Objective
The purpose of this study was to investigate the impact of chronic hypoxia on the nitric oxide synthase isoenzymes in specific brain structures.
Study Design
Time-mated pregnant guinea pigs were exposed to 10.5% molecular oxygen for 14 days (animals with chronic fetal hypoxia; HPX) or room air (control animals; NMX); L-N6-(1-iminoethyl)-lysine ( L-NIL ; an inducible nitric oxide synthase inhibitor, 1 mg/kg/d) was administered to HPX group for 14 days (L–NIL + HPX). Fetal brains were harvested at term. Multilabeled immunofluorescence was used to generate a brain injury map. Laser capture microdissection and quantitative polymerase chain reaction were applied; cell injury markers, apoptosis activation, neuron loss, total nitric oxide, and the levels of individual nitric oxide synthase isoenzymes were quantified.
Results
Chronic hypoxia causes selective fetal brain injury rather than global. Injury is associated with differentially affected nitric oxide synthases in both neurons and glial cells, with inducible macrophage-type nitric oxide synthase up-regulated at all injury sites. L-NIL attenuated the injury, despite continued hypoxia.
Conclusion
These studies demonstrate that chronic hypoxia selectively injures the fetal brain in part by the differential regulation of nitric oxide synthase isoenzymes in an anatomic- and cell-specific manner.
Fetal brain abnormalities (whether developmental or acquired) contribute to numerous postnatal disorders that range from learning disability to severe neurologic handicap. On the severe end, cerebral palsy (CP) complicates 1.5-6 of every 1000 deliveries and is associated independently with spontaneous preterm birth, maternal-perinatal infection-inflammation, and intrauterine growth restriction (IUGR),which is a frequent surrogate for chronic fetal hypoxia. The fact that other central nervous system abnormalities are common in CP suggests that CP is a phenotype more than a specific injury. The estimated lifetime excess cost of CP and CP-associated disorders per individual exceeds $1.5 million. The proportion of CP that generally is attributed to acute intrapartum ischemia-reperfusion approximates 15% and reflects the assumption that the intrapartum event was causative. Yet many affected newborn infants have other antecedents of CP that include prematurity, chorioamnionitis, IUGR, or structural malformation ; and the combination of intrauterine asphyxia and inflammation dramatically increases the risk of CP. In one of the few studies of the presumably chronically hypoxic fetal brain, 43% of perinates who either died in utero or shortly after birth had evidence of placental vascular problems such as funisitis and antemortem brain injury that included gliosis and neuronal necrosis. Such changes could not result from an intrapartum event because there was inadequate elapsed time before death to account for the findings. This body of information suggests that acute intrapartum ischemia-reperfusion in isolation is an uncommon cause of CP. It is also suggests that inflammation (funisitis and gliosis) is part of the human phenotype and is consistent with the clinical fact that early delivery of the chronically hypoxic fetus does not improve neurodevelopmental outcome.
The associations between intrauterine infection/inflammation and developmental brain injury have been studied extensively. Interleukin-1 and -6 are central to the pathogenesis of white matter injury that is mediated by intrauterine infection. Tumor necrosis factor–α in the setting of intrauterine infection is associated with neonatal intraventricular hemorrhage, periventricular leukomalacia, and the subsequent development of CP. Thus, it is reasonable to speculate that inflammation plays a role in the pathogenesis of perinatal brain injury. We demonstrated that chronic hypoxia increases interleukin-6 and tumor necrosis factor–α in the fetal blood of guinea pig, which fulfills the diagnostic criteria in humans for fetal inflammatory response syndrome. This inflammatory response is associated highly with both spontaneous preterm birth and subsequent neurodevelopmental compromise. Chronic hypoxia also increased the expression of these cytokines in multiple fetal organs, which included the brain. Although there is extensive evidence that links acute perinatal ischemia to proinflammatory cytokine and reactive oxygen species generation, no such association has been identified for the more common clinical condition that is associated with neurodevelopmental compromise, chronic fetal hypoxia, due to placental dysfunction.
Nitric oxide (NO) is involved in diverse physiologic and pathologic processes. A decade ago, we documented that chronic hypoxia altered the expression of endothelial neuronal nitric oxide synthase (eNOS) and neuronal nitric oxide synthase (nNOS) in the ovine fetal cerebrum. Our finding that chronic fetal hypoxia decreased eNOS, but increased nNOS, suggested the normal fetal response to chronic hypoxia could be actually maladaptive because the lack of eNOS-derived NO might prolong an episode of ischemia, while the increased nNOS could prove cytotoxic by increasing the release of excitatory amino acids. Recently, Haynes et al applied immunocytochemistry to archived human tissue and observed a significant increase in the density of inducible nitric oxide synthase (iNOS)–expressing cells in the cerebral white matter of cases with periventricular leukomalacia, compared with control. This study supports an important role for iNOS-induced nitrosative stress in the reactive/inflammatory component of periventricular leukomalacia (PVL). Herein, we test the hypothesis that fetal brain injury that is the result of chronic hypoxia is selective rather than global and that the anatomic sites of injury reflect altered expression of ≥1 NOS isoform.
Materials and Methods
Animal model
Female Duncan-Hartley guinea pigs (term, approximately 65 days; Charles River Laboratories International, Inc, Wilmington, MA) were time-mated; dams were assigned randomly to either room air (approximately 21% O 2 [control animals: NMX group; n = 6) or to an environmental chamber that contained 10.5% O 2 for 14 days (animals with chronic fetal hypoxia [HPX group]; n = 6) beginning days 46-49 (approximately 0.7 gestation). Normal guinea pig chow was provided without restriction. On days 60-64, a hysterotomy was performed aseptically while dams respired spontaneously (ketamine [80 mg/kg] and xylazine [1 mg/kg]), and the fetal brains were collected into either formalin for histologic study as described later, or were frozen in liquid nitrogen for gene quantification. Only the presenting pup was studied. We have shown in the past that the hypoxic dams gain weight normally throughout gestation and undergo labor and deliver without event and that the pups are reduced in size but have increased hemoglobin and hypoxia inducible factor measurements. To test the role of iNOS on fetal brain injury, L-N6-(1-Iminoethyl)-lysine (L-NIL), which is a selective pharmacologic inhibitor of iNOS known to cross the placenta was administered to HPX animals in their drinking water (1 mg/kg/d, L-NIL+HPX; n = 6) for 14 days.
Fetal brain frozen section preparation
The fresh fetal whole brains from NMX animals (n = 6), HPX animals (n = 6), or L-NIL+HPX animals (n = 6) were removed and fixed in 4% paraformaldehyde overnight at 4°C, cryoprotected in 30% sucrose at 4°C for 2-3 days until no longer buoyant, and then embedded in optimal cutting temperature compound for sectioning. Five sets of coronal mirror sections were prepared for 3,3′-diaminobenzidine–based immunostaining, multilabeled fluorescence immunostaining, terminal deoxynucleotidyl transferase dUTP nick end labeling (TUNEL) staining, Nissl staining, and laser capture microdissection (LCM). The coronal sections were made at the interaural level of 6.72-5.40 mm (bregma: from –2.28 to –3.60 mm) of the fetal brain, cut on a cryostat at 8-μm thickness, with each mirror section cut at 10-μm intervals before being stored at –20°C for later study. These sections did not include the cerebellum and brainstem, which will be studied in the future.
Fetal brain cell injury detection by immunohistochemistry
The antibodies are described in Table 1 .
Application | Primary antibodies | Species | Dilution | Secondary antibodies |
---|---|---|---|---|
Injury Index Mapping | Anti-GFAP (Sigma) | Mouse | 100 | Universal LSAB + System-HRP (DAKO) |
Anti-ATF3 (Sigma) | Mouse | 50 | Universal LSAB + System-HRP (DAKO) | |
ATF3 Colocalization | Anti-ATF3 (Sigma) | Rabbit | 200 | Donkey anti rabbit + FITC (Jackson) |
Anti-GFAP (Sigma) | Mouse | 500 | Donkey anti mouse + Red-X (Jackson) | |
Anti-β-Tubulin III (Sigma) | Mouse | 1000 | Donkey anti mouse + Red-X (Jackson) | |
NOSs Colocalization | Anti-iNOS (BD bioscience) | Mouse | 200 | Donkey anti mouse + Red-X (Jackson) |
Anti-ATF3 (Sigma) | Rabbit | 200 | Donkey anti rabbit + FITC (Jackson) | |
Anti-GFAP (Sigma) | Rabbit | 200 | Donkey anti rabbit + FITC (Jackson) | |
Anti-nNOS (Cell signaling) | Rabbit | 100 | Donkey anti rabbit + FITC (Jackson) | |
Anti-ATF3 (Sigma) | Mouse | 200 | Donkey anti mouse + Red-X (Jackson) | |
Anti-GFAP (Sigma) | Mouse | 500 | Donkey anti mouse + Red-X (Jackson) |
The first set of mirror slides was immunostained with specific antibodies against activating transcription factor 3 (ATF3), a marker of neuronal injury, and glial fibrillary acidic protein (GFAP), a marker of glial activation. The Universal Dako LSAB immunohistochemistry staining assay (Dako North America, Inc, Carpinteria, CA) was used for signal detection. The fetal brain sections were incubated at room temperature in 3% hydrogen peroxide for 5 minutes and then rinsed with phosphate-buffered saline solution. Primary antibodies ( Table 1 ) to ATF3 (mouse, 1:50; Sigma Chemical Company, St. Louis, MO) and GFAP (mouse, 1:100; Sigma Chemical Company) were applied overnight at 4°C, labeled with streptavidin-biotin, and developed in 3,3′-diaminobenzidine to produce brown staining. Hematoxylin was used as a counterstain. Histologic quantification was performed as described later.
Colocalization of NOS isoforms with cell injury by multilabeled immunofluorescence histochemistry staining
The second set of mirror slides was used for protein colocalization studies by multilabeled immunofluorescence histochemistry with the primary antibodies ( Table 1 ). For ATF3 colocalization, β-tubulin III and GFAP were used to label neurons and glial cells, respectively. The primary antibodies for ATF3 and GFAP or ATF3 and β-tubulin III were applied to the slides and incubated overnight at 4°C with gentle agitation. The sections then were washed 5 times for 10 minutes in phosphate buffered saline solution, incubated in darkness with the specific secondary antibodies, and rinsed 5 times for 10 minutes in phosphate-buffered saline solution before being mounted with 4,6-diamino-2-phenylindole and coverslipped. Protein expression was identified under the appropriate specific fluorescence wavelengths.
For the NOS colocalization studies, the injured areas were first detected with either ATF3 or GFAP, and then followed by nNOS or iNOS (primary antibodies and specific secondary antibodies; Table 1 ). The staining procedure was the same as described earlier. The primary antibodies were omitted as a negative control.
Detection of apoptotic cells
The third set of mirror slides was used to identify apoptotic cells on the basis of the presence of DNA strand breaks with the use of TUNEL. Frozen fetal brain sections were used for TUNEL staining by the Fluorescent in Situ Cell Death Detection Kit (Roche Diagnostics Corporation, Indianapolis, IN), according to the manufacturer’s instructions. The negative control was generated by Label Solution (Roche Diagnostics Corp, Indianapolis, IN) without terminal transferase. Positive control slides were generated by treating sections with recombinant DNase I. Each stained section was examined by fluorescent microscopy, and TUNEL-positive cells in the fetal brain were quantified as previously reported.
Neuron loss identification by Nissl staining
The fourth set of mirror slides was used to quantify neuronal density with the use of Nissl staining with Cresyl violet. Frozen slides were air dried for 1 hour, stained in Cresyl violet solution (0.1% Cresyl violet) for 5 minutes, rinsed in distilled water, differentiated in 95% ethyl alcohol for 15 minutes, dehydrated in 100% alcohol for 5 minutes twice, cleared in xylene for 5 minutes twice, and then mounted by resinous medium for the quantification.
Histologic injury marker quantification and reconstruction
Histologic quantification was performed as previously described. We selected 6 animals from each group (NMX, HPX, and L-NIL+HPX). Five slides were selected randomly from each animal, and 5 injured areas were selected randomly from each slide. The grading of ATF3, GFAP, and TUNEL staining intensity was determined in a blinded fashion with the use of a 1-4 scale: weak (+), positive stained cells in ≤25% of the surface area; medium (++), positive cells in >25%, but <50%, of the surface area; strong (+++), positive cells in ≥50%, but ≤ 70%, of the surface; very strong (++++), positive cells in ≥70% of the surface. For Nissl staining, the number of stained neurons was quantified with the Stereo Investigator software system (version 8.0; MBF Bioscience, Williston, VT). Neuronal density was defined as the mean total number of neurons per square millimeter.
The locations of the injured cells marked by ATF3, GFAP, and TUNEL were reconstructed from the immunostained/TUNEL-stained mirror sections with the aid of a microscope (Zeiss, Jena, Germany) and a fluorescent microscope (Nikon Instruments Inc, Melville, NY). The counts of positively stained cells were performed as described earlier; the locations of GFAP-, ATF3-, and TUNEL-positive cells were projected onto a schematic representation of the brain coronal section, as previous published.
The fifth and final set of mirror slides was used for LCM. The areas of injury identified by ATF3-, GFAP-, or TUNEL-labeling in the first 4 sets of mirror sections were excised selectively with LCM. The position of anatomic landmarks (third ventricle, hippocampus, and cortex) was projected visually onto the sections for LCM from hematoxylin-stained images. LCM was performed with the Veritas Microdissection Instrument (Arcturus Bioscience Inc, Mountain View, CA) on the unstained frozen section adjacent to the mirror section that had been processed for either immunostaining or TUNEL staining. The regions of the unstained section that corresponded to boundaries of the injured brain areas were dissected under a ×4 objective into CapSure Macro caps (Arcturus Bioscience Inc), and the LCM samples then were used for gene quantification. Laser settings varied as needed from 50-75 mW (power), 1500-3500 msec (duration), and 200-250 mV (intensity).
Quantitative reverse transcriptase polymerase chain reaction
Injury index genes (ATF3, GFAP, and Bax [a marker for apoptosis]) and the 3 NOS isoforms (nNOS, iNOS, and eNOS) messenger RNA (mRNA) expressions were quantified by SYBR Green I labeled (BioRad Laboratories, Hercules, CA) quantitative real-time polymerase chain reaction using mRNA extracted from LCM samples and whole fetal brain, as indicated. Total RNA was isolated (RNeasy Mini Kit and RNase-Free DNase Set; Qiagen, Valencia, CA) and reverse-transcribed (Sensiscript RT Kits; Qiagen). The primer sequence for each gene target was based on previous publications. Polymerase chain reaction parameters (iCyler iQ Real-time PCR Detection System; BioRad Laboratories) consisted of an initial denaturation at 95°C for 180 seconds, followed by 40 cycles at 95°C for 30 seconds, annealing at 60°C for 25 seconds, extension at 72°C for 30 seconds, and 1 cycle at 72°C for 7 minutes. A melt analysis was performed to confirm the specificity of the polymerase chain reaction amplification. Polymerase chain reaction efficiency was demonstrated by the standard curve slope. Target gene mRNA was quantified by the delta-delta computed tomography (2-DDCt) method and normalized to the 18S subunit of ribosomal RNA (Applied Biosystems, Foster City, CA).
Total NO product measurement
Nitrite/nitrate levels (NO 2 – and NO – ) were measured using a commercial assay (Fluorometric NO Assay; EMD Biosciences, San Diego, CA). Whole frozen fetal brains from NMX, HPX, and L-NIL+HPX animals were homogenized, centrifuged at 16,000 g for 20 minutes; the supernatant was filtered through a 10-kd cutoff filter at 14,000 g for 1 hour. Enzyme cofactors and nitrate reductase were added to each sample, based on the manufacturer’s instructions. The fluorescing reagent, 2,3-diaminonaphthotriazole, was then added to the samples and loaded onto 96-well plates that were read with a microtiter plate reader (Synergy HT Multi-Detection plate reader; Biotek US, Winooski, VT) at the following settings: excitation wavelength, 360-365 nm; emission wavelength, 430-450 nm.
Statistical analyses
All results are presented as the mean ± SEM. Comparisons between the control and study groups were calculated by t test or analysis of variance, as indicated. A probability value of < .05 was considered to indicate statistically significant differences between or among groups.
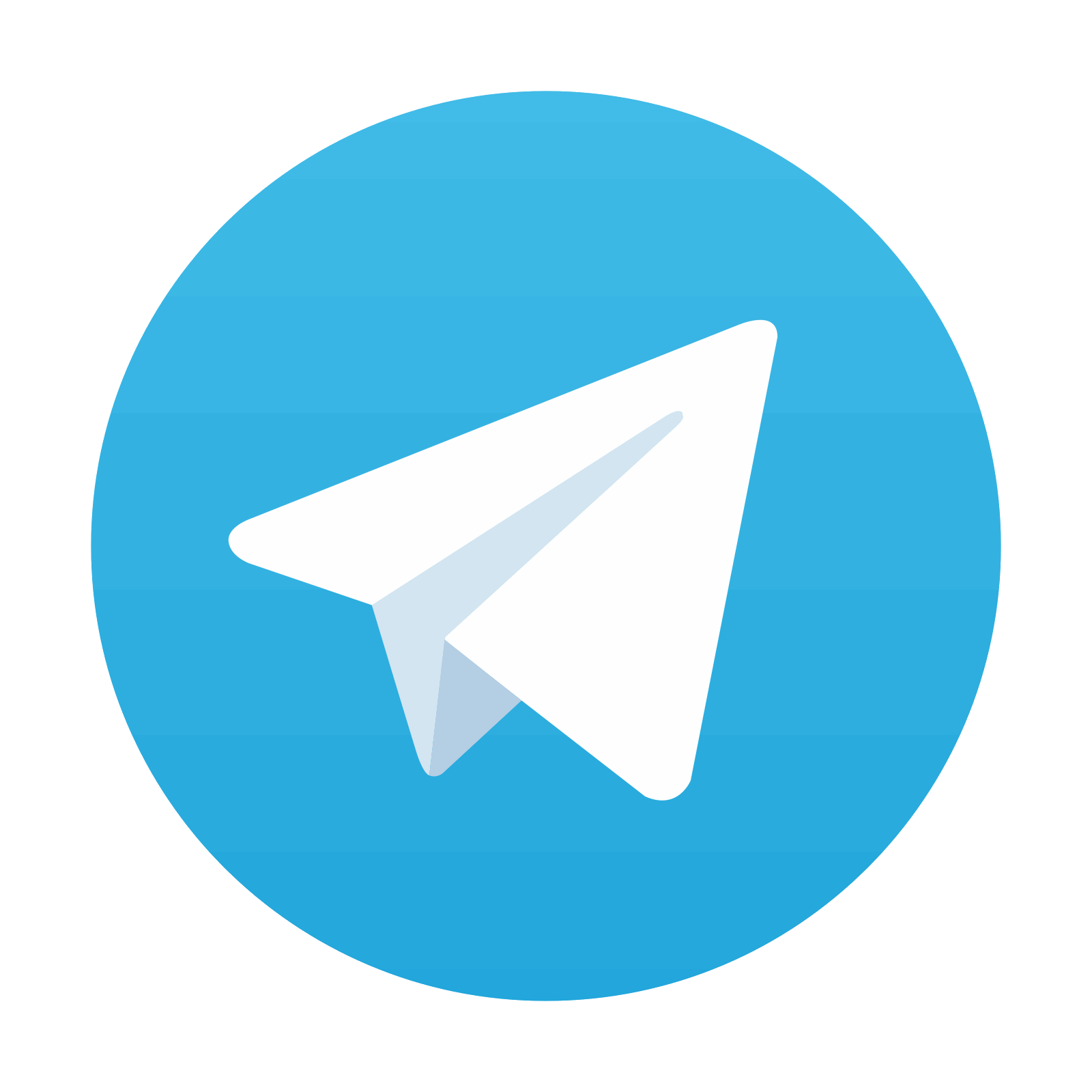
Stay updated, free articles. Join our Telegram channel

Full access? Get Clinical Tree
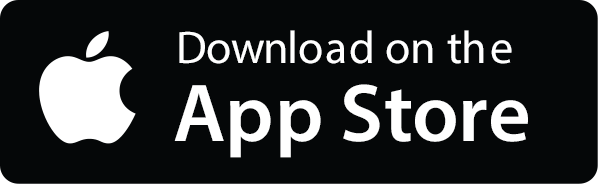
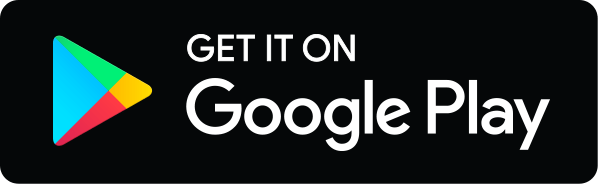