Abstract
The history of in vitro fertilization (IVF) and embryo transfer (ET) goes back to the early 1890s when Walter Heape, a professor and physician at the University of Cambridge, England, reported the first known case of embryo transplantation in rabbits, long before the applications to human fertility were even suggested. In 1934 Pincus and Enzmann, from the Laboratory of General Physiology at Harvard University, published a paper in the Proceedings of the National Academy of Sciences of the USA, raising the possibility that mammalian oocytes can undergo normal development in vitro. Two decades later, in 1948, Miriam Menken and John Rock retrieved more than 800 oocytes from women during operations for various conditions. One hundred thirty-eight of these oocytes were exposed to spermatozoa in vitro, and they published their experiences in the American Journal of Obstetrics and Gynecology.
6.1 History of IVF and ICSI
The history of in vitro fertilization (IVF) and embryo transfer (ET) goes back to the early 1890s when Walter Heape, a professor and physician at the University of Cambridge, England, reported the first known case of embryo transplantation in rabbits, long before the applications to human fertility were even suggested. In 1934 Pincus and Enzmann, from the Laboratory of General Physiology at Harvard University, published a paper in the Proceedings of the National Academy of Sciences of the USA, raising the possibility that mammalian oocytes can undergo normal development in vitro. Two decades later, in 1948, Miriam Menken and John Rock retrieved more than 800 oocytes from women during operations for various conditions. One hundred thirty-eight of these oocytes were exposed to spermatozoa in vitro, and they published their experiences in the American Journal of Obstetrics and Gynecology.
The first mammalian (rabbit) life birth after IVF was reported by Chang and colleagues in 1959. Fertilization was induced by the incubation of ovulated oocytes with capacitated sperm in a small Carrel flask for four hours. This achievement paved the way for human reproductive technologies. It took more than two decades before the first human IVF pregnancy was reported by the Monash research team of Professors Carl Wood and John Leeton in Melbourne, Australia. Unfortunately, this resulted in an early miscarriage.
Professionals in the fields of microscopy, embryology, and anatomy laid the foundations for future achievements. Through the years, numerous modifications have been made in the development of human IVF: refinement of fertilization and embryo culture media, improvements in equipment and cleanroom conditions, embryo biopsy, and genetic testing. The world’s first B2 culture medium, known as “the French medium,” was developed by Menezo in 1976. This specific medium reflected the follicular, tubal, and uterine environments of the sheep, rabbits, and humans.
Prior to 1978, women with defected fallopian tubes were considered to be sterile by their physicians. At least one functional fallopian tube is necessary for natural fertilization of an oocyte by sperm in vivo. Physicians often performed reparative surgeries for the defective tubes as an endeavor of restoring fertility. IVF was considered experimental, was not applied clinically and resulted in miscarriages whenever attempted. Not until the late 1970s when a patient of nine years of primary infertility due to tubal occlusion named Lesley Brown, was treated by Patrick Steptoe and Robert Edwards. She underwent laparoscopic single oocyte retrieval, that was fertilized in the laboratory and later transferred on day 2.5 as an eight-cell embryo into the uterus and resulted in the first live birth from IVF on July 25, 1978, Louise Brown (1).
In the mid-1980s new techniques were developed to increase the success rate after embryo transfer. One of these techniques was referred to as gamete intrafallopian transfer (GIFT) in which oocytes are retrieved laparoscopically, followed by immediate replacement of the oocytes and sperm in the fallopian tubes. Despite the fact that GIFT increased the success rates, patients with severe male factor, tubal occlusion, or pelvic adhesions could not benefit from this technique. Another technique arose in that period called zygote intrafallopian transfer (ZIFT) where oocytes were retrieved laparoscopically, fertilized in vitro and transferred into the fallopian tubes at the pronuclear stage. While ZIFT allowed the confirmation of fertilization, the use of two laparoscopies, one for oocyte retrieval and the other for zygote transfer, was a major limitation of this approach (2).
In 1987 transvaginal follicle aspiration was introduced, this method of follicle aspiration became the procedure of choice due to better visualization, precise control, and less patient discomfort (3). Infertility treatment regimens became more dependent on conventional IVF, however, there was still a limitation in the treatment of male factor infertility, whether it was due to a lower sperm count (oligozoospermia), sperm motility (asthenozoospermia), morphology (teratozoospermia), or even azoospermic males. Several procedures were developed in the eighties in order to improve the success rate and to compensate the compromised outcome in cases of male factor infertility. The first procedure was partial zona dissection (PZD) where a small opening was made in the zona pellucida to enhance sperm penetration (4). Another technique was subzonal insemination (SUZI) where few motile sperm were microinjected in the perivitelline space. The results of these techniques were not satisfactory, were inconsistent, and were of limited use in cases of severe male infertility. The work of Gianpiero Palermo was dedicated to improving fertilization especially in cases of severe male infertility. Palermo exposed sperm cells to follicular fluid to enhance acrosome reaction (5) and reported a fertilization rate of 40 percent using SUZI when adequate number of sperm cells were available but failed to achieve the same outcome in cases of severe male factor cases. To overcome the low fertilization in severe male factor cases, Palermo decided to further enhance the interaction between the sperm cell and the oocyte by releasing the sperm in a cleft in the oolema. While performing this procedure, in some cases unintentionally the membrane was pierced, and the spermatozoon was released in the ooplasm. He noticed that almost whenever this incident happens the oocyte got fertilized. Palermo shared his observation with Hubert Joris, a technician who was working with him, who recalled a case where the only fertilized oocyte was the one pierced accidently through the membrane, and the resulting embryo was transferred and achieved a pregnancy.
The technique of intracytoplasmic sperm injection (ICSI) where a single sperm is injected directly into the oocyte’s cytoplasm was one of the biggest achievements in the field of reproductive medicine, introduced in 1992 by Palermo and Van Steirteghem (6). Fertilization rates of 60–70 percent were reported using ICSI, which was a significant improvement compared to other techniques such as SUZI and PZD. From that day onward, ICSI could successfully be applied in cases of severe male factor and surgically retrieved sperm.
6.2 Natural Conception
Fertilization is a complex process that leads to the union of the male and female germ cells to produce a zygote. Fertilization only occurs after both the sperm cell and the oocyte complete their cytoplasmic and nuclear maturation. In nature, normal fertilization involves several essential consecutive steps:
ovulation
introduction of adequate number of motile sperm within the vagina
sperm capacitation
sperm interaction with the cumulus cells
sperm penetration of the zona pellucida
sperm–oocyte fusion
oocyte activation
pronucleus formation
syngamy
Pituitary gonadotropins act on large antral follicles and initiate the resumption of meiosis for oocytes arrested at prophase of meiosis I. The cumulus oophorus starts to expand in preparation for ovulation, these sticky cells will aid in the oocyte-sperm interaction as well as the uptake by the human oviduct fimbriae. Semen is disposed near the cervical os and sperm will start swimming into the cervical canal, which will coagulate within minutes of coitus by forming a loose gel structure made of structural proteins (Semenogelin I and II). Prostate-specific antigen, which is an enzyme secreted by the prostate gland, will degrade the gel coagulum after 30–60 minutes. The coagulum protects the sperm from vaginal acidic environment and immunological responses that can damage the sperm. The sperm traverse the cervical canal and will exit the uterine cavity into the isthmus of the oviduct. Out of the millions of sperm disposed in the anterior vagina, only a few thousand reach the oviducts, and only hundreds make it to the site of fertilization, the ampulla region, which can be thought of as a natural selection of the fittest and most suitable sperm to achieve fertilization.
Sperm capacitation is a combination of processes that alter the sperm cell structure to enable them to undergo the acrosome reaction and result in obtaining fertilization competence. These processes involve alteration of the membrane proteins and lipids to get rid of the inhibitory factors, changes in the intracellular pH, calcium concentration, and cAMP-dependent protein tyrosine phosphorylation and dephosphorylation. Human sperm capacitation starts as soon as the sperm is ejaculated and takes around six hours. In vitro, capacitation can be induced by the removal of the seminal plasma during a swim-up procedure or gradient and incubating the spermatozoa in a bicarbonate-buffered solution containing electrolytes, metabolic energy sources, and protein source, at 37°–39°C. The acrosome represents the anterior region of the sperm head, and contains several enzymes including hyaluronidase, acrosin, collagenase, phospholipase C, and arylsulfatase. The spermatozoa traverse the cumulus oophorus by utilizing its motility and with the help of enzyme hyaluronidase that is either present on the sperm plasma membrane or from the sperm acrosome. The sperm then reaches the zona pellucida (ZP) which triggers the acrosome reaction process, causing changes in the sperm plasma membrane permeability and intracellular ions concentration. The oocyte ZP is an acellular glycoprotein that is composed of four glycoproteins: ZP1, ZP2, ZP3, and ZP4 that are encoded by genes on chromosomes 11, 16, 7, and 1, respectively. The capacitated sperm bind to both ZP3 and ZP4 inducing the acrosome reaction marked by the fusion of the outer acrosomal membrane and the sperm head plasma membrane allowing its content to be released. The acrosome-reacted sperm then binds to ZP2 and releases enzymes such as acrosin and lysins that dissolve the ZP.
Once the sperm is through the ZP, it binds to the oolemma and induces the cortical reaction. Cortical granules are membrane-bound organelles containing enzymes and mucopolysaccharides. When the sperm penetrates the ZP, the cortical granules fuse with the oocyte plasma membrane and release their content into the perivitelline space by exocytosis, causing hardening of the ZP, and the oocyte plasma membrane becomes a mosaic of cortical granule membrane and original plasma membrane. The cortical reaction process also induces the hydrolysis of ZP sperm receptors which is essential in the block to polyspermy.
Oocyte activation is marked by the cortical granule exocytosis and resumption of meiosis. After the sperm penetrates the oolemma the sperm postacrosomal region fuses with the oocytes’ microvillus surface. The sperm introduces soluble cytosolic factor, phospholipase C zeta, into the oocyte cytoplasm which triggers the oocyte activation via inositol 1,4,5-triphosphate pathway (IP3), which triggers the calcium oscillations. The sperm nucleus contains chromatin that is tightly packed with highly charged amino acids called protamines, which are responsible for condensing the DNA and repressing transcription. Extensive disulfide bonds between protamines make the sperm head rigid which is important for zona pellucida penetration. When the sperm nucleus enters the oocyte cytoplasm, the disulfide bonds between the protamines are reduced by oocyte-derived glutathione. Another molecule is required to replace the protamines with oocyte histones, data suggest that this molecule is heparin sulfate. The sperm nuclear envelope breaks down and the highly condensed chromatin disperse so that chromatin filaments are released into the cytoplasm.
The sperm enters the oocyte when it is in metaphase II stage, during the sperm chromatin decondensation, as described above, the oocyte enters the telophase II. During telophase II sperm chromatin decondensation is completed, protamines replaced by histones and the male and female pronuclear envelops develop synchronously. During oogenesis the oocyte loses both centrioles and does not exhibit granular perinuclear centrosomal material, while during spermatogenesis there is partial loss of male centrosomes when the spermatids transform into mature sperm. The proximal centriole remains intact, though inactive, while the distal centriole aids in the formation of sperm axoneme and doesn’t function as centriole. After fertilization an active zygotic centrosome is formed, with some maternal input around the sperm centriole, which duplicates at the pronuclear stage and forms sperm aster. The formed sperm aster is essential for pronuclear development, migration of pronuclei toward syngamy and the formation of the mitotic spindles.
The two pronuclei (PN) formed in the zygote contain nuclear precursor bodies (NPBs), their number and arrangement can be used as an indicator of embryonic development potential (7). The NPBs migrate and merge to form the nucleoli in a time dependent manner. The pre-RNA synthesis in the nucleoli is important for translational processes when the embryonic genome is activated.
The last step of fertilization process before the first cleavage is called syngamy. After the formation of the male and female pronucleus, they start to gradually migrate toward the center of the oocyte. The chromatin of each PN rotates to face each other placing the centrosome between them. The nuclear envelops dissolve few hours before the first mitotic division and the two sets of chromosomes from each PN pair in syngamy, align on the newly formed mitotic spindles and are ready for segregation during the first mitotic division.
6.3 IVF or ICSI in Case of Non–Male Factor Infertility
ART treatments in the 1970s and 1980s consisted of conventional IVF to treat tubal factor infertility in combination with non–male factor infertility. Only after the introduction of ICSI in 1992, a tremendous decrease was noted in the use of conventional IVF to treat non–male factor infertility – with some centers performing almost exclusively ICSI, despite the lack of any evidence of a benefit of ICSI over conventional IVF (Figure 6.1). Couples with non–male factor infertility according to the WHO criteria (<1 ×106/mL round cells, concentration >15 × 106/mL, total motility >40 percent and progressive motility >32 percent; with a progressive motility >65 percent after capacitation, >4 percent normal morphology) (8), can easily undergo conventional IVF as the sperm velocity is sufficient for cumulus-zona penetration. On the other hand, in case of ICSI, rapidly progressive, morphologically normal sperm can be selected as well. This is translated into equal or improved fertilization rates and equal or improved blastocyst formation following conventional IVF. In case of negative selection during preimplantation development, this is attributed to the selection of abnormal sperm for ICSI which may result in low fertilization, abnormal cleavage, and developmental arrest. Also important, most prospective and retrospective studies have failed to demonstrate higher proportions of total fertilization failure after IVF.
Figure 6.1 ICSI and conventional IVF. (A–C) Representation of ICSI and (D–F) conventional IVF. (A) Selection of the sperm for ICSI. (B) Position of the oocyte with the polar body at 6 o’clock and the injection needle with sperm (arrow) at 3 o’clock. (C) Mature oocyte after injection of the sperm (arrow) in the cytoplasm. (D) Cumulus oocyte complex after insemination with sperm on day 0 (sperm not visible). (E) Fertilized oocyte on day 1 with disintegrated cumulus. (F) Fertilized oocytes with a low number of spermatozoa attached to the zona pellucida.
These improved fertilization and developmental outcomes can be explained by the fact that conventional IVF facilitates a more “natural” selection of biologically fit sperm as compared to ICSI. During conventional IVF, the sperm is first exposed to the cumulus cells and it has been suggested that the cumulus has sperm chemotaxis properties, playing an important physiological role in the selection of functionally and genetically competent sperm. Different concerns have been raised – but not proven conclusively – regarding safety of the ICSI technique, that also explain the absence of improved outcomes after ICSI: (1) the sperm is chosen based solely on the embryologists’ subjective opinion of sperm phenotypic traits with no knowledge of the sperm genetic quality; (2) physical or biochemical disturbance of the ooplasm or the meiotic spindle; (3) errors in the selection of the injection site by inaccurate positioning of the injection needle with respect to the meiotic spindle; (4) the injection of biochemical contaminants; and (5) the injection of foreign sperm-associated exogenous DNA. Different selection methods have been tested to select the sperm with the highest fertilization potential for ICSI, like MACS or cumulus oocyte selected spermatozoa, however, in case of non–male factor infertility, conventional IVF allows the best selection of the most competent sperm.
Currently, the best treatment option in case of normozoospermia is conventional IVF, especially in patients undergoing their first ART treatment. To prevent total fertilization failure – be it after conventional IVF or after ICSI – in the first cycle, a split between IVF and ICSI can be recommended.
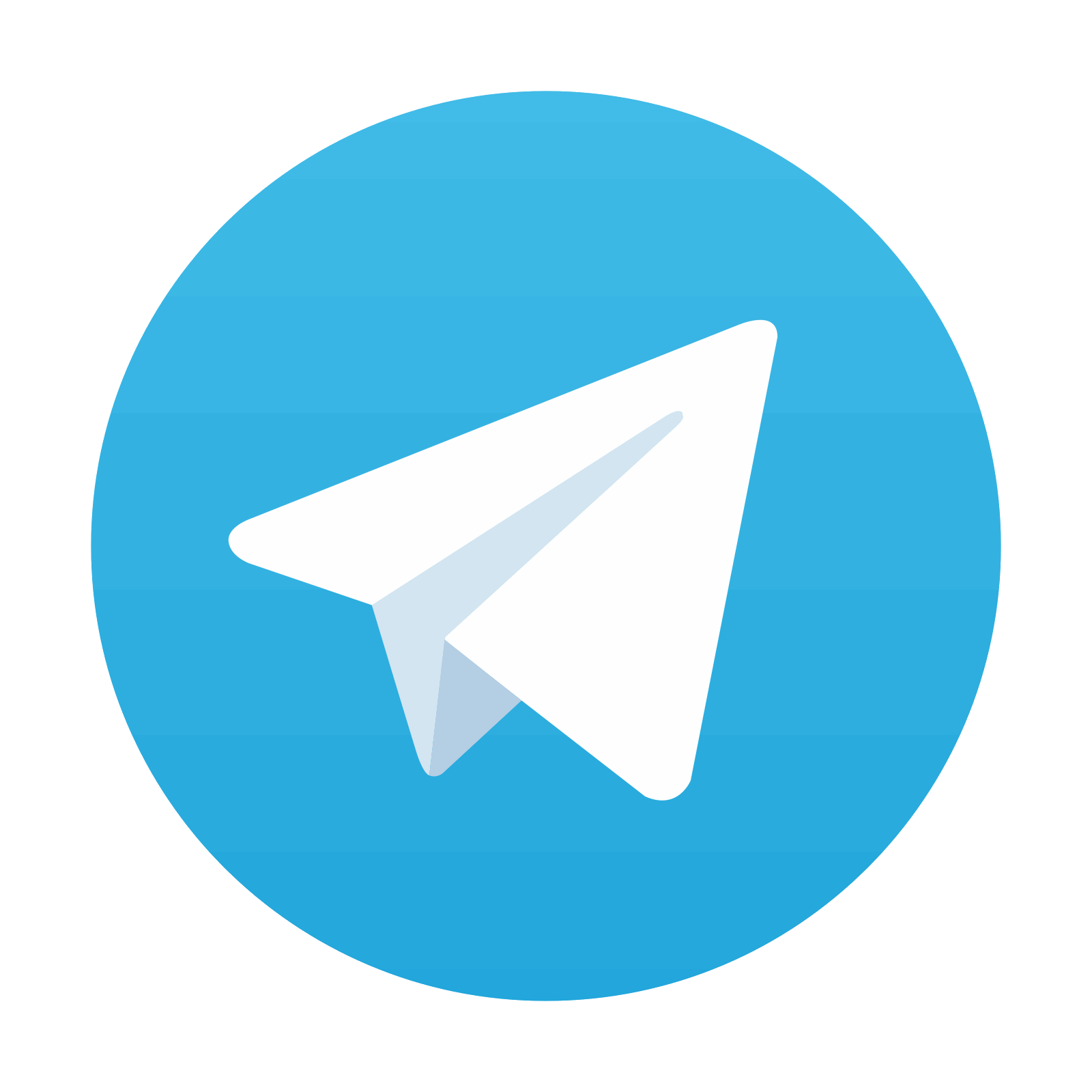
Stay updated, free articles. Join our Telegram channel

Full access? Get Clinical Tree
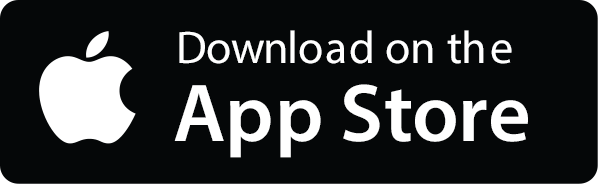
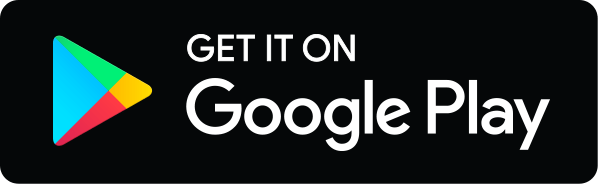