Objective
We sought to determine the predominant cell type (macrophage, T lymphocyte, B lymphocyte, mast cell) within the area of implantation of the prototypical polypropylene mesh, Gynemesh PS (Ethicon, Somerville, NJ); and to determine the phenotypic profile (M1 proinflammatory, M2 antiinflammatory) of the macrophage response to 3 different polypropylene meshes: Gynemesh PS (Ethicon), and 2 lower-weight, higher-porosity meshes, UltraPro (Ethicon) and Restorelle (Coloplast, Humblebaek, Denmark).
Study Design
Sacrocolpopexy was performed following hysterectomy in rhesus macaques. Sham-operated animals served as controls. At 12 weeks postsurgery, the vagina-mesh complex was excised and the host inflammatory response was evaluated. Hematoxylin and eosin was used to perform routine histomorphologic evaluation. Identification of leukocyte (CD45 + ) subsets was performed by immunolabeling for CD68 (macrophage), CD3 (T lymphocyte), CD20 (B lymphocyte), and CD117 (mast cell). M1 and M2 macrophage subsets were identified using immunolabeling (CD86 + and CD206 + , respectively), and further evaluation was performed using enzyme-linked immunosorbent assay for 2 M1 (tumor necrosis factor-alpha and interleukin [IL]-12) and 2 M2 (IL-4 and IL-10) cytokines.
Results
Histomorphologic evaluation showed a dense cellular response surrounding each mesh fiber. CD45 + leukocytes accounted for 21.4 ± 5.4% of total cells within the perimesh area captured in a ×20 field, with macrophages as the predominant leukocyte subset (10.5 ± 3.9% of total cells) followed by T lymphocytes (7.3 ± 1.7%), B lymphocytes (3.0 ± 1.2%), and mast cells (0.2 ± 0.2%). The response was observed to be more diffuse with increasing distance from the fiber surface. Few leukocytes of any type were observed in sham-operated animals. Immunolabeling revealed polarization of the macrophage response toward the M1 phenotype in all mesh groups. However, the ratio of M2:M1 macrophages was increased in the fiber area in UltraPro ( P = .033) and Restorelle ( P = .016) compared to Gynemesh PS. In addition, a shift toward increased expression of the antiinflammatory cytokine IL-10 was observed in Restorelle as compared to Gynemesh PS ( P = .011).
Conclusion
The host response to mesh consists predominantly of activated, proinflammatory M1 macrophages at 12 weeks postsurgery. However, this response is attenuated with implantation of lighter-weight, higher-porosity mesh. While additional work is required to establish causal relationships, these results suggest a link among the host inflammatory response, mesh textile properties, and clinical outcomes in the repair of pelvic organ prolapse.
More than 250,000 women per year in the United States will undergo surgery for the treatment of pelvic organ prolapse, with direct costs totaling >$1 billion. Native tissue repair has a recurrence rate of 40% at 2 years ; therefore, mechanical reinforcement of tissues using synthetic mesh has increased over the last decade. While mesh implantation has been shown to improve anatomical outcomes in the anterior and apical compartments, complications are observed, particularly with transvaginal placement, including mesh exposure through the vaginal wall, shrinkage, erosion, and pain.
Recent work suggests that mesh exposures may be induced by stress shielding. That is, a mismatch in stiffness between the mesh and tissue lead to degeneration of the underlying vagina and a loss of mechanical integrity over time. This maladaptive remodeling response precipitates atrophy of the smooth muscle layer associated with a decrease in contractility as well as a shift in tissue extracellular matrix composition and a loss of biomechanical integrity. Differences in mesh properties (weight, pore size, porosity, stiffness) were shown to be related to the degree to which this degenerative process occurs, with higher-weight, lower-porosity, and increased-stiffness mesh being associated with increased vaginal tissue degradation. Mesh with higher weight, lower porosity, and increased stiffness has also been suggested to result in increased rates of complications in clinical practice.
Mesh complications may also be attributable to the inflammatory processes associated with the macrophage-predominated foreign body reaction mounted by the host following implantation. Without question, the long-term presence of activated proinflammatory cells can have a negative impact on the ability of a material to function as intended. However, a number of recent studies have demonstrated that the macrophage response is also an essential component of the process leading to tissue incorporation, and functional remodeling of implanted materials, suggesting the potential for phenotypic dichotomy in the host response. Indeed, macrophages have been classified as having diverse and plastic phenotypes along a continuum between M1 (classically activated; proinflammatory) and M2 (alternatively activated; regulatory, homeostatic) extremes. An increasing number of studies in the field of biomaterials have begun to apply these paradigms and concepts, showing that macrophage polarization is a predictor of integration following implantation in multiple applications. However, the macrophage response following implantation of surgical mesh with varying characteristics has not been described. Moreover, limited studies to date have addressed the impact of mesh implantation on the vagina–an organ with an immunologically distinct environment from that of other tissues in which the host response to mesh has been examined.
The objectives of the present study were 2-fold: (1) to determine the predominant cell type (macrophage, T lymphocyte, B lymphocyte, mast cell) within the area of implantation of the prototypical polypropylene mesh, Gynemesh PS (Ethicon, Sommerville, NJ); and (2) to determine the phenotypic profile (M1 proinflammatory, M2 antiinflammatory) of the macrophage response to 3 different polypropylene meshes: Gynemesh PS, and 2 lower-weight, higher-porosity meshes, UltraPro (Ethicon) and Restorelle (Coloplast, Humblebaek, Denmark).
Materials and Methods
Meshes
The test articles consisted of 3 polypropylene meshes with varying textile and mechanical characteristics as previously described. Briefly, specific weight and pore size were provided by the manufacturer. Porosity was determined using a custom-designed algorithm (Matlab, Version 8.0; Mathworks, Natick, MA) and stiffness was determined by ball burst testing. Table 1 shows the relevant mechanical and structural characteristics associated with each mesh. Of note, UltraPro is manufactured with an absorbable component (poliglecaprolactone 25) in addition to polypropylene allowing it to have very large pores (4 mm) when this component is fully absorbed.
Gynemesh PS (Ethicon) | UltraPro (Ethicon) | Restorelle (Coloplast) | |
---|---|---|---|
Weight, g/m 2 | 44 | 31 | 19 |
Pore size, μm | 2240 | ≥4000 a | 2370 |
Porosity, % | 64 ± 2.1 | 69 ± 1.8 | 78 ± 3.0 |
Stiffness, N/mm | 28 ± 2.7 | 22 ± 2.8 | 11 ± 0.89 |
a UltraPro contained resorbable component (poliglecaprolactone 25) in addition to polypropylene allowing it to have very large pores (4 mm) when this component is resorbed; values reported with resorbable component dissolved.
Animals
The samples for the present study were obtained from a larger study. A subset of animals from that study was selected based on the availability of sufficient tissue samples for completion of the assays described in the present study. All animals in this study were maintained and treated according to an approved institutional animal care and use committee protocol and in accordance with the National Institutes of Health Guide for the Care and Use of Laboratory Animals. Demographic data of each animal were collected prior to surgery, including age, weight, gravidity, and parity ( Table 2 ). In all, 32 middle-aged parous rhesus macaques underwent implantation with Gynemesh PS (n = 8), UltraPro (n = 8), Restorelle (n = 8), or sham (n = 8). Mesh was implanted by sacrocolpopexy after an abdominal hysterectomy as previously described. Sacrocolpopexy was chosen as observational data suggest that complications related to this procedure are less than those following transvaginal implantation.
Groups | Age, y a | Parity b | Weight, kg a | POP-Q stage b |
---|---|---|---|---|
Sham | 12.6 ± 2.8 | 3 (2, 6) | 7.3 ± 1.4 c | 0 (0, 1) |
Gynemesh PS | 12.9 ± 2.2 | 4 (3.8, 5) | 8.2 ± 1.6 | 0 (0, 0) |
UltraPro | 13.0 ± 2.2 | 3.5 (2, 5.8) | 7.8 ± 1.4 | 0 (0, 0.25) |
Restorelle | 13.8 ± 1.7 | 5 (3, 5.5) | 10.0 ± 2.8 c | 0.5 (0, 1.3) |
P value d | .780 | .970 | .042 | .700 |
b Median (first quartile, second quartile)
c Statistical significance between groups ( P < .05)
Sample harvest
At 12 weeks postsurgery, vagina-mesh tissue complexes were harvested as previously described. The equivalent tissues were excised in sham-operated animals. A portion of the vagina-mesh complex was embedded in optimal cutting temperature solution (Sakura Finetek USA, Torrence, CA) prior to flash freezing on liquid nitrogen for histologic staining and immunofluorescent labeling. Another portion was harvested and frozen for enzyme-linked immunosorbent assay (ELISA). All samples were stored at –80°C until testing.
Histologic staining and immunofluorescent labeling
Tissue sections (7 μm) were cut and stored at –80°C until use. Slides were thawed at room temperature and stained with hematoxylin and eosin. Slides were dehydrated through a series of graded ethanol (70-100%) and xylenes prior to coverslipping. The histologic appearance of the tissue sections was then evaluated and imaged using a microscope (E600; Nikon, Melville, NY).
For immunolabeling, sections were fixed in 50:50 methanol/acetone for 10 minutes. Antigen retrieval was performed in 10 mmol/L citric acid monohydrate buffer (pH 6.0) at 95°C for 20 minutes. After cooling, the sections were incubated in copper sulfate with ammonium acetate for 20 minutes at 37°C to reduce autofluorescence. The sections were blocked with 1% normal donkey serum, 2% bovine serum albumin, 0.1% Triton-X100, and 0.1% Tween 20 at room temperature for 1 hour. Consecutive sections were then labeled with antibodies specific for leukocytes (CD45), macrophages (CD68), T lymphocytes (CD3), B lymphocytes (CD20), and mast cells (CD117). Primary antibodies, diluted in blocking solution, were applied overnight at 4°C, followed by the corresponding secondary antibodies (product information and dilutions for each primary and secondary antibody are listed in Supplemental Table ) and then coverslipped using aqueous mounting media containing 4′,6-diamidino-2-phenylindole; (DAPI; Vectashield with DAPI; Vector Laboratories, Burlingame, CA). Localization of staining to the appropriate regions of lung, liver, kidney, spleen, lymph node, and intestine were used to verify appropriate labeling and incubation of slides without primary antibodies was used as a control. Three representative areas of the mesh-tissue interface were imaged for each individual marker using a ×20 objective on an imaging microscope (Eclipse 90i; Nikon). Quantification of cell types was performed using software (ImageJ; National Institutes of Health, Bethesda, MD). Cell counts were averaged for each sample and expressed as a percentage of total cells within a ×20 field.
Additional sections were triple-labeled with antibodies specific for a panmacrophage marker (CD68), an M1 marker (CD86), and an M2 marker (CD206) as above. Slides were imaged using a ×20 objective at the interface with either single fibers (3 images) or mesh knots (3 images) using a standardized protocol. CD68 + CD86 + cells were considered to have an M1 phenotype and CD68 + CD206 + cells, an M2 phenotype. Cell counts were averaged for each sample and expressed as a percentage of total cells within a ×20 field. Additionally, the ratio of M2:M1 cells was calculated. Because of the scarcity of macrophages in the sham group, the ratio of M2:M1 was not reported. The perimeter of the mesh-tissue interface present in each image was calculated by tracing using ImageJ.
ELISA assay
Frozen tissues were mechanically pulverized and homogenized in a high salt buffer (50 mmol/L Tris base, 150 mmol/L sodium chloride, and 10 μg/mL Halt protease inhibitor cocktail, Pierce Biotechnology, Rockford, IL). After centrifugation, supernatants were collected. Using the DC protein assay (Bio-Rad, Hercules, CA), protein concentrations of all extracts were determined so that all sample volumes contained 40 μg of protein. Amounts of proinflammatory M1 (tumor necrosis factor-alpha, and interleukin [IL]-12p70) and antiinflammatory M2 (IL-10, IL-4) cytokines were assessed using commercially available ELISA assays (Life Technologies, Carlsbad, CA). Both the concentrations of individual cytokines and the ratio of M2/M1 cytokines ([IL-10 + IL-4]/[tumor necrosis factor-alpha + IL-12]) were calculated.
Statistical analysis
Statistical comparisons were made using software (SPSS 18.0; SPSS Inc, Chicago, IL). Primate demographic and immunolabeling data were assessed using 1-way analysis of variance with a Tukey post hoc procedure. As cytokine data were nonparametric, a Kruskal–Wallis test with a Bonferroni-adjusted alpha after pairwise comparisons was performed for each group. A Spearman correlation was used to examine the relationship between the number of M1 and M2 cells and mesh perimeter in each image. A P value < .05 was used to determine significance.
Results
Animals had similar age, parity, and pelvic organ prolapse quantification (POP-Q) stage ( Table 2 ). The POP-Q staging methods utilized were the same as that utilized in human beings adjusted to account for the shorter length of the macaque vagina. Animals in the Restorelle group weighed more than the other groups ( P = .042); however, weight did not correlate with any of the measured outcomes ( P > .36 for all). One animal in the study demonstrated a mesh exposure into the vagina. There were no erosions into adjacent structures.
Histologic analysis
All samples had an intact and qualitatively normal vaginal epithelium as well as a clearly delineated subepithelium, muscular layer, and adventitia ( Figure 1 ). The subepithelial tissues were histologically similar across all groups, with few differences observed between sham and mesh-implanted animals. The largest differences between samples occurred in the smooth muscle layer as previously described, in which the Gynemesh PS induced the most negative impact. All mesh-implanted animals elicited an inflammatory reaction to individual mesh fibers and around knots consisting of a dense infiltrate of mononuclear cells and formation of a fibrous capsule. The response was highly localized with fewer cells observed with increasing distance from the mesh. Multinucleated giant cells were observed at the surface of some, but not all, fibers and knots, regardless of mesh type. The cells at the mesh-tissue interface were predominantly mononuclear in appearance and few, if any, polymorphonuclear cells (neutrophils) were observed. The adventitial layer in sham-operated animals was qualitatively normal, consisting of well-organized loose connective tissue.

Characterization of the immune response to polypropylene mesh
CD45 + cells (panleukocyte) were observed predominantly at the mesh-tissue interface and in the perimesh space in the adventitia with few, if any of these cells within the subepithelium or muscularis of Gynemesh PS–implanted animals indicating a highly localized inflammatory response. CD68 + cells (macrophage) were the immune cell type found in the greatest density immediately surrounding each mesh fiber, while other cells types were fewer in number and found to be located more distantly from the mesh surface ( Figure 2 ). CD45 + cells accounted for 21.4 ± 5.4% of total cells within ×20 fields at the mesh-tissue interface. CD68 + cells (macrophages, 10.5 ± 3.9%) were found to be the predominant leukocyte subtype, followed by CD3 + (T lymphocyte, 7.3 ± 1.7%), CD20 + (B lymphocyte, 3.0 ± 1.2%), and CD117 + (mast, 0.2 ± 0.2%) cells. Although the percentage of macrophages was 44% greater than that of T cells, no significant statistical differences were observed between these two. Both the percentage of macrophages and T lymphocytes were significantly higher than the percentage of B lymphocytes or mast cells (all P < .001) ( Table 3 ). No differences in the total number of DAPI + cells were observed between image sets for each antibody. Few positively labeled cells of any type were observed within the sham (<5 per ×20 field) and, therefore, quantitative analysis of immunolabeled slides was not performed for this group.

Treatment (Gynemesh PS) | Total no. of cells (per ×20 field) | Positive cells (per ×20 field), % |
---|---|---|
CD45 | 514 ± 121 | 21.4 ± 5.4 |
CD68 | 510 ± 108 | 10.5 ± 3.9 a |
CD3 | 510 ± 114 | 7.3 ± 1.7 b |
CD20 | 509 ± 109 | 3.0 ± 1.2 |
CD117 | 508 ± 121 | 0.2 ± 0.2 |
P value | 1.00 c | <.001 d |
a Significance seen between CD68 and CD20, and CD68 and CD117
b Significance seen between CD3 and CD20, and CD3 and CD117
c Comparison of P value among groups, significant difference if P < .05
Analysis of macrophage phenotype
In all implanted animals, the macrophage response to mesh was observed to be predominantly of the M1 phenotype with fewer cells of either phenotype observed with increasing distance from the mesh surface ( Figure 3 ). In areas with individual fibers, the percentage of M1 cells per ×20 field was increased in mesh-implanted groups (Gynemesh PS 6.7 ± 2.8%, P = .003; UltraPro 7.3 ± 2.6%, P = .002; Restorelle 7.0 ± 3.7%, P = .008) relative to sham (0.8 ± 0.7%). The percentage of M2 cells was also increased in mesh-implanted groups (Gynemesh PS 3.5 ± 2.2%, P = .046; UltraPro 4.6 ± 1.5%, P = .001; Restorelle 4.6 ± 2.1%, P = .001) relative to sham (0.07 ± 0.03%). The percentage of M2 cells around individual fibers was similar in lighter-weight, higher-porosity meshes (UltraPro, P = .24; Restorelle, P = .32) as compared to Gynemesh PS. However, the M2/M1 ratio around individual fibers was higher for UltraPro ( P = .033) and Restorelle ( P = .016) as compared to Gynemesh PS ( Table 4 ). However, the M2/M1 ratio around individual fibers was higher for UltraPro ( P = .033) and Restorelle ( P = .016) as compared to Gynemesh PS ( Table 4 ).

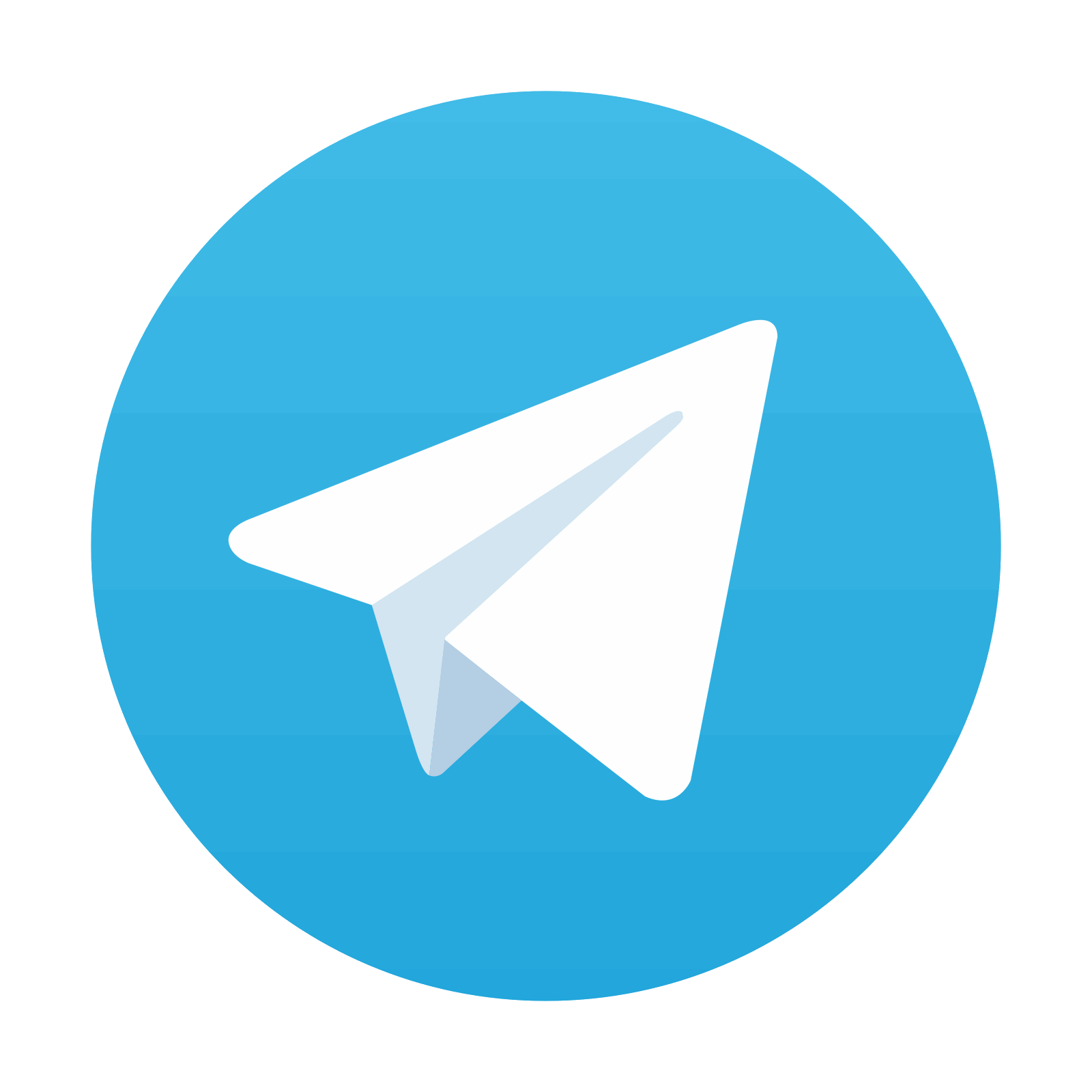
Stay updated, free articles. Join our Telegram channel

Full access? Get Clinical Tree
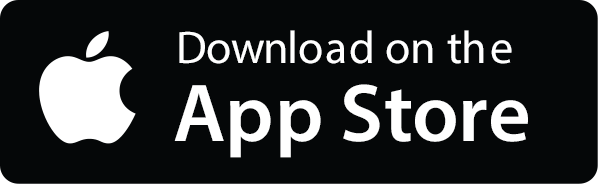
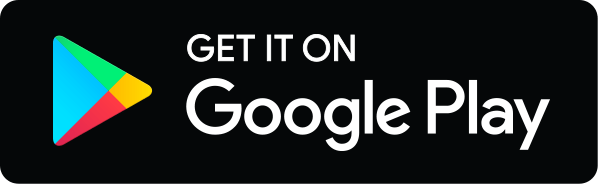
