Abstract
Approximately 8–15 percent of couples are unable to conceive after 1 year of unprotected intercourse, and they are thereby considered infertile; this problem has become a global health concern, affecting roughly 187 million couples worldwide (). Male factor infertility occurs in 40–50 percent of the infertile couples, being solely responsible in approximately 20 percent of cases and in association with a female factor in another 20–30 percent. Although the male factor infertility is traditionally defined by the presence of abnormal semen parameters, it can nowadays be considered even when the seminal analysis is normal but with abnormal functional sperm tests ().
5.1 Introduction
Approximately 8–15 percent of couples are unable to conceive after one year of unprotected intercourse, and they are thereby considered infertile; this problem has become a global health concern, affecting roughly 187 million couples worldwide (1). Male factor infertility occurs in 40–50 percent of the infertile couples, being solely responsible in approximately 20 percent of cases and in association with a female factor in another 20–30 percent. Although the male factor infertility is traditionally defined by the presence of abnormal semen parameters, it can nowadays be considered even when the seminal analysis is normal but with abnormal functional sperm tests (2).
In the past 20 years, sperm concentration and total sperm count have decreased among young men, male partners in infertile couples, fertile men, and semen donors. In several countries, the sperm concentration levels of several young men have been associated with an increase in time to pregnancy. The reasons for this worsening are poorly established; however, environmental factors are likely to have an impact in this decline (3). The risk factors of male factor infertility may include environmental and lifestyle factors (e.g., smoking, pesticides, radiation, and excessive mobile phone use), local conditions (e.g., varicocele, genitourinary infection, epididymo-orchites, and testicular trauma), and systemic conditions (e.g., diabetes, cancer, medications, and systemic infections). Moreover, the advanced age of the males is associated with a decrease in sperm function and accumulation of sperm genomic damage; male fertility also declines as the male age increases, although not as important as the decline in fecundability with increasing maternal age (1). The same report mentioned that along with this decline observed in the last decades, diagnostic and treatment modalities for infertile men have improved. For instance, physicians now examine not only the traditional semen analysis but also the sperm function and genomic and proteomic expression of seminal plasma proteins; the surgical techniques applied to male infertility problems have also been refined.
In the present chapter, the most recent scientific evidence on male infertility diagnosis and management is reviewed, aiming for the individualized management of infertile patients and an improvement of reproductive outcomes.
5.2 Sperm Physiology and Sperm DNA Integrity
Understanding the sperm physiology and the adequate functioning of the hypothalamic-pituitary-gonadal (HPG) axis is critical for the assessment and management of infertile male patients. Male fertility in humans depends on the continuous daily production of millions of spermatozoa and on adequate spermatogenesis and steroidogenesis. Spermatogenesis is a highly efficient and coordinated process that deals with the production and development of spermatozoa. The entire spermatogenic process requires approximately 74 days, but a more recent study concluded that the total time to produce sperm in normal men might vary between 42 and 76 days, taking approximately 64 days. Every 16 days, a new group of spermatogonia enters the spermatogenic process (4). Spermatogenesis depends on the proper functioning of the HPG axis, which is regulated through the release of gonadotropin-releasing hormone (GnRH). Hypothalamic neurons secrete GnRH in a pulsatile form. Then, GnRH is transported to the anterior compartment of the pituitary gland where it will bind to specific receptors located in the gonadotrophic cells to modulate the synthesis and secretion of pituitary gonadotropins, namely, the follicle-stimulating hormone (FSH) and luteinizing hormones (LH). These hormones are secreted into the systemic circulation and act on the testes. While FSH acts via receptors located in the Sertoli cells, LH stimulates the Leydig cells to produce testosterone, which is an essential prerequisite for male fertility and maintenance of spermatogenesis. Subsequently, steroids and gonadal peptides are secreted and transported to the systemic circulation to modulate the secretion of hypothalamic and pituitary hormones. Thus, spermatogenesis depends on the high levels of intratesticular testosterone and FSH stimulation of the Sertoli cells (5).
Spermatogenesis is classically divided into the following three phases: (1) proliferative or mitotic phase – the primitive germ cells/spermatogonia pass through a series of mitotic divisions, forming new stem cells or cells that will produce the spermatocytes; (2) meiotic phase – the spermatocytes pass through two consecutive divisions producing the spermatids (haploid cells); and (3) spermiogenesis – spermatids differentiate into spermatozoa (6). During the spermatogenesis, significant morphological and functional changes occur in the nucleus. In round spermatids, the nucleus is found in a central position; however, as the spermatid lengthens, it moves to a more eccentric position and undergoes a significant condensation, reaching the size of 10 percent of the initial, favoring hydrodynamics of the sperm. The sperm chromatin has a highly organized and compact structure, consisting of DNA and heterogeneous nucleoproteins. At this point, the chromatin is insoluble and condensed. Such chromatin characteristics are essential to protect genetic integrity during the transport of the paternal genome through the male and female reproductive tracts. Chromatin also ensures that the paternal DNA is delivered in the oocyte enabling the adequate fusion of two gametic genomes and allows that the developing embryo correctly expresses the genetic information (6).
Chromatin compaction and nucleoprotein composition change at the molecular level. In round spermatids, nuclear DNA is arranged around nucleosomes that are composed of histones, as well as in other somatic cells. However, in mature spermatozoa, histone is replaced by protamine, which is a small arginine-rich protein. Protamination is responsible for both higher condensation of the sperm DNA and higher resistance to denaturation, considering that protamines have a higher affinity for DNA than histones. Hypotheses were raised for the function of protamines, stated as follows: condenses the spermatic nucleus, transforming into a more compact and hydrodynamic form; protects the genetic code derived from the spermatozoid; participates in the process of maintenance and repair of sperm DNA integrity; and correlates with the spermatic genetic imprint. For a spermatozoon to be fertile, it must be capable of undergoing decondensation at an appropriate time during fertilization. Thus, the normal sperm chromatin structure and the condensation and decondensation processes are essential for sperm fertilizing ability (7).
Protamination of sperm DNA is essential in the compaction of paternal genome during its transportation to the female genital tract; the DNA integrity is also vital for the accurate transmission of paternal genetic information. Furthermore, the importance of DNA integrity in fertilization, early embryo development, implantation, and pregnancy outcomes has been supported by various in vivo and in vitro studies. Alteration in spermatogenesis, varicocele, inflammatory processes, and other issues related to an increase in oxidative stress may be associated with sperm DNA integrity defects, leading to functional modifications in the sperm that may impact the reproductive outcome in a natural or even assisted conception (7).
Finally, the physiologic levels of reactive oxygen species (ROS) are necessary for adequate sperm capacitation and maturation. However, abnormally elevated amounts of ROS may adversely impair and damage the function of several enzymes and induce lipid peroxidation, causing sperm DNA fragmentation (SDF). Natural antioxidants may act as scavengers of excessive ROS, helping in maintaining a natural redox balance. However, when an imbalance occurs due to excessive ROS production or a reduction in antioxidant activity, an excess of oxidative stress (OS) will occur. Regarding the impact of OS on male fertility, the semen samples of infertile men present higher levels of ROS than those of fertile men (1). Sperm DNA damage is deemed promutagenic, leading to mutations after fertilization as the oocyte attempts to repair the DNA damage before initiating the first cleavage. As speculated, these mutations may be responsible for the induction of infertility, miscarriage, childhood cancer in the offspring, and also a higher risk of imprinting diseases but will be fixed in the germline (4).
5.3 Evaluation
When pregnancy has not occurred after 12 months of unprotected intercourse or after 6 months of failed attempts with a >35-year-old female partner, the male partner must undergo screening evaluation as well. When medical history and/or physical findings are related to specific male infertility, these male partners may be evaluated earlier. The male factor infertility investigation aims to determine the following: (1) potentially reversible conditions; (2) irreversible conditions that are amenable to assisted reproductive techniques (ART) using the sperm of the male partner; (3) irreversible conditions that are not amenable to ART, with sperm donor or adoption as alternatives; (4) life-threatening conditions, such as testicular cancer, that may underlie the infertility and require specific medical treatment; and (5) genetic abnormalities that may impact on child’s health if ART is to be employed, allowing to perform a genetic counseling. An appropriate male evaluation may allow the couple to better understand the basis of their infertility. Detection of conditions for which no treatment is available will make couples undergo ineffective therapies. At a minimum, the infertility work-up must include a detailed history, physical examination, and two separate semen analyses to identify the risk factors related to male infertility. Thereafter, specific evaluation must be performed according to the findings of the initial evaluation, allowing the identification of wide-ranging causes of male infertility (2).
5.3.1 Semen Analysis
The semen analysis is the cornerstone on the male factor infertility evaluation, and important treatment decisions are based on the results of the semen analysis. This analysis has been standardized by the World Health Organization (WHO). Its latest references were published in 2010, with a manual for the examination and processing of human semen, establishing the reference limits that should be considered normal when performing a semen analysis (8). If the results of semen analysis are normal, a single test is sufficient. When at least two semen analyses exhibited abnormal results, further andrological evaluation is required (8).
When comparing the last three WHO seminal references that were published in 1992, 1999, and 2010, the latest version established new references that are markedly lower than those previously reported (Table 5.1). The most important change in the references is concerning on the criteria for sperm morphology evaluation. In the last two versions, the Tygerberg (Kruger) criteria were used to evaluate morphology. The morphology judged by Kruger’s strict criteria correlated with the fertilization potential of the sperm (9). Kruger et al. observed that when the morphology is <14 percent according to their criteria, the fertilization rate per oocyte is impaired compared with higher percentage levels. When normal morphology is <4 percent, fertilization will be severely impaired. In performing classical in vitro fertilization (IVF), when the normal morphology was <4 percent, the fertilization rate was 7.6 percent; when the normal morphology range was 4–14 percent, it was 63.9 percent; and when the morphology was >14 percent, it was within the normal range of the IVF laboratory (9).
Table 5.1 Semen parameters according to World Health Organization references from 1992, 1999, and 2010
1992 | 1999 | 2010 | |
---|---|---|---|
Volume (mL) | ≥2 | ≥2 | ≥1.5 |
Count (106/mL) | ≥20 | ≥20 | ≥15 |
Total count (106) | ≥40 | ≥40 | ≥39 |
Motility (%) | ≥50 | ≥50 | ≥40 |
Progressive (%) | ≥25 (a) | ≥25 (a) | ≥32 |
Vitality (%) | ≥75 | ≥75 | ≥58 |
Morphology (%) | ≥30 | 14 (Tygerberg) | ≥4 (Tygerberg) |
Leukocytes (106/mL) | <1.0 | <1.0 | <1.0 |
Note. Boldface indicates the reference values that have been updated from one to the next version of WHO parameters.
Although the semen analysis has been standardized by WHO and the 2010 WHO reference values have been used worldwide as reference, numerous concerns regarding these values have been raised. Despite using controlled studies involving couples with known time to pregnancy (lower than 12 months) to establish new limits, these studies are limited in terms of the population (i.e., 1953 semen samples from only seven countries worldwide) analyzed, the methods used for semen evaluation (studies using different morphology criteria), and having only one sample from each patient was evaluated. According to the fifth edition WHO manual for semen analysis, the fifth centile was the power limit of semen characteristics. Thus, for sperm count, the WHO normal value is 15.0 × 106/mL. However, if we evaluated the 50th percentile, a value into which 50 percent of the reference population of “fertile” men falls, and the sperm count would increase to 73.0 × 106/mL (10).
5.3.2 Endocrine Evaluation
Although causes of male infertility remain uncommon, hormonal abnormalities of the hypothalamic-pituitary-testicular axis are well recognized. Some clinicians advocate that all infertile patients should undergo endocrine evaluation; however, endocrine disorders are rare in men with normal sperm parameters (2). Thus, the evaluation is indicated for men presenting the following: (1) sperm concentration <10 million/mL; (2) impaired sexual function; or (3) clinical findings suggestive of any endocrinopathy (4). Initially, hormonal evaluation should be performed with serum FSH and total testosterone measurements. When the total testosterone level is <300 ng/mL, additional evaluation must be performed, including a new testosterone measurement, LH, and prolactin (PRL). A normal FSH level does not exclude impairment in spermatogenesis, but markedly elevated FSH levels are indeed associated with abnormal spermatogenesis (2).
5.3.3 Radiographic Tests
Scrotal ultrasound should not be performed as a routine screening procedure, given that most scrotal pathologies can be identified by a careful examination. However, it is indicated in patients with a history of scrotal surgery, difficulty in genital examination that does not allow the adequate evaluation of related genital diseases, and suspected varicocele recurrence. It also must be performed for men presenting with infertility and testicular cancer risk factors, such as cryptorchidism or a previous testicular neoplasm (2, 11).
Transrectal ultrasound (TRUS) is indicated in patients with low semen volume with/without azoospermia, fructose negative, or an acidic semen. TRUS depicting dilated seminal vesicle (>1.5 cm in diameter), dilated ejaculatory ducts (>2 mm in diameter), and/or mid-line cystic prostatic structures suggests the diagnosis of complete or partial ejaculatory duct obstruction (11).
Pituitary magnetic resonance imaging (MRI) should be performed in cases of true prolactinemia (PRL > 2× normal reference values). Meanwhile, pelvic/scrotum MRI is indicated in cases of equivocal TRUS, suspicious testicular lesion, and undescended testis. Nowadays, vasography has not been often performed, but it can be performed to rule out obstruction; it is also generally performed during a reconstructive surgery (11).
5.3.4 Genetic Evaluation
Genetic abnormalities may be associated with problems in the production and transport of sperm and may be present in the form of chromosomal, genetic, nucleotide, or epigenetic modifications, representing as one of the most clinically important aspects of male factor infertility (4). Karyotype and Y chromosome analysis is recommended in men with nonobstructive azoospermia (NOA) or severe oligozoospermia (<5 million/mL) (2, 8).
Compared with fertile men, patients with NOA or severe oligozoospermia have a higher risk of exhibiting a genetic abnormality. Although many patients with azoospermia and oligozoospermia present with a genetic predisposition to infertility, the cause in most cases remains unknown. Among the known genetic causes of male infertility are numeric and structural chromosomal abnormalities, Y chromosome microdeletions, X-linked inheritance, and autosomal gene mutations (2, 8). The most common sex chromosome abnormality observed in infertile men is Klinefelter syndrome (47, XXY). Autosomal karyotype abnormalities may also be present, and the most common are Robertsonian translocations, reciprocal translocations, paracentric inversions, and marker chromosomes (8).
Moreover, Y chromosome microdeletions are presented anywhere from 1 in 2000 to 1 in 3000 men. However, this incidence increases to rates of around 7 percent among infertile men with severely impaired spermatogenesis and to 16 percent among patients with azoospermia, though marked differences are reported in different areas worldwide. In general, patients having a Y chromosome microdeletion are asymptomatic, though they may manifest a reduced testicular volume. A specific region on the long arm of the Y chromosome (Yq11) contains 26 genes related to spermatogenesis, the so-called azoospermia factor (AZF) regions. These genes are organized into three distinct locations as follows: the AZFa, AZFb, and AZFc regions. Deletions in these regions, which may occur independently or in association, may lead to severe oligozoospermia or even azoospermia. The effect on spermatogenesis depends on the affected AZF subregions. The most frequent microdeletion subtype is the AZFc region, which accounts for 80 percent of AZF microdeletions. Furthermore, AZFb occurs in 15 percent of Y chromosome microdeletions, whereas AZFa is rare and accounts for <3 percent. Sperm-retrieval procedures should be avoided in patients with AZFa, and a controversy exists as to whether it should be performed in patients with AZFb and AZFab; additionally, sperm can be found in around 50–70 percent of patients with AZFc (12).
Most men with congenital bilateral absence of vas deferens (CBAVD) present an abnormality of the cystic fibrosis transmembrane conductance regulator (CFTR) gene, which is located on chromosome 7. Almost all men with clinical cystic fibrosis have CBAVD, and 80 percent of them manifest CFTR gene mutations. However, failure to identify a CFTR abnormality in a male patient with CBAVD does not exclude a mutation entirely, considering that 10–40 percent are undetectable using common clinically available methods. These mutations are also increased in men with congenital bilateral obstruction of the epididymis and those with unilateral vasal agenesis. Importantly, before any treatment using sperm from a patient with CBAVD or congenital unilateral absence of the vas deferens, the female partner of such patient can also be tested to exclude the possibility of being a carrier of the same mutation and to determine the risk of conceiving a child affected by cystic fibrosis (2, 13).
5.3.5 SDF Test
Although the sperm with fragmented DNA can efficiently fertilize an egg with the same efficiency as the sperm without DNA fragmentation, SDF negatively affects embryo quality, impairing the embryo development and compromising the integrity of the embryonic genome. Sperm DNA damage is also associated with spontaneous recurrent miscarriage. Conventional semen analysis is slowly giving space to sperm function tests that measure OS and SDF (4, 14). Thus, although conventional semen analysis continues to be the cornerstone on the assessment of male fertility potential, the sperm DNA damage found in sperm function tests in several cases may indicate subfertility despite the semen analysis results (4). According to a recent published clinical practice guideline, the SDF testing should be performed in the following: (1) male patients with risk factors for OS, including lifestyle conditions (e.g., smoking, obesity, and metabolic syndrome), varicocele, genital infections, advanced age, and toxicant exposure (e.g., environmental, licit or illicit drugs, radiation and chemotherapy); (2) after failed intrauterine insemination (IUI), IVF, or ICSI cycles, provided that no other apparent reasons exist to explain the failure; (3) couples with unexplained infertility; and (4) couples with recurrent miscarriage (Figure 5.1) (7).

Full access? Get Clinical Tree
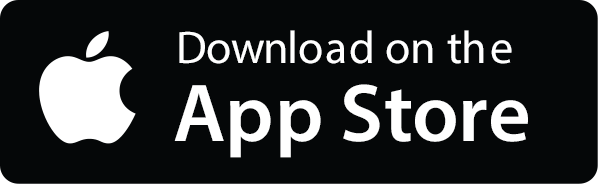
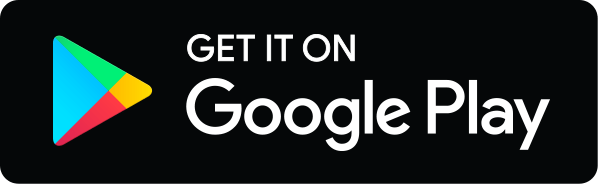