical severity of holoprosencephaly ranges from mild to severe. Common manifestations include apneic episodes during infancy, seizures, and neurological dysfunction. Holoprosencephaly often is associated with combined anterior and posterior pituitary hormone defects; diabetes insipidus is common in these patients. Poikilothermia may occur as a result of hypothalamic hypoplasia. Cardiac, skeletal, genitourinary, and GI anomalies are common with the severe forms of holoprosencephaly.37
Holoprosencephaly comprises a spectrum of anomalies, with 4 generally recognized subtypes: alobar, semilobar, lobar, and syntelencephaly (middle-hemisphere variant) (Table 14-3). Not all patients, however, have features that fit precisely into one of these categories. The alobar and lobar forms represent the most severe and least severe types, respectively. In all forms of holoprosencephaly, the septum pellucidum and the olfactory bulbs are absent and the sylvian fissures are poorly developed. The posterior fossa structures are usually normal. Most patients with holoprosencephaly are normocephalic or microcephalic; approximately 10% have macrocephaly. Facial dysmorphism, such as hypotelorism and midline facial clefts, often occurs in the more severe forms of holoprosencephaly.38
Structure | Alobar | Semilobar | Lobar | Syntelencephaly |
---|---|---|---|---|
Septum pellucidum | Absent | Absent | Absent | Absent |
Rostrum corpus callosum | Absent | Absent | ± | Present |
Body corpus callosum | Absent | Absent | ± | Absent |
Splenium corpus callosum | Absent | Present | Present | Present |
Interhemispheric fissure | Absent | Partial | Partial | Partial |
Falx | Absent | Partial | Partial | Partial |
Frontal horns | Absent | Rudimentary | Squared | Present |
Third ventricle | Absent | Variable | Present | Present |
Site of fusion | Cerebrum | Variable | Inferior frontal | Parietal/Posterior frontal |
There is an anterior-to-posterior gradient in the severity of holoprosencephaly. The corpus callosum is an important marker of brain development in these patients. In general, the severity of brain maldevelopment inversely relates to the degree of corpus callosum development. In the severe forms of the disorder, that is, alobar holoprosencephaly, the corpus callosum is absent. With the lobar and semilobar forms, the posterior aspect of the corpus callosum is intact (Figure 14-24). With syntelencephaly, the callosal genu and splenium are present, and a portion of the callosal body is absent.
Figure 14–24
Semilobar holoprosencephaly.
A. Only the splenium (arrow) of the corpus callosum is present on this sagittal T1-weighted image. Heterotopic gray matter and fused central gray matter structures surround the anterior aspects of the malformed lateral and third ventricles. B. On the axial image, there is fusion of the frontal lobes. The anterior interhemispheric fissure is absent. The fissure is normal posteriorly. There is dilation of the posterior aspect of the fused lateral ventricles, with a batwing configuration.

Barkovich et al propose a grading system for the severity of holoprosencephaly based on the “sylvian angle.” With holoprosencephaly, there is anterior and medial displacement of the sylvian fissures; in severe cases, there are no identifiable sylvian fissures. The sylvian angle is defined as the angle between lines that extend tangential to the sylvian fissures (Figure 14-25). Normal measurements are 12 degrees to 18 degrees. The sylvian angles are largest with the severe forms of holoprosencephaly.39
Figure 14–25
Holoprosencephaly.
The sylvian angle measures 40 degrees in this child with semilobar holoprosencephaly. The sylvian fissures (arrows) are located more anteriorly than normal and there is deficient frontal lobe development. The anterior interhemispheric fissure is absent and there is a common thin gyrus at the midline anteriorly, with fusion of gray matter and white matter structures at the base of this gyrus. There is relatively normal differentiation of the thalami. The occipital lobes are normal in appearance.

Alobar holoprosencephaly is the most severe form of this anomaly. There is absence of a distinct third ventricle on neuroimaging studies; the thalami are fused (i.e., fused diencephalon). There is no interhemispheric fissure, and the falx cerebri is absent or markedly hypoplastic. The cerebrum consists of a thin rim of tissue, which is usually most prominent in the frontal and occipital regions. A crescent-shaped or horseshoe-shaped single ventricle (monoventricle) occupies most of the intracranial cavity. The fused diencephalon indents the inferior aspect of the ventricle. In some patients, the roof of the monoventricle balloons out to form a large dorsal midline cyst; these children are often macrocephalic (Figure 14-26). There is a single midline “azygous” anterior cerebral artery. The internal cerebral veins, straight sinus, and superior sagittal sinus are absent.
Many infants with alobar holoprosencephaly are stillborn or die in infancy. Physical examination typically shows severe midline facial anomalies. Hypotelorism in these infants results from absence or hypoplasia of the premaxillary portion of the face; a severe form results in cyclopia. CT and MRI of the facial structures in patients with alobar holoprosencephaly demonstrates cleft palate, microphthalmia or anophthalmia, trigonocephaly, micrognathia, and absence of the nasal septum. Skull radiographs show a small anterior cranial fossa, superior arching of the orbits, and severe hypotelorism.
Semilobar holoprosencephaly consists of incomplete separation of the prosencephalon into cerebral lobes. The severity of the brain dysmorphism that occurs in the semilobar type of holoprosencephaly is intermediate between that of the alobar and lobar types. Individual patients may have features that overlap the descriptions of these 3 types. There is more cerebral tissue with the semilobar than the alobar type. The thickness of the cerebral cortex is often normal. There are usually small gyri in the anterior aspect of the cerebrum. The sulci in the abnormal cortex are often shallow (Figure 14-27). Occasionally, the cerebral tissue in the anterior/inferior aspect of the cerebrum appears as a mushroom-shaped mass. Subcortical heterotopic gray matter that crosses the midline in the anterior aspect of the cerebrum can occur in association with semilobar holoprosencephaly (Figure 14-28).
A single ventricle is present with semilobar holoprosencephaly, but there is rudimentary formation of the frontal and temporal horns. The posterior aspect of the monoventricle is usually somewhat dilated, and sometimes has a batwing character (see Figure 14-24). In some patients, the monoventricle communicates with a dorsal cyst (Figure 14-29). There is at least partial formation of the posterior aspect of the interhemispheric fissure. The posterior portion of the falx is usually present, but it may deviate from the midline.
Figure 14–29
Severe semilobar holoprosencephaly.
A. A coronal ultrasound image shows fusion of central gray matter structures into a midline mass (arrow). There is no separation of the malformed lateral ventricles at this level. The frontal lobe white matter is fused deep to a foreshortened interhemispheric fissure. B. There is a dorsal cyst (arrow) on this image of the posterior aspect of the brain.

There is a variable degree of third ventricular development in patients with semilobar holoprosencephaly. There is also a spectrum of deep gray matter separation, ranging from fusion into a common mass to near-complete separation of the thalami and basal ganglia. The temporal horns are rudimentary, and hippocampal gyrus formation is generally incomplete. As with all forms of holoprosencephaly, the septum pellucidum is absent and the lateral ventricles are fused. The splenium of the corpus callosum is present in patients with semilobar holoprosencephaly. Important markers for assessing the severity of semilobar holoprosencephaly include the degree of corpus callosum formation, the anterior extent of interhemispheric fissure formation, and the severity of central gray matter fusion. In most children with semilobar holoprosencephaly, the facial structures are normal; mild facial anomalies (cleft lip or cleft palate) occasionally occur.
Lobar holoprosencephaly is the least-severe type. There is usually a small area of midline cerebral fusion along the inferior aspect of the frontal lobes. There is at least partial formation of the interhemispheric fissure and falx cerebri; however, the anterior aspect of the falx is usually dysplastic. The septum pellucidum is absent. The hippocampal formations are nearly normal. There is less distortion of temporal horn and third ventricular morphology than with the alobar and semilobar forms. Moderate ventriculomegaly is typical. The frontal horns typically have a “squared off” appearance. The sylvian fissures are absent or small, and the frontal lobes are often hypoplastic. The corpus callosum may or may not be present. Lobar holoprosencephaly is distinguished from septooptic dysplasia by visualization of a normal falx cerebri in the latter. The facial bones are normal in patients with lobar holoprosencephaly.
The middle interhemispheric variant of holoprosencephaly, also termed syntelencephaly, refers to fusion of the parietal lobes and posterior aspects of the frontal lobes. There appears to be an association with a mutation in the ZIC2 gene, which is important for neural tube closure and embryonic roof plate differentiation. Neuroimaging evaluation demonstrates continuity of the mid portions of the cerebral hemispheres and a common ventricle. The interhemispheric fissure is absent at the site of fusion, but intact between the occipital lobes and the anterior aspects of the frontal lobes. There is often abnormal connection of the sylvian fissures across the midline over the vertex. The body of the corpus callosum is absent, while the genu and splenium are preserved. The basal portions of the frontal lobes are normal in appearance. The basal ganglia are normal. Heterotopic gray matter or dysplastic cerebral cortex is common.40–42
Septooptic dysplasia (SOD) consists of hypoplasia of the optic nerves and absence of the septum pellucidum. Hormonal deficiencies and a variety of forebrain defects can occur in these patients. Although SOD is generally sporadic, there are unusual familial cases. The pathogenesis is likely multifactorial, involving contributions from environmental factors as well as abnormalities of crucial developmental genes such as HESX1, SOX2, SOX3.43,44
Nystagmus and/or diminished visual acuity are sometimes present in patients with SOD. Ophthalmological examination shows hypoplasia of the optic discs. Endocrine deficiencies related to pituitary or hypothalamic dysfunction occur in about two-thirds of patients with SOD. These children may exhibit manifestations of growth hormone deficiency, hypogonadotropic hypogonadism, central diabetes insipidus, hypothyroidism, or adrenal insufficiency. Of those patients with an endocrinopathy, more than half have anterior pituitary hormone deficiency and approximately 10% have diabetes insipidus. Deficiencies of adrenocorticotropic hormone and gonadotrophin can also occur; these may lead to neonatal hypoglycemia or genital anomalies. Endocrinopathy occasionally occurs in patients with optic nerve hypoplasia (unilateral or bilateral) despite the presence of an intact septum pellucidum.45,46
Structure | Imaging finding |
---|---|
Septum pellucidum | Aplasia or hypoplasia |
Optic nerves, chiasm, tracts | Small |
Frontal horns | Box-like |
Neuroimaging studies of patients with SOD show hypoplasia or aplasia of the septum pellucidum (Figure 14-30). This is associated with “box-like” frontal horns on coronal images. Sagittal MR images show low positions of the fornices. The optic nerves, chiasm, and optic tracts usually appear small; however, normal imaging morphology of these structures does not exclude the diagnosis. Optic nerve hypoplasia in patients with SOD is usually bilateral. Some patients with SOD have absence of the septum pellucidum in association with cerebral white matter hypoplasia and normal cerebral cortex. Others have disorders of neuronal organization, such as schizencephaly and gray matter heterotopia; this is termed SOD-plus (Figure 14-31). Posterior pituitary ectopia or absence of the pituitary stalk occasionally occurs in association with SOD. Other reported morphological abnormalities of the brain in these patients include cerebellar hypoplasia, dysgenesis of the corpus callosum, aplasia of the fornix, and empty sella turcica.47–50
Arhinencephaly is a rare anomaly in which there is deficient development of components of the olfactory system (i.e., olfactory aplasia). Although occasionally isolated, arhinencephaly usually occurs in association with other cranial and facial anomalies, such as holoprosencephaly, dysgenesis of the corpus callosum, cleft palate, ocular/orbital deformities, ventral forebrain anomalies, and hypoplasia or aplasia of the nose (arhinia). Patients with bilateral arhinencephaly lack olfactory sensation (anosmia). Coronal MR images show absence or hypoplasia of the olfactory bulbs and olfactory tracts (Figure 14-32). The olfactory sulcus is absent. The gyrus rectus and orbital gyrus form a common gyrus. MR and CT examinations are also important for the detection of associated intracranial and facial anomalies.51
Figure 14–32
Arhinencephaly.
A, B. T2-weighted coronal MR images of a 6-year-old child with anosmia show bilateral gyrus rectus and orbital gyrus fusion (arrow); the olfactory sulcus is absent. The olfactory tracts and olfactory bulbs are absent bilaterally. C. An image of a normal child shows the olfactory bulbs (large arrows) at the cribriform plates, surrounded by cerebrospinal fluid. There is normal gyrus rectus, orbital gyrus, and olfactory sulcus development (small arrows).


Kallmann syndrome is a hereditary anomaly in which aplasia or hypoplasia of components of the olfactory system occurs in patients with hypogonadism and anosmia or hyponosmia. This is a localized neuronal migration anomaly of cells from the embryonic olfactory placode into the forebrain. Proper migration of these cells is required for development of the olfactory system. In addition, cells from the olfactory placode normally migrate to the hypothalamus where they differentiate into luteinizing hormone-releasing hormone cells that control adenohypophyseal release of follicle-stimulating hormone and luteinizing hormone. KAL1 or FGR1 mutations are responsible for most instances of Kallmann syndrome.52
High-resolution coronal MR images of patients with Kallmann syndrome show small or absent olfactory sulci and absent olfactory bulbs. The olfactory tracts are usually hypoplastic. In some patients, there are small irregular “nodules” ventral to the frontal lobes, apparently representing dysplastic tissue caused by deficient neuronal migration. In patients with severe functional impairment of the hypothalamus, the pituitary gland is small. Kallmann syndrome is sometimes associated with renal anomalies or facial anomalies, such as cleft palate, dental agenesis, and mandibular deformities.53,54
Dysgenesis of the corpus callosum is a common developmental lesion of the brain. This anomaly ranges from a small posterior callosal defect to complete agenesis. In general usage, the term dysgenesis refers to the spectrum of developmental lesions of the corpus callosum. Agenesis indicates complete absence and hypoplasia indicates partial failure of development; the misnomer “partial agenesis of the corpus callosum” refers to the latter instance. Interference with normal development of the corpus callosum can occur as a consequence of hereditary factors, chromosomal disorders (8, 9, 13, and 18), fetal exposure to exogenous teratogens (e.g., alcohol), and metabolic disorders (e.g., peroxisomal disorders and nonketotic hyperglycinemia). The estimated prevalence of agenesis of the corpus callosum in the general population is 0.2% to 0.7%. A population-based study from California demonstrated a prevalence of 1.8 per 10,000 livebirths.55,56
Most patients with callosal agenesis suffer neurodevelopmental manifestations of the anomaly, although there is substantial individual variation in severity. More than 80% of children with agenesis of the corpus callosum have clinically detectable neurological manifestations such as macrocephaly, epilepsy, developmental delay, and various forms of neurological disability. Many patients with callosal agenesis or hypoplasia have additional CNS anomalies or a genetic syndrome; for example, holoprosencephaly, intracranial lipoma, interhemispheric cyst, migrational disorders, cephalocele, craniofacial anomalies, Toriello-Carey syndrome, Aicardi syndrome, Chiari II malformation, orofacial-digital syndrome, Rubinstein-Taybi syndrome, Dandy-Walker malformation, and frontonasal dysplasia.57–61
The initial phase of corpus callosum development is at approximately the seventh week of gestation, with the formation of callosal precursors along the ventral aspect of the embryonic commissural plate and cortical fibers. The callosal precursors secrete the chemoattractant axonin-1 to guide the developing axons across the midline, thereby causing each region of the hemispheric fibers to connect to the contralateral side through the corpus callosum. The corpus callosum develops in a sequential fashion: the genu develops first, then the body, the splenium, and lastly the rostrum. Normally, the corpus callosum is structurally complete by the 20th week of gestation. The corpus callosum is the largest of the cerebral commissures; the anterior commissure and the hippocampal commissure also derive from the embryonic commissural plate.
True agenesis of the corpus callosum usually is the result of failure of formation of the commissural plate. Deficiencies of the other cerebral commissures sometimes accompany dysgenesis of the corpus callosum. Because of the developmental sequence of the corpus callosum, partial deficiencies most often involve the portions that are formed later during embryogenesis; that is, the rostrum, splenium, and posterior portion of the body. Exceptions to this pattern include some forms of holoprosencephaly and focal cerebral lesions that interfere with embryonic axonal migration across the midline. Also, acquired destructive abnormalities of the corpus callosum in the fetus or child can involve any portion of the structure. With agenesis of the corpus callosum, hemispheric axons fail to cross the midline and instead course longitudinally to form paramedian bundles along the medial borders of the lateral ventricles; that is, the bundles of Probst.
The normal corpus callosum appears on neuroimaging studies as a C-shaped midline structure. The rostrum is the small anterior inferior portion. The anterior curved portion is in the genu. The body is the longest portion of the structure; the isthmus is an area of slight thinning in the dorsal aspect of the body. The slightly expanded posterior aspect of the corpus callosum is the splenium. The hippocampal commissure crosses between the bodies of the fornices, adjacent to the splenium. The anterior commissure is located in the anterior wall of the third ventricle.
Prenatal detection of corpus callosum anomalies is possible with sonography and MR. The corpus callosum in the normal fetus reaches adult form by 18 to 20 weeks’ gestation. Coronal and midline sagittal images are most useful for depicting the integrity of the fetal corpus callosum. Supplemental imaging findings of hypoplasia or agenesis include enlarged atria and occipital horns, teardrop configurations of the lateral ventricles, absence of the cavum septum pellucidum, superior extension of the third ventricle, and radiating medial sulci.62,63
Structure | Imaging finding |
---|---|
Corpus callosum | Absent |
Lateral ventricles | Separated and parallel lateral ventricular bodies Enlarged atria and occipital horns |
In infants and older children with agenesis of the corpus callosum, the lateral ventricles have a separated and parallel appearance on axial images because of the interposed bundles of Probst. The third ventricle extends superiorly between the lateral ventricles, into the interhemispheric fissure. Colpocephaly is usually present; that is, enlargement of the atria and occipital horns. The medial borders of the frontal horns are somewhat concave because of indentation by the bundles of Probst (Figure 14-33). Coronal and sagittal images provide optimal evaluation of the corpus callosum and are essential for the detection and characterization of callosal hypoplasia (Figure 14-34). With hypoplasia, the most common imaging pattern is absence of the rostrum, inferior aspect of the genu, the splenium, and a variable segment of the posterior aspect of the body. With agenesis, sagittal images often show a “cartwheel” or “sunburst” appearance of the interhemispheric sulcal markings, as a result of lack of inversion of the cingulate gyri (Figures 14-35 and 14-36). Normal cingulate sulci are lacking. Hypoplasia of the hippocampi is associated with enlargement of the temporal horns (Figure 14-37). Reduced cerebral hemispheric white matter volume and malformations of cortical development are common additional neuroimaging findings in patients with dysgenesis of the corpus callosum.64,65
Figure 14–37
Agenesis of the corpus callosum.
A coronal T1-weighted MR image of a 10-month-old infant shows superior extension of the third ventricle into the interhemispheric fissure; the wall of the ventricle is too small to be visualized. The bundles of Probst (arrows) cause lateral and inferior displacement of the frontal horns. The temporal horns are prominent.

Diffusion-tensor MR and MR fiber tractography can demonstrate the abnormal orientation of white matter fibers in patients with corpus callosal anomalies. With agenesis of the corpus callosum, hemispheric cortex fibers fail to cross the midline and instead form the paramedian Probst bundles. The Probst bundles are longitudinally oriented fibers adjacent to the medial walls of the lateral ventricles. There is fusion of the Probst bundles with the rudimentary cingulum and dysplastic fornices. In patients with hypoplasia of the corpus callosum, diffusion-tensor MR shows extensive fiber connections through the intact portion of the corpus callosum. The white matter fibers from the parietooccipital regions form a back-to-front bundle and enter into the remaining portion of the genu. Frontal lobe fibers also join the connection through the partially formed corpus callosum; these form an H-shaped configuration of hemispheric fibers on axial views.66
There are various acquired causes of thinning of the corpus callosum that should not be mistaken for dysgenesis. Focal or diffuse thinning because of neonatal hemorrhage, head trauma, ischemia, or hydrocephalus is most common. A variable degree of thinning often occurs in association with various developmental brain lesions, such as Chiari II malformation. Focal thinning of the corpus callosum is common in children with hereditary spastic paraplegia.
Complete or partial absence of the corpus callosum sometimes occurs in association with a large midline ventricular diverticulum or interhemispheric cyst(s). Hydrocephalus is common with both forms of this disorder. The latter form often has multiloculated cysts and is sometimes associated with subcortical heterotopia, subependymal heterotopia, or polymicrogyria. Some of these patients have manifestations of Aicardi syndrome.67,68
Imaging studies of callosal dysgenesis with an interhemispheric diverticulum demonstrate a large interhemispheric fluid collection that widely communicates with the dilated third and/or lateral ventricles (Figure 14-38). The findings are similar with the cystic form of this anomaly except that thin walls separate the cysts from the ventricles.
Figure 14–38
Callosal dysgenesis with interhemispheric diverticulum.
A. A T1-weighted sagittal MR image of a 1-year-old child with macrocephaly and hydrocephalus shows a large midline fluid collection. Only the genu and anterior aspect of the body of the corpus callosum are present. B. The diverticulum extends along the left side of the interhemispheric fissure. It is contiguous with the lateral ventricles and third ventricle. C. The dilated left lateral ventricle widely communicates with the diverticulum.


The cysts may be slightly hyperintense to clear CSF on T1-weighted MR images and hyperintense on T2-weighted images, as a result of proteinaceous fluid or subacute hemorrhage. The cysts usually bulge superiorly along one or both sides of the interhemispheric fissure (Figure 14-39).69
Figure 14–39
Callosal agenesis with an interhemispheric cyst.
A. There are multiple midline cysts on this enhanced T1-weighted image. The corpus callosum is absent. B. A coronal fluid-attenuated inversion recovery (FLAIR) image shows one of the cysts to extend superiorly along the left side of the interhemispheric fissure. There is dilation of the right lateral ventricle.

Hypoplasia of the corpus callosum is often present in patients with a lipoma of the adjacent portion of the interhemispheric fissure. Interhemispheric fissure lipoma in association with callosal dysgenesis occurs with a frequency of approximately 1 per 1700 livebirths. Closure defects such as encephalocele are common in these patients. Cutaneous lipomas may also be present. Lipomas are hypoattenuating on CT and hyperintense on T1-weighted MR (Figure 14-40). There is considerable variation between patients in the size and morphology of interhemispheric lipomas and the severity of associated callosal dysgenesis (Figure 14-41). Substantial corpus callosum deficiency is common with a large round or oval lipoma. The corpus callosum often is nearly normal in patients with a thin curvilinear lipoma along the splenium. Occasionally, there is intraventricular extension of an interhemispheric lipoma via the choroid plexus.70,71
Aicardi syndrome is a rare X-linked syndrome that only occurs in females. The causative mutation is apparently lethal for male embryos. The major clinical features of Aicardi syndrome include chorioretinal lacunae (100% of patients), dysgenesis of the corpus callosum (100% of patients; agenesis in 72% and hypoplasia in 28%), infantile spasms, cerebral cortical heterotopias (50%), cortical dysplasia, and costovertebral defects (39%). The callosal anomaly in these patients frequently includes interhemispheric cysts. Other potential neuroimaging findings in patients with Aicardi syndrome include cerebellar hypoplasia, choroid plexus papilloma, posterior fossa cyst, microphthalmia, and polymicrogyria.72–74
Hereditary spastic paraplegia comprises a clinically and genetically heterogeneous group of neurodegenerative disorders. A subtype of autosomal recessive hereditary spastic paraplegia is associated with thinning of the corpus callosum. The thinning apparently represents atrophy rather than hypoplasia. The major clinical features include progressive spastic paraplegia, dementia, muscle rigidity, and cerebellar ataxia. The clinical onset is usually during the second decade of life. MR shows a thin, but otherwise intact, corpus callosum. Thinning is most prominent in the anterior portion. White matter signal abnormality may also be present.75
Microcephaly is a nonspecific term for an abnormally small head. Microencephaly refers to subnormal brain volume. The usual clinical definition is an occipital–frontal head circumference that is greater than 2 standard deviations below the normal mean adjusted for age and gender. Some clinicians use a cutoff measurement of 3 standard deviations. The most common causes of microcephaly are severe postnatal brain destruction, metabolic brain disease, and neurosurgical procedures that substantially diminish the volume of brain or ventricular fluid. Various forms of brain hypoplasia can also lead to congenital microcephaly. Potential mechanisms for brain hypoplasia include maternal alcoholism, fetal irradiation, intrauterine infection, maternal undernutrition, and various chromosomal disorders (Table 14-4). There are hundreds of genetic disorders that can cause microcephaly.76,77
Acquired | Hypoxic–ischemic encephalopathy |
Status post shunting of hydrocephalus | |
Intrauterine infection | |
Fetal teratogen exposure (e.g., alcohol) | |
Maternal phenylketonuria | |
Poorly controlled maternal diabetes | |
Fetal irradiation | |
Genetic | Autosomal recessive microcephaly |
Microcephaly with simplified gyral pattern | |
Autosomal dominant microcephaly | |
X-linked microcephaly | |
Trisomies 13, 18, 21 | |
4p Deletion syndrome | |
Williams syndrome | |
Lissencephaly | |
Cornelia de Lange syndrome | |
Smith-Lemli-Opitz syndrome | |
Lymphedema, microcephaly, chorioretinopathy syndrome |
Primary microcephaly includes conditions in which failure of brain development is caused by a genetic abnormality. Secondary microcephaly indicates the involvement of an extrinsic insult that interferes with brain development or causes brain damage. Microcephaly that occurs in utero can be primary or secondary. The term isolated microcephaly indicates a lack of associated anomalies. These classification schemes are imprecise, and not all children have findings that allow accurate differentiation between primary versus secondary mechanisms.
Autosomal recessive primary microcephaly is a spectrum of disorders in which there is subnormal size of the CNS, particularly the cerebral cortex. In some of these patients, the brain architecture is normal aside from being small. Others, including those described below with a simplified gyral pattern, have focal or generalized brain anomalies. The phenotype includes a receding forehead and a normal-size face. The responsible genes apparently regulate mitosis of progenitor cells in the neuroepithelium that lines the cerebral ventricles. There are also rare autosomal dominant and X-linked forms of primary microcephaly.78,79
Microcephaly with simplified gyral pattern (oligogyric microcephaly) refers to a spectrum of genetic brain anomalies in which the gyri are shallow and diminished in number and there is substantial microcephaly. The thickened cortex of lissencephaly is lacking. The pathophysiology apparently involves subnormal neuronal and glial proliferation in the germinal zones and increased apoptosis (programed cell death). Tertiary sulci fail to form. There is thinning of the cerebral white matter. Severe forms may lead to neonatal death; survivors have developmental delay, intellectual disability, and neurological deficits. Seizures are common.80–82
Barkovich and others divide microcephaly with simplified gyral pattern into 6 groups according to the clinical and neuroimaging features. Group 1 infants are the products of normal pregnancies and have uneventful deliveries. MR demonstrates microcephaly, a paucity of gyri, shallow sulci, normal cortical thickness, and normal myelination. Infants in group 2 often have a history of a breech presentation, develop seizures during the first few days of life, and exhibit limb spasticity and abnormal reflexes as neonates. MR shows a paucity of gyri, shallow sulci, delayed myelination, and preserved cerebral cortical thickness. Group 3 consists of term infants who exhibit spasticity, seizures, and lack of normal reflexes. The abnormalities of the gyri and sulci are more pronounced than in the group 1 and group 2 patients. Additional potential neuroimaging findings include subependymal heterotopias and arachnoid cysts. Infants in group 4 have clinically obvious abnormalities as neonates: abnormal reflexes, seizures, nystagmus, thumb deformities, and hypertonia. The MR appearance is identical to that of group 1. Infants in group 5 and group 6 have lissencephaly. Clinical findings in group 5 include severe microcephaly, hypotonia, abnormal reflexes, seizures, and severe developmental delay. MR shows prominent subarachnoid spaces, severe microencephaly, markedly deficient gyral formation, deficient white matter, and cortical thinning. There is severe neurological impairment of infants in group 6. MR shows agyria and cortical thickening; agenesis of the corpus callosum and cerebellar hypoplasia are common.
Megalencephaly refers to abnormal enlargement of the entire brain in association with clinical manifestations of brain pathology. The pathogenesis may involve overproduction of neurons because of a derangement in neural proliferation. Megalencephaly occurs as a primary isolated condition (idiopathic anatomic megalencephaly), a primary developmental lesion in association with another disorder, or as a secondary phenomenon caused by a metabolic disorder. The isolated form can occur as a familial or sporadic lesion. Primary megalencephaly can be a feature of Beckwith-Wiedemann syndrome, Sotos syndrome, achondroplasia, neurocutaneous syndromes, and various endocrine disorders. Metabolic disorders that can cause secondary megalencephaly include leukodystrophies, mucopolysaccharidoses, sphingolipidoses, and gangliosidoses.83–85
Megalencephaly typically leads to macrocephaly; that is, an occipitofrontal head circumference greater than the 98th percentile. However, most children with macrocephaly do not have megalencephaly. Other causes of head enlargement include hydrocephalus, extraaxial fluid collections, neoplasm, and calvarial thickening.
Macrocephaly is a component of the macrocephaly–capillary malformation syndrome (macrocephaly–cutis marmorata telangiectasia congenita). Cranial prominence in these children is usually a result of brain overgrowth as well as hydrocephalus. The megalencephaly is sometimes asymmetric. Inferior herniation of the cerebellar tonsils occurs in approximately 70% of children with this syndrome, apparently because of progressive enlargement of the cerebellum. There is delay in white matter myelination. Focal cortical dysplasia and polymicrogyria are common. The typical locations of polymicrogyria in children with macrocephaly-capillary malformation syndrome are the perisylvian and insular regions (Figure 14-42). The corpus callosum often has a thickened character. Other findings of this syndrome include somatic overgrowth, hemihyperplasia, extensive cutaneous capillary malformation, polydactyly, syndactyly, and frontal bossing.86,87
Figure 14–42
Macrocephaly-capillary malformation syndrome.
A. A sagittal T1-weighted image at 2 months of age shows a prominent head size relative to the face. There is mild inferior herniation of the cerebellar tonsils. B. Craniofacial disproportion is more pronounced at age 4 years. The tonsillar herniation has increased. The corpus callosum is thick. C. An axial T2-weighted image shows ventriculomegaly and bilateral insular polymicrogyria.


Hemimegalencephaly
Hemimegalencephaly refers to hamartomatous overgrowth of one cerebral hemisphere or some of its lobes. The histopathological features include immature-appearing neurons, astrogliosis, and balloon cells. There is disorganized tissue architecture, as well as manifestations of incomplete cellular maturation. The involved hemisphere is hypertrophied and dysplastic, and contains areas of polymicrogyria, gray matter heterotopia, pachygyria, and white matter gliosis. The anomaly can be focal, lobar, or hemispheric; bilateral cerebral megalencephaly is quite rare. The pathophysiology of hemimegalencephaly apparently involves aberrant cell proliferation; a secondary disturbance of neuronal migration during the first trimester contributes to the disorderly tissue architecture. Hemimegalencephaly likely represents a spectrum of disorders that lead to similar pathophysiological changes in the brain. Involvement of the right cerebral hemisphere is more common than the left.88–90
Common clinical manifestations of hemimegalencephaly consist of the triad of contralateral hemiparesis, psychomotor retardation, and intractable seizures that develop soon after birth. Most often, there is clinically evident macrocephaly during infancy. In some patients, there is ipsilateral hemihypertrophy of all or a portion of the body. Slightly less than half of patients with hemimegalencephaly have an associated syndrome. Hemimegalencephaly is common in patients with epidermal nevus syndrome. Other associated syndromes include tuberous sclerosis, Proteus syndrome, neurofibromatosis type 1, congenital infection (Cytomegalovirus, toxoplasmosis), hypomelanosis of Ito, Klippel-Trenaunay-Weber syndrome, and encephalocraniocutaneous lipomatosis. Individuals with idiopathic hemihyperplasia sometimes have craniofacial and brain asymmetry.91,92
MRI or CT imaging of hemimegalencephaly demonstrates enlargement of all or a large portion one hemisphere. The contralateral hemisphere is usually somewhat smaller than normal; that is, contralateral hemimicrencephaly. In most patients, there is proportionate enlargement of the ipsilateral lateral ventricle. The frontal horn usually has a thin irregular character, and tapers superiorly and anteriorly. The appearance of the ipsilateral cortex is variable. Most often, the cortex is grossly dysplastic, with cortical thickening and deficient sulci (Figure 14-43). There may be enlarged and irregular deep gray matter and white matter structures. The border between the cortex and the subjacent white matter is usually indistinct or obliterated. The deeper white matter may be heterogeneously hyperintense on T2-weighted MR images and hypoattenuating on CT; this is due to gliosis, neuronal dysplasia, and heterotopia (Figure 14-44). Occasionally, there is enlargement of the ipsilateral cerebellar hemisphere and brainstem. Other potential findings include ipsilateral olfactory nerve enlargement and cerebral vascular dilation. Fluorodeoxyglucose positron emission tomography imaging indicates glucose hypometabolism in the ipsilateral hemisphere. Proton MR spectroscopy demonstrates diminished glutamate and N-acetyl aspartate (NAA) peaks.93–98
The neurophakomatoses (also termed phakomatoses or neurocutaneous syndromes) comprise a clinically heterogeneous group of congenital disorders that have the common patho-physiological mechanism of anomalous development of neuroectodermal derivatives (Table 14-5). There is variable involvement of the nervous system, skin, and eyes. Visceral organs can also be involved. The most clinically important phakomatoses are neurofibromatosis type 1, neurofibromatosis type 2, tuberous sclerosis, Sturge-Weber syndrome, von Hippel-Lindau syndrome, and ataxia-telangiectasia. The most common phakomatosis is neurofibromatosis type 1.99,100
Neurofibromatosis type 1 | Neurofibromas, optic glioma, focal skeletal dysplasia |
Neurofibromatosis type 2 | Schwannomas (acoustic neuromas) |
Tuberous sclerosis | Hamartomatous lesions in multiple organ systems |
Sturge-Weber syndrome | Angiomatosis of the leptomeninges, face, and choroid of the eyes |
von Hippel-Lindau disease | Retinal angiomas, CNS hemangioblastoma, cysts/neoplasms of abdominal organs, epididymal cystadenomas |
Ataxia-telangiectasia | Mucocutaneous and ocular telangiectasias, cerebellar atrophy |
Neurocutaneous melanosis | Cutaneous pigmented nevi, deposits of melanin in the leptomeninges and brain |
Epidermal nevus syndrome | Epidermal nevi, dysplasias/deformities of the CNS, eyes, skin, skeleton, and heart |
Incontinentia pigmenti | Skin eruptions, retinal vascular lesions, dental anomalies, multifocal/diffuse brain atrophy |
Hypomelanosis of Ito | Skin lesions, musculoskeletal deformities, ± brain anomalies |
Chediak-Higashi syndrome | Abnormal pigmentation of skin and hair, immunodeficiency, brain atrophy, white matter lesions |
PHACES syndrome | Posterior fossa malformations, hemangiomas, arterial anomalies, cardiac anomalies, aortic coarctation, eye abnormalities, sternal cleft or suprapubic raphe |
Neurofibromatosis type 1 (NF-1) is a multisystem disorder that predominantly results in CNS lesions, skin lesions, and focal areas of mesodermal dysplasia. The hallmark lesion of this disorder is the peripheral neurofibroma. NF-1 is an autosomal dominant genetic disorder that is caused by a mutation of chromosome 17. The penetrance is high, but the expressivity is variable. Approximately half of cases are a result of new mutations. The NF-1 tumor-suppressor gene encodes the protein neurofibromin. Among other functions, such as tumor suppression, this protein is apparently required for normal myelination by Schwann cells. The prevalence of NF-1 is 1 in 3000 individuals.99,101
Neurofibromatosis type 1 has great variability in clinical expression. Some patients have very mild manifestations, whereas others are severely affected. At least 95% of NF-1 patients have cutaneous involvement. The characteristic skin lesions of neurofibromatosis type 1 include café-aulait spots (with smooth outlines), freckling, and cutaneous neurofibromas. The café-au-lait spots are usually detectable during the first year of life. Cutaneous neurofibromas and iris Lisch nodules most often become evident during the second and third decades of life. Children with NF-1 have 200 to 500 times the normal risk of developing leukemia compared to the general population. Table 14-6 outlines the diagnostic criteria for NF-1.102
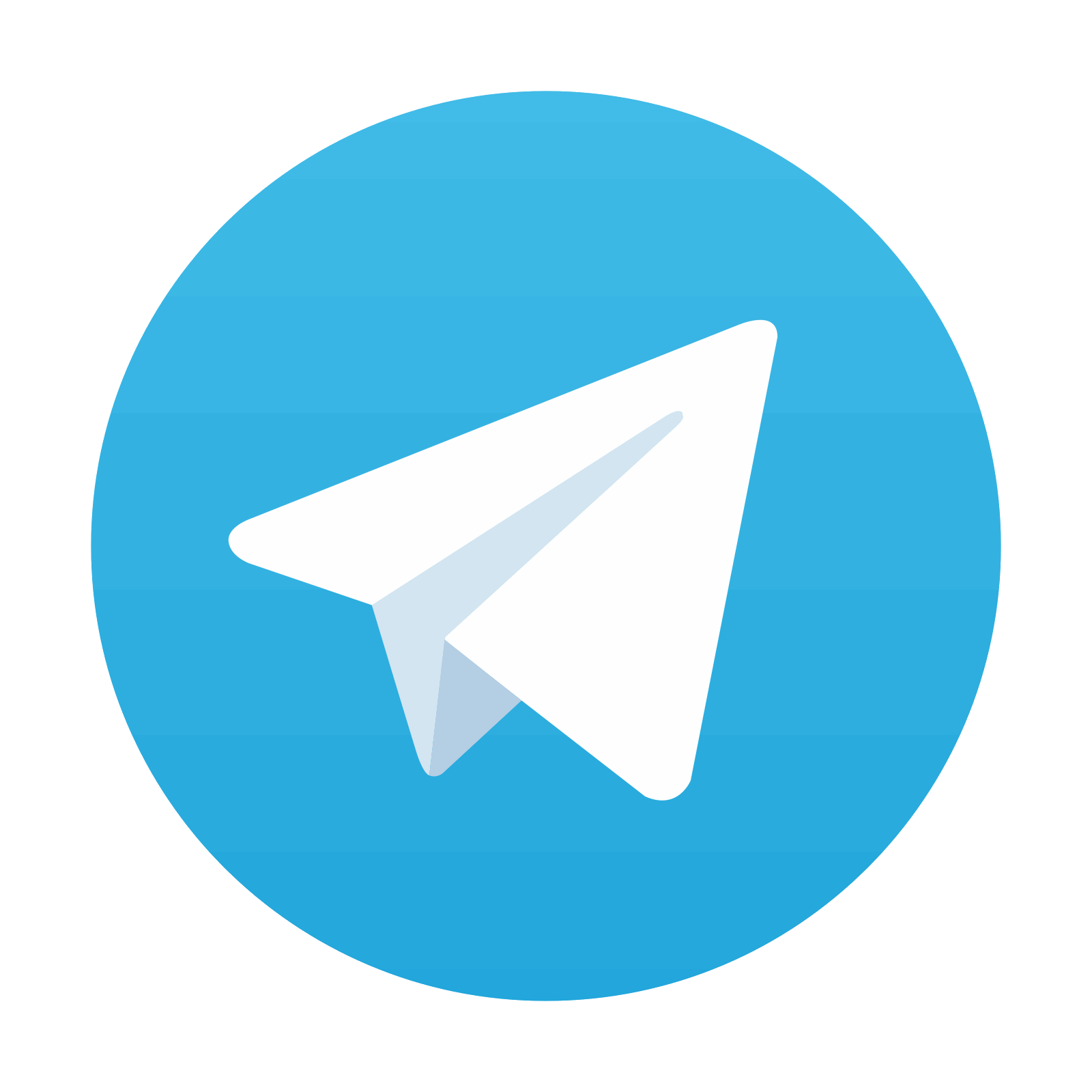
Stay updated, free articles. Join our Telegram channel

Full access? Get Clinical Tree
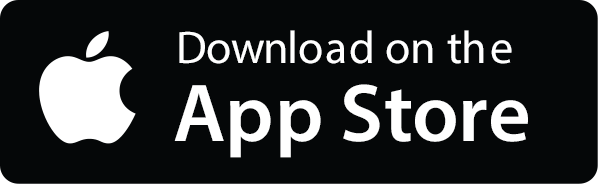
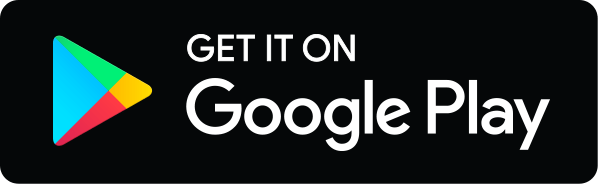