Abstract
The main aim of preimplantation genetic testing (PGT), which up until recently was known as preimplantation genetic diagnosis (PGD), is the identification of embryos that are free of inherited genetic conditions. PGT can therefore be considered as a treatment option for couples where one or both partners are at risk of transmitting such a condition to their offspring. Inherited genetic conditions can affect gene function or chromosome structure, and could either be present in families or arise de novo. PGT for inherited mutations affecting gene function is defined as PGT for monogenic disorders or PGT-M, whereas PGT for inherited chromosome rearrangements is termed as PGT for structural rearrangements or PGT-SR (). The selection and preferential transfer of healthy embryos could lead to the birth of babies who are free of the genetic disorder for which PGT was carried out, as well as potentially eradicate it from the family. Hence, PGT can be considered as an alternative form of prenatal diagnosis, with the added advantage that it avoids the termination of affected pregnancies.
7.1 Introduction
The main aim of preimplantation genetic testing (PGT), which up until recently was known as preimplantation genetic diagnosis (PGD), is the identification of embryos that are free of inherited genetic conditions. PGT can therefore be considered as a treatment option for couples where one or both partners are at risk of transmitting such a condition to their offspring. Inherited genetic conditions can affect gene function or chromosome structure, and could either be present in families or arise de novo. PGT for inherited mutations affecting gene function is defined as PGT for monogenic disorders or PGT-M, whereas PGT for inherited chromosome rearrangements is termed as PGT for structural rearrangements or PGT-SR (1). The selection and preferential transfer of healthy embryos could lead to the birth of babies who are free of the genetic disorder for which PGT was carried out, as well as potentially eradicate it from the family. Hence, PGT can be considered as an alternative form of prenatal diagnosis, with the added advantage that it avoids the termination of affected pregnancies.
The first PGT case took place over 20 years ago and involved the sexing of embryos with the use of the polymerase chain reaction (PCR) (2). During a PGT treatment cycle embryos are generated via in vitro fertilization (IVF), and sampled by employing a procedure called embryo biopsy. During biopsy one or more cells are removed from the embryo for subsequent genetic testing. Biopsy can take place at different stages of embryo development, as will be outlined further on. Embryo testing can occur with several different diagnostic strategies, depending on the type of condition for which PGT is being carried out.
A modified version of PGT termed PGT-A where the A stands for aneuploidy, aims to identify numerical chromosome abnormalities present in embryos. Soon after the development of methods capable of examining the chromosomes of embryos, in the context of PGT, it became evident that the presence of numerical abnormalities is extremely common during preimplantation development (e.g., 3, 4). The transfer of aneuploid embryos during IVF is unlikely to lead to ongoing pregnancies and live births. It has therefore been hypothesized that the preferential selection and transfer of chromosomally normal embryos would improve clinical outcomes for couples requiring IVF in order to conceive (5). Currently PGT-A is mostly used for couples going through IVF who are also considered to be at elevated risk of generating gametes and/or embryos affected by aneuploidy. Patient groups that would seek to use PGT-A to aid them achieve a pregnancy commonly include those who have experienced repeated implantation failure (RIF), or recurrent pregnancy loss (RPL), or where the female partner is of advanced reproductive age/advanced maternal age (ARA/AMA). PGT-A could also be used for male factor infertility cases where there is an indication of increased sperm aneuploidy, as well as for couples who have had previous aneuploid conceptions (1, 5, 6). The clinical procedures associated with PGT-A are very similar to those taking place during PGT, and will be outlined further on. A detailed overview of PGT-M, PGT-SR, and PGT-A will be undertaken in this chapter. Additionally, the clinical value, potential benefits, and drawbacks of genetic testing in the context of PGT-M, PGT-SR, and PGT-A will be discussed.
7.2 Embryo Sampling and Genetic Analysis
There are three different stages of early development during which embryo biopsy can take place, namely, before and after fertilization, on day 3 when the embryo is at the cleavage stage, or on days 5 or 6 when the embryo has undergone the first cellular differentiation into trophectoderm (TE, will give rise to the placenta), and inner cell mass (ICM, will give rise to the fetus) and has become a blastocyst (reviewed in 7). Biopsy taking place before and after fertilization involves the removal of the first and second polar bodies (PBs), respectively, cleavage-stage biopsy involves the removal of a single blastomere, whereas blastocyst-stage biopsy involves the removal of a few (usually three to five) TE cells. PB sampling can only be used for female carriers of genetic conditions as the analysis of these cells provides information on the status of the oocyte. Cleavage and blastocyst-stage sampling and analysis, on the other hand, provide information on the status of the embryo. Currently, blastocyst-stage biopsy is the most widely used and it is considered the least damaging to the embryo, as it samples cells from the TE that will form the placental tissue after implantation. PB biopsy is only employed in countries where embryo sampling is not allowed. Technical details for the three different biopsy stages, as well as their advantages and disadvantages, are summarized in Table 7.1.
Table 7.1 Biopsy stages for preimplantation genetic testing
Biopsy type | When does it take place? | How does it take place? | Cell type removed | Type of errors assessed | Used for PGT-M, PGT-SR, and PGT-A? | Impact on embryo development? | Other considerations |
---|---|---|---|---|---|---|---|
First and second PBs |
|
| Polar bodies which are by-products of oocyte meiosis I and II | Gene mutations or chromosome abnormalities of female origin | Yes | No |
|
Cleavage stage | Day 3 of preimplantation development |
| Blastomere |
| Yes | Yes, cleavage-stage biopsy has been shown to reduce embryo implantation ability |
|
Blastocyst stage | Days 5 or 6 of preimplantation development |
| Cells from trophectoderm part of the embryo which will form the placenta after implantation |
| Yes | Little if any |
|
A single cell contains 5–10 pg of DNA. The analysis of such minute DNA amounts during PGT, means that all diagnostic strategies will include an initial DNA amplification step, and can end up being technically demanding. Issues such as DNA contamination, amplification failure, or allele dropout (ADO) can all influence the diagnostic accuracy of PGT, especially when this takes place for the detection of mutations affecting gene function (PGT-M) (8). ADO is defined as the amplification failure of one of the alleles in a heterozygous cell. This amplification failure would cause the heterozygous cell to appear homozygous at the ADO affected locus, increasing in this way the misdiagnosis risk (4). To avoid such issues and maximize diagnostic accuracy, standard PGT-M approaches commonly use multiplex PCR methodologies, during which several different loci, including those affected by the mutation as well as others in the vicinity, are simultaneously amplified with the use of several distinct primer sets (9). The development of robust diagnostic PGT-M strategies requires careful protocol design optimization and validation, and can be lengthy and expensive. It is therefore not unusual for patients requesting PGT-M to have to wait for several months before starting their IVF cycle so as to undergo embryo testing. Moreover, PGT-M via multiplex PCR does not permit chromosome assessment, meaning that any aneuploid embryos will not be identified.
Recently an alternative PGT-M approach has been developed, termed karyomapping (10). Karyomapping employs an array platform which consists of approximately 300,000 single-nucleotide polymorphisms (SNPs). These SNPs are spread across the human genome, and during PGT-M are used to perform linkage analysis for the gene of interest and determine the presence of normal or mutated copies in the examined embryos (9, 10). Karyomapping enables the application of a single protocol for the PGT-M of several different inherited monogenic conditions, and importantly, allows the detection of certain types of chromosome abnormalities, such as losses leading to monosomies as well as some gains leading to trisomies. Additionally, the parental origin of meiotic chromosome errors can be determined.
Targeted next-generation sequencing (NGS) approaches have also been developed for PGT-M (11). NGS is a term that encompasses a variety of different methods capable of generating large quantities of DNA sequence information, rapidly, and at a low cost per base. Targeted NGS strategies have several advantages, over karyomapping, including the combination of accurate mutation detection, as well as all different aneuploidy types, and a potential decrease in costs associated with the procedure. A summary of the technical aspects, advantages, and limitations of the main PGT-M strategies can be found in Table 7.2.
Methodology | Technical details | Work-up required? | Aneuploidy detection? | Other considerations |
---|---|---|---|---|
Multiplex PCR |
| Yes | No, unless chromosome carrying the mutation is affected |
|
Karyomapping |
| Yes, in cases where specific mutation detection is required | Yes |
|
Targeted next-generation sequencing |
| Yes, to ensure that all primers combine without technical issues | Yes |
|
For many years the main method for the PGT of chromosome abnormalities, both structural (PGT-SR) and numerical (PGT-A) was fluorescent in situ hybridization (FISH), with thousands of successful cycles having been carried out with its use. However, FISH-based PGT tests had several limitations. The most important of these limitations was the inability of FISH to assess the entire chromosome complement of biopsied samples. FISH PGT-SR or PGT-A strategies would only examine a limited number of chromosomes. Specifically, PGT-SR FISH protocols would focus on the chromosomes participating in the rearrangement, whereas those for PGT-A would target chromosomes that were most frequently seen in samples from spontaneous miscarriages and abnormal live births, and have been known to increase in malsegregation frequency with advancing female age, such as 13, 14, 15, 16, 18, 21, 22, and the sex chromosomes. Similar to PGT-M, most PGT-SR protocols had to be tailor-made for the structural rearrangement in question, and their optimization and validation could end up being technically challenging and lengthy. Moreover, FISH needed embryonic nuclei to be fixed on microscope slides, a process which required specialized skills and experience, and could lead to diagnostic problems, such as unclear or overlapping chromosome-specific probe signals.
The FISH associated shortcomings led to the development and use of new molecular comprehensive cytogenetic methodologies, which were capable of examining the entire chromosome complement of biopsied embryonic cells. The first molecular cytogenetic method to be used for the analysis of embryonic samples was metaphase comparative genomic hybridization (CGH) (e.g., 4). CGH involves a competitive hybridization of two differentially labeled DNAs, one which is normal (reference sample) and another one of unknown constitution (test sample) on a microscope metaphase slide, followed by analysis which will determine the karyotype of the test sample. Metaphase CGH evolved to microarray CGH (aCGH), a similar method which used microarray slides for the competitive hybridization to take place, rather than the metaphase ones (12). Other PGT-A approaches used SNP microarrays or fluorescent quantitative real-time PCR (13), whereas various different NGS strategies have been devised recently (14). NGS has several advantages, compared to other comprehensive chromosome screening approaches, and for this reason it has become the method of choice for both PGT-SR and PGT-A in recent years. Table 7.3 summarizes the technical details and compares each of the main PGT-A and PGT-SR diagnostic strategies.
Table 7.3 PGT-SR and PGT-A diagnostic strategies
Methodology | Technical details | Work-up required? | Aneuploidy types detected | Other considerations |
---|---|---|---|---|
FISH |
| Yes, for PGT-SR | Whole or segmental abnormalities for targeted chromosomes |
|
CGH |
| No | Whole or segmental abnormalities for entire chromosome complement |
|
aCGH |
| No | Whole or segmental abnormalities for entire chromosome complement |
|
SNP arrays |
| No | Whole or segmental abnormalities for entire chromosome complement |
|
Q-PCR |
| No | Whole abnormalities for entire chromosome complement |
|
NGS |
| No | Whole or segmental abnormalities for entire chromosome complement |
|
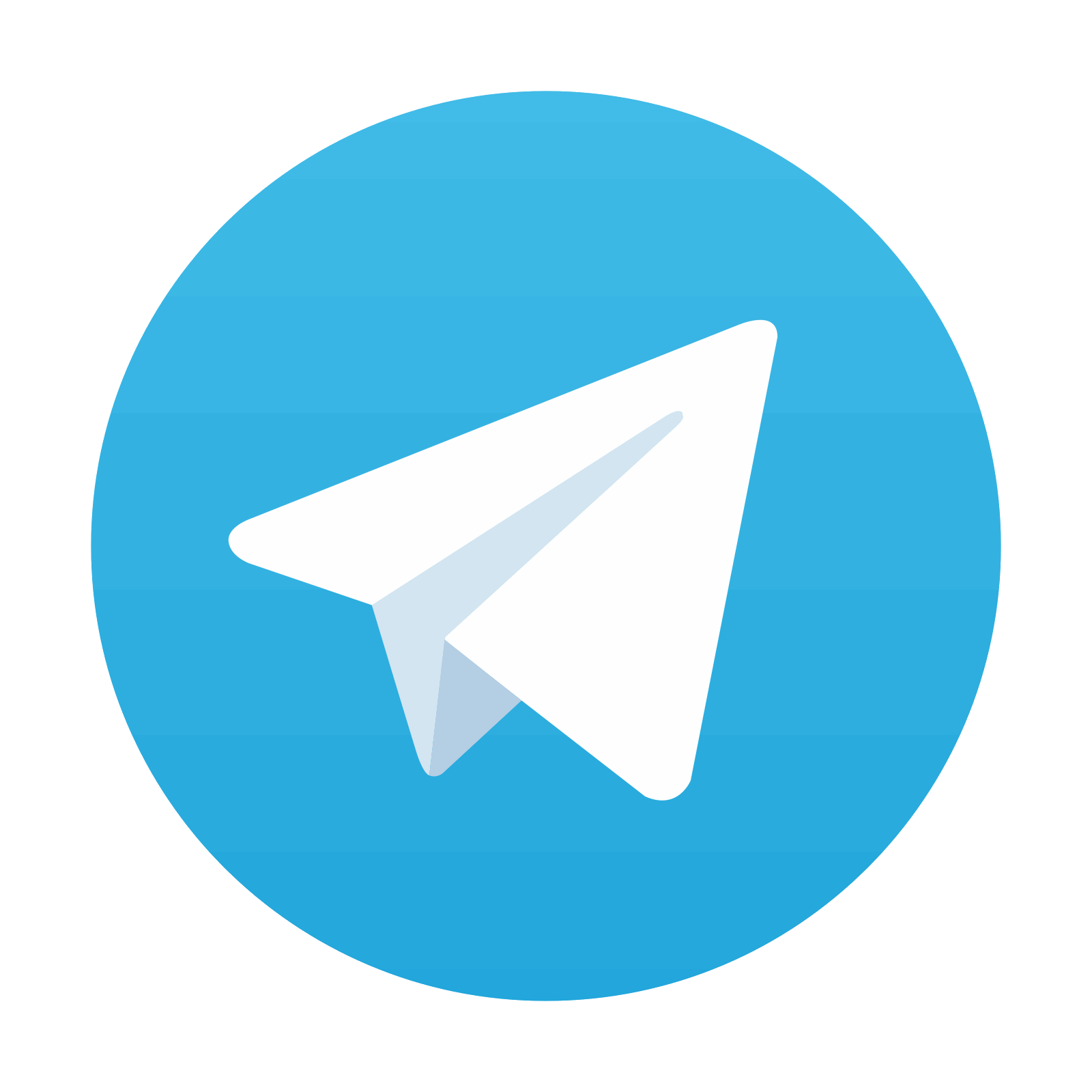
Stay updated, free articles. Join our Telegram channel

Full access? Get Clinical Tree
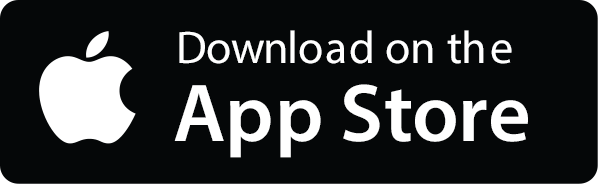
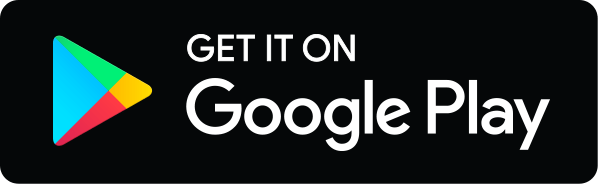
