Abstract
According to the European Society of Human Reproduction and Embryology (ESHRE) Working Group on Oocyte Cryopreservation [], between 2010 and 2014, cancer patients in Europe underwent similar numbers of ovarian tissue cryopreservation procedures (~4,400) and oocyte cryopreservation procedures (~3,800). This milestone in the field of ovarian tissue cryopreservation is responsible for a new surge of interest in primordial follicles and their requirements for survival and development, as they represent more than 90% of the follicle population to be cryopreserved. When patients cannot have their frozen-thawed ovarian tissue reimplanted due to the risk of transmission of malignant cells, these follicles can be isolated and grafted inside a bioengineered ovary (see Chapter 36) or grown in vitro (see Chapter 31). We will focus on the latter strategy, more specifically on the main elements that primordial follicles need to survive in vitro.
According to the European Society of Human Reproduction and Embryology (ESHRE) Working Group on Oocyte Cryopreservation [1], between 2010 and 2014, cancer patients in Europe underwent similar numbers of ovarian tissue cryopreservation procedures (~4,400) and oocyte cryopreservation procedures (~3,800). This milestone in the field of ovarian tissue cryopreservation is responsible for a new surge of interest in primordial follicles and their requirements for survival and development, as they represent more than 90% of the follicle population to be cryopreserved. When patients cannot have their frozen-thawed ovarian tissue reimplanted due to the risk of transmission of malignant cells, these follicles can be isolated and grafted inside a bioengineered ovary (see Chapter 33) or grown in vitro (see Chapter 29). We will focus on the latter strategy, more specifically on the main elements that primordial follicles need to survive in vitro.
Primordial Follicle Requirements to Survive In Vitro
It is not yet known how and why some primordial follicles are able to survive and even remain in a quiescent state throughout a woman’s life, while others undergo atresia before being activated. Indeed, mechanisms involved in depletion of the primordial follicle pool throughout adult life have not been elucidated [2]. In vitro culture studies rely only on scarce information obtained from animal species like rodents, as well as empirical observations, to keep follicles alive during culture.
Achieving in vitro survival of primordial follicles presents the unique challenge of mimicking the dynamics of the ovarian environment. While the ovary is responsible for preserving the three-dimensional (3D) structure of follicles, follicle-follicle and follicle-cell crosstalk, oxygenation and synthesis of factors involved in follicle survival, the body supplies various nutrients and factors required by follicles. Therefore, when culturing human primordial follicles in vitro, all these features must be taken into account.
In Vitro Culture of Primordial Follicles Enclosed in Ovarian Tissue
In order to maintain the 3D structure of follicles and not disturb their interaction with the surrounding microenvironment, some research groups opt to in vitro culture primordial follicles enclosed in ovarian tissue. Indeed, preserving primordial follicles with their surrounding interstitial ovarian tissue allows stromal cells to interact with follicles, which may be crucial for follicle survival and development.
While in vitro tissue culture eliminates endocrine and paracrine processes involved in regulating primordial follicle survival and activation, the surrounding environment of primordial follicles has an impact on their survival, activation and development, as it may affect their response to stimulatory and inhibitory factors [3]. Table 32.1 summarizes existing studies on in vitro culture of human primordial follicles enclosed in ovarian tissue fragments, detailing the size of cultured ovarian fragments and main outcomes.
Despite the promising results obtained with this approach [4–35], one must be aware that diffusion of nutrients and waste metabolites and the process of gas exchange can prove challenging during in vitro culture of human ovarian cortex. For this reason, small, thin fragments of tissue (~1 mm²) are most suitable, as they offer a larger ratio of surface area to volume and allow better contact with media and more efficient gas exchange [37]. Scott et al. [17] reported that in vitro culture of small ovarian tissue cubes (1–2 mm³) yielded higher follicle survival rates than larger ovarian fragments (1–2 × 5–8 mm).
Most studies do not actually specify the proportion of primordial follicles remaining at the end of in vitro culture, nor indeed if they were present during long periods of culture. When ovarian tissue is incubated for more than 2 days, the primordial follicle pool is depleted by atresia and follicle activation (Table 32.1). These two phenomena occur to a greater or lesser extent depending on culture conditions, ovarian tissue donor age and intrinsic characteristics of the ovary. For instance, activation of primordial follicles is higher in ovarian tissue from adult women than prepubertal girls [32]. Lower recruitment rates in younger ovaries may be the result of inhibitory factors produced by primordial follicles, which prevent activation of surrounding follicles [38]. Since there are more primordial follicles in these ovaries, higher concentrations of these factors would be present in stromal tissue [32]. Data from the literature show that in vitro culture can support primordial follicle survival for up to 63 days in fetal ovarian tissue fragments [23], and four weeks in their adult counterparts [6, 20, 27].
Table 32.1 Studies on in vitro culture of human primordial follicles enclosed in ovarian tissue fragments
Size of tissue fragment | Medium supplementation | IVC period | Main outcomes | Ref. |
---|---|---|---|---|
Small pieces (0.1–0.3 mm thick) | EBSS with pyruvate (or α-MEM) + human serum, FSH, LH and insulin | 4–21 days |
| [8] |
Small pieces (1–3 mm thick) |
| 5, 10 or 15 days |
| [9] |
Small pieces (0.1–0.3 mm thick) | EBSS + antibiotics, human serum, FSH and pyruvate | 7–28 days | Follicle activation and growth were observed, but primordial follicles were still found after 28 days. | [10] |
Small pieces (1–3 mm thick) | EBSS + antibiotics, human serum, FSH and pyruvate with or without insulin (or IGF-I or IGF-II or insulin + IGF-I + IGF-II) | 7 or 14 days |
| [11] |
1 mm³ | DMEM + antibiotics, FBS, L-glutamine, β-mercaptoethanol, NAA and FSH | 6 weeks | Follicle survival was observed. | [12] |
~1 mm³ | α-MEM + antibiotics, HSA (or FBS), ITS, 8-br-cGMP and FSH with or without GDF-9 | 7 or 14 days |
| [13] |
0.3–0.5 mm |
|
|
| [4] |
0.8 × 0.8 × 0.8 mm | DMEM + antibiotics, FBS, ITS, β-mercaptoethanol, EGF and FSH | 21 days | Follicle survival was observed after IVC only in ovarian tissue vitrified using ethylene glycol. | [14] |
0.5–1 mm | EBSS + FBS and FSH | 4 weeks | Follicle development was noted, but there was a decrease in follicle survival rates throughout IVC. | [15] |
0.3–0.5 mm | Ham’s F-10 + antibiotics, FSH, insulin and HSA, with or without T (or DHT or 17β-estradiol) | 24 hours | DHT had a positive effect on tissue survival. | [16] |
1–2 × 5–8 mm or 1–2 mm³ | α-MEM + antibiotics, HSA, FSH, ITS and 8-br-cGMP | 7 or 14 days |
| [17] |
1–2 mm³ | α-MEM + antibiotics, HSA, FSH and ITS, with or without 8-br-cGMP | 7 or 14 days |
| [18] |
1–2 mm³ | EBSS + antibiotics, pyruvate and human serum, with or without 8-br-cAMP | 7, 14 or 21 days |
| [19] |
Small pieces | α-MEM + antibiotics, glutamine, ITS, EGF, FSH, LH and HSA, with or without testosterone and/or AMH | 4 weeks |
| [20] |
Slices (0.5–1 mm thick) | EBSS + antibiotics, HSA (or human serum) and FSH, with or without KL (or ACK2) | 7 or 14 days |
| [21] |
1–2 mm³ | α-MEM + antibiotics, HSA, FSH, 8-br-cGMP, ITS and AMH | 7 days |
| [22] |
1 × 1 × 5 mm | Iscove’s modified Dulbecco’s medium + antibiotics, FBS, ITS, EGF and FSH | 2 or 6 weeks | Follicles survived for up to 2 weeks. By 6 weeks, all follicles had degenerated. | [5] |
1 × 1 mm² | Serum-free medium + antibiotics | 7–14, 21–35 or 63 days | Primordial follicle populations decreased due to atresia and activation, but follicles were still found after 63 days. | [23] |
1 × 1–1.5 × 0.7–1 mm | AIM-V medium | 14 days | Higher rates of follicle survival were seen in fresh and slow-frozen-thawed ovarian fragments compared to quick-frozen tissue. | [24] |
2 × 2 mm | α-MEM + antibiotics, FBS, pyruvate, FSH and HCG | 3, 5, 7, 9, 11, 13 or 15 days | Primordial follicles were cultured in high oxygen concentrations, but there was a gradual decrease in primordial follicle survival. | [25] |
~0.5 mm³ | McCoy’s 5a medium + bicarbonate supplemented with HEPES, antibiotics, BSA, glutamine, ITS and ascorbic acid | 6 days | Primordial follicle populations decreased after 6 days due to both degeneration and activation. | [26] |
1×1 mm² | Defined Keratinocyte-SFM culture medium | 7, 14, 21 or 28 days | Primordial follicle populations decreased after 28 days due to both degeneration and activation. | [6] |
1–2 mm |
| 1–4 weeks |
| [27] |
~2 × 1 × 1 mm | α-MEM + FSH, HSA and ITS | 1 or 2 weeks |
| [28] |
1–2 mm | α-MEM + FSH, HSA and ITS, with or without GDF-9 (or BMP-15) | 1–4 weeks | Primordial follicles were still found after 4 weeks. GDF-9 and BMP-15 increased follicle activation, but follicle atresia was higher with BMP-15 than GDF-9. | [29] |
0.5 mm | McCoy’s 5a medium + bicarbonate supplemented with HEPES, antibiotics, BSA, glutamine, ITS and ascorbic acid, with or without rapamycin | 6 days | Rapamycin induced oocyte loss. | [30] |
Slices (<1 mm or 1–2 mm thick) | α-MEM + FSH, HSA and ITS, with or without bpV (or 740Y-P) | 6 or 12 days | Primordial follicles were still found after 1 week in groups cultured with basic medium or supplemented with 740Y-P. Tissue cultured with bpV supplementation resulted in greater follicle atresia. Similarly, higher atresia rates were found after 12 days. | [31] |
~3 × 1 × 0.5 mm | McCoy’s 5a medium + bicarbonate supplemented with HEPES, antibiotics, BSA, glutamine, ITS and ascorbic acid | 6 days | Higher levels of primordial follicles were observed in prepubertal tissue because of lower activation compared to pubertal and adult ovaries. | [32] |
1 × 1 × 0.5 mm | α-MEM-Glutamax + antibiotics, HSA, bovine fetuin, ITS and FSH | 1–6 weeks | Follicle survival was still seen after 6 weeks, despite an increase in follicle atresia. | [33] |
~2 × 1 × 1 mm | α-MEM + FSH, HSA, ITS and 4hc (or PM) | 24 or 48 hours | Cyclophosphamide metabolites (4hc and PM) had a positive impact on primordial follicle activation. | [34] |
Small pieces | α-MEM + FSH, HSA and ITS, with or without LIF (or LIF + anti-LIFNA) | 6 days | Despite high atresia rates, primordial follicles survived and some were activated. Different matrices tested for culture had no particular effect. | [35] |
Slices (~0.5 mm thick) | α-MEM + antibiotics, BSA, glutamine, ITS, ascorbic acid | 6 or 9 days | Higher follicle survival rates were found in ovarian tissue samples cultured in gas-permeable dishes containing high volumes of medium. | [36] |
1 × 1 × 0.5 mm³ | McCoy’s 5a medium + bicarbonate supplemented with HEPES, antibiotics, BSA, glutamine, ITS and ascorbic acid | 8 days | Primordial follicles were observed after 8 days, with both follicle activation and growth detected. | [7] |
4hc: 4-hydroperoxycyclophosphamide; 8-br-cAMP: 8-bromoguanosine cyclic adenosine 3’, 5’-monophosphate; 8-br-cGMP: 8-bromoguanosine 3’,5’-cyclic monophosphate; α-MEM: minimum essential medium alpha; AAA: non-essential amino acids; ACK2: anti-c-kit antibody; AMH: anti-Müllerian hormone; bFGF: basic fibroblast growth factor; BMP-15: bone morphogenetic protein 15; BSA: bovine serum albumin; cAMP: cyclic adenosine 3’,5’-monophosphate; DHT: dihydrotestosterone; DMEM: Dulbecco’s modified Eagle’s medium; EBSS: Earle’s balanced salt solution; EGF: epidermal growth factor; FBS: fetal bovine serum; FSH: follicle-stimulating hormone; GDF-9: growth differentiation factor 9; HCG: human chorionic gonadotropin; HEPES: N-2-hydroxyethylpiperazine-N’-2-ethanesulfonic acid; HSA: human serum albumin; IGF: insulin-like growth factor; ITS: insulin, transferrin and selenium; IVC: in vitro culture; KL: kit ligand; LH: luteinizing hormone; LIF: leukemia inhibitory factor; LIFNA: leukemia inhibitory factor neutralizing antibody; NAbFGF: neutralizing antibody against basic fibroblast growth factor; NAC: N-acetyl-L-cysteine; PM: phosphoramide mustard; T: testosterone.
It is also important to bear in mind that due to the dense and fibrous nature of human ovarian cortex [39], assessing the same follicle population before and after in vitro culture is simply not feasible. Indeed, it is not possible to identify small primordial follicles in tissue fragments during in vitro culture, which hampers evaluation of the success rate of the strategy. For this reason, studies often calculate follicle survival by comparing a sample of ovarian tissue before in vitro culture with another after culture [3, 7]. However, such indirect appraisal is somewhat unreliable, as follicle distribution in human ovarian cortex is highly heterogeneous [40]. To conduct more accurate follow-up of follicles and assess their survival rate and fate during in vitro culture, vital dyes like neutral red can be used [41, 42]. Peters et al. [42] developed a procedure to easily detect pre-antral follicles in human ovarian tissue using neutral red and full-field optical coherence tomography. This fast noninvasive method allows selection of tissue fragments with high follicle density, avoiding in vitro culture of samples without follicles. Neutral red is a relatively nontoxic dye, but known to be a photosensitizer, forming reactive oxygen species in the presence of light [43, 44]. While its toxicity has not been investigated in human follicles, in vitro culture of bovine and macaque follicles has not demonstrated any toxic effect of this dye [45, 46].
In Vitro Culture of Isolated Primordial Follicles
Within tissue, follicles benefit from the original microenvironment that provides 3D support with adequate rigidity, and accommodates stromal cells, other follicles and even immune cells like macrophages. By contrast, for isolated follicles to survive and grow outside the ovary, the appropriate environment and conditions must all be supplied by the culture system.
Primordial follicles can also survive after isolation and in vitro culture [47–51]. The advantage of this alternative is direct monitoring of follicles during the culture period [52] and the possibility to assess the influence of bioactive factors on folliculogenesis. Because of the fibrous nature of human ovarian cortex, a combination of mechanical chopping and enzymatic digestion should be applied to successfully isolate primordial follicles [33, 47–52]. A number of proteolytic enzymes have been used to dissociate human ovarian interstitial tissue, such as different types of collagenase [47–49, 52], combinations of collagenase and deoxyribonuclease [47, 52], and commercial enzyme blends like Liberase [33, 49–51, 53] and tumor dissociation enzyme reagent [54]. More recently, Chiti et al. [55] developed a tailored follicle isolation protocol that can be adapted to different types of ovarian tissue, ranging from the softer consistency found in young women to a stiffer texture in older patients. This gentle protocol was shown to increase the number and quality of isolated follicles by decreasing the number of damaged follicles, while completely digesting whole ovarian fragments. Interestingly, it is also capable of isolating a higher proportion of primordial follicles, another great advantage.
It was initially believed that enzymatic digestion could compromise follicle integrity, which would have a negative impact on primordial follicle survival [56, 57]. For this reason, most studies on in vitro culture use growing pre-antral follicles that are larger and easier to isolate [37]. Only a few studies (Table 32.2) have attempted to in vitro culture isolated primordial follicles and two of them obtained negative results [33, 52]. However, recent reports from Amorim’s group show that the integrity of primordial follicles can be maintained and they can survive and develop in vitro [49, 51], or after encapsulation in fibrin and xenotransplantation [55, 57].
Follicle isolation technique | 3D system | Medium supplementation | Culture period | Main outcomes | Ref. |
---|---|---|---|---|---|
Cutting into 0.5–1 mm pieces and use of collagenase IX + DNase IV | Collagen gel | EBSS + antibiotics, sodium pyruvate, L-glutamine, FBS and FSH | 24 hours | Small follicles did not survive IVC. | [52] |
Cutting into 0.5–1 mm pieces and use of collagenase IX + DNase IV | Collagen, ECM or poly-L-lysine coating or collagen gel | EBSS + antibiotics, sodium pyruvate, L-glutamine, FBS (or human serum) and FSH | 24 hours | Fully isolated follicles, but not partially isolated follicles, grew in collagen gel, but not on coated inserts. | [47] |
Mincing with a tissue chopper and use of collagenase IA | 1% alginate | MEM-Glutamax + antibiotics, FBS, pyruvic acid and ITS | 7 days | Primordial follicles survived and showed increased follicle diameter. | [48] |
Mincing with a tissue chopper and use of Liberase DH or collagenase IA | 1% alginate | MEM-Glutamax + antibiotics, FBS, pyruvic acid and ITS | 7 days | Primordial follicles survived and showed increased follicle diameter. | [49] |
Mincing with a tissue chopper and use of Liberase DH | 1% alginate | MEM-Glutamax + antibiotics, FBS, pyruvic acid and ITS | 7 days | Viability and increased diameter were greater in follicles isolated from fresh samples and tissue cryopreserved with DMSO. | [50] |
Mincing with a tissue chopper and use of Liberase DH | 1% alginate | MEM-Glutamax + antibiotics, FBS, pyruvic acid and ITS | 7 days | Primordial follicles survived and were activated. | [51] |
Mincing with a tissue chopper and use of Liberase Blendzyme 3 | 0.5 or 2% alginate |
| 3 days | Primordial follicles did not survive IVC. | [33] |
BF: bovine fetuin; bFGF: basic fibroblast growth factor; DMSO: dimethyl sulfoxide; DNase: deoxyribonuclease; EBSS: Earle’s balanced salt solution; ECM: extracellular matrix; FBS: fetal bovine serum; FSH: follicle-stimulating hormone; IVC: in vitro culture; MEM: modified Eagle’s medium; ITS: insulin, transferrin and selenium.
The isolation procedure strips primordial follicles from their surrounding cells and extracellular matrix, but they need neighboring somatic cells and other follicles to survive and develop. Indeed, in their natural environment, primordial follicles are found in clusters at the periphery of the cortex surrounded mainly by stromal cells, which are responsible for supporting follicles and producing extracellular matrix components like collagen, glycoproteins and glycosaminoglycans. Furthermore, following as yet unknown stimuli, some of them differentiate into theca cells, which are essential to growing follicles. Thus, if isolated primordial follicles are to be developed in vitro, it may be beneficial to add somatic cells to enhance follicle survival and growth. These somatic cells will act as “feeder” cells, producing essential factors and removing toxic waste from the culture medium.
While no attempt has been made to in vitro culture isolated human primordial follicles with feeder cells, some types of somatic cells, including ovarian stromal cells, ovarian mesenchymal cells, cumulus cells, theca cells and embryonic fibroblasts, have been used to culture mouse pre-antral follicles [57–60]. All studies showed that these different types of cells, or media conditioned by them, promote follicle survival and development [59–61] and they could potentially exert a positive impact on primordial follicle survival as well.
Culturing primordial follicles in groups is also advantageous [62, 63]. It is known that the fewer primordial follicles there are in the ovary, the faster they will be activated and depleted. Current understanding suggests that signals regulating dormancy versus activation of primordial follicles originate from different compartments of the ovary, including neighboring follicles, stromal cells and other somatic cells. Isolating primordial follicles therefore disrupts this delicate signaling balance and causes follicles to activate, making them more likely to undergo atresia [63]. According to Hornick et al. [62], intact follicles growing together secrete factors that support survival and development of surrounding follicles, with a positive correlation between the number of follicles in the group and survival rates. This is probably one of the reasons why culturing isolated primordial follicles in groups yielded such high follicle survival [48–51]. Hence, to achieve the closely related environment that primordial follicles need in vitro, it is advisable to culture them in the presence of other follicles and somatic cells.
Matrices
3D Matrices to Encapsulate Isolated Follicles
Folliculogenesis is a complex process requiring constant crosstalk between the oocyte and granulosa cells, so achieving a perfect connection between these cells is essential. Indeed, destruction of the metabolic link between granulosa cells and oocytes would result in uncoordinated growth or even death of follicles [64]. Studies on in vitro culture of isolated human follicles have shown that maintenance of their 3D structure has a positive impact on their survival and development [48, 65].
Some of the first attempts to culture human primordial follicles in vitro were in two-dimensional systems [47]. This strategy was not able to sustain follicle survival, probably because it is not capable of maintaining the original structure of follicles. In order to preserve the morphology of follicles and support cell interaction, 3D systems are required. There are a number of natural and synthetic scaffold materials, such as alginate, collagen, fibrin, hyaluronan and poly(ethylene) glycol, which could potentially be utilized to in vitro culture isolated human primordial follicles and preserve their original structure [66], but only collagen and alginate have been tested so far [33, 47–52].
Collagen is the most abundant protein found in the human ovarian extracellular matrix [67], and collagen hydrogels have successfully served as 3D matrices for in vitro culture of various types of cells [68]. Apart from offering 3D support, this protein can promote cell viability, proliferation and development, thanks to several endogenous factors.
Abir’s group was the only one to report use of collagen for isolated human primordial follicles [47, 52]. Unfortunately, it is not possible to draw any conclusions on the applicability of this polymer, as they obtained discrepant results between studies; in the first, primordial follicles did not survive [52], and in the second, they survived and became activated [47]. While they did not investigate such differences, one can hypothesize that this could have been due to inherent batch-to-batch variability. Indeed, along with limited long-term stability and low levels of stiffness, inconsistency between batches is one of collagen’s main drawbacks [68].
Most studies on in vitro culture of isolated human primordial follicles have focused on alginate [33, 48–51]. Alginate is a polysaccharide constituted of two repeating monomer units, β-d-mannuronate and α-l-guluronate joined by β(1–4) linkage, derived from brown seaweed and bacteria. Like collagen, it has been widely used as a matrix in tissue engineering thanks to its biocompatibility and versatility [69]. Amorim’s group successfully cultured isolated human primordial follicles in 1% alginate [48–51]. Batches of around 10 isolated follicles were encapsulated in alginate and cultured for 7 days to evaluate a new isolation protocol using Liberase DH [49], as well as the ability of alginate to sustain follicle survival [48]. Isolated human primordial follicles were even frozen-thawed in alginate beads prior to in vitro culture, demonstrating that this polymer can also be applied to cryopreserve isolated follicles [50, 51]. However, Laronda et al. [33] did not achieve the same results and none of their isolated human primordial follicles survived culture. This was probably due to compromised follicle integrity after the isolation procedure [33] or their selected alginate concentrations (0.5 and 2%), which were different from those chosen by Amorim’s group [48–51].
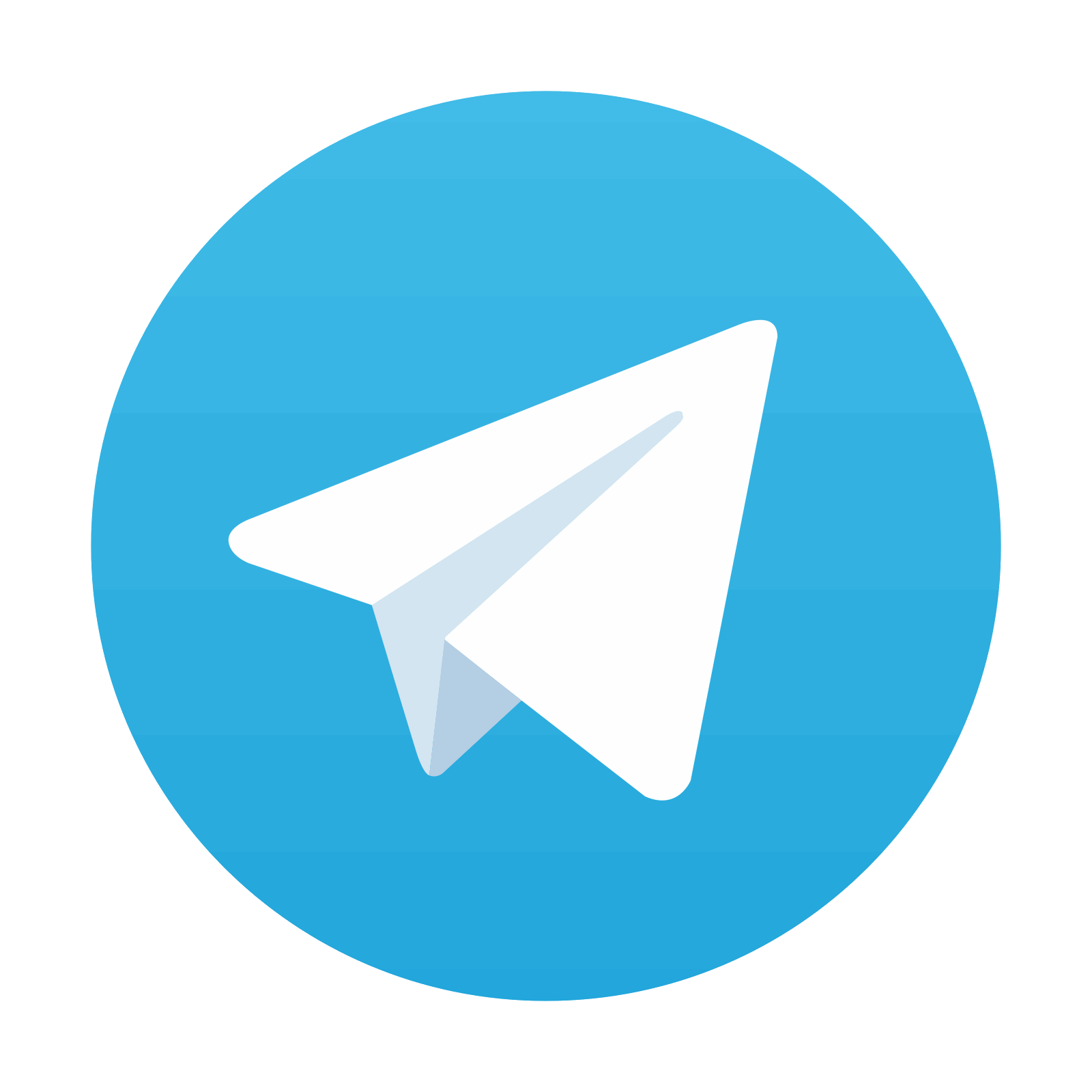
Stay updated, free articles. Join our Telegram channel

Full access? Get Clinical Tree
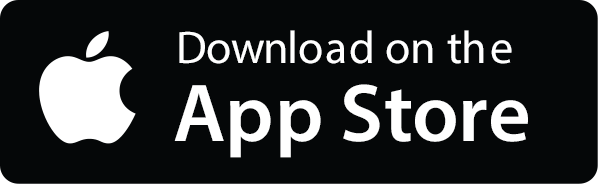
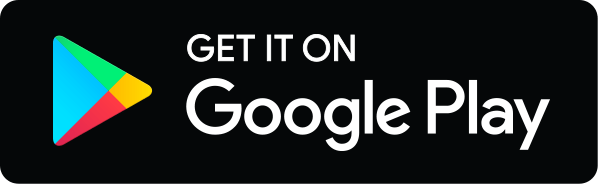
