Abstract
In vitro fertilization (IVF) outcomes are strongly correlated with the number of mature oocytes retrieved following controlled ovarian stimulation (COS) (). Cumulative live birth rates continuously increase with the number of oocytes available, irrespective of the patient’s age (). Multiple factors may influence the number of oocytes to be fertilized, such as the use of individualized stimulation protocols, patient compliance and the ovulation trigger (OT) strategy.
3.1 Introduction
In vitro fertilization (IVF) outcomes are strongly correlated with the number of mature oocytes retrieved following controlled ovarian stimulation (COS) (1). Cumulative live birth rates continuously increase with the number of oocytes available, irrespective of the patient’s age (2). Multiple factors may influence the number of oocytes to be fertilized, such as the use of individualized stimulation protocols, patient compliance, and the ovulation trigger (OT) strategy.
In natural cycles, the processes of follicle dominance and oocyte maturation rely on the shift of follicular FSH-dependency to LH-dependency, as the FSH levels fall along the end of the follicular phase leading to atresia of nondominant follicles (3). The FSH activity is responsible for nuclear maturation, induction of LH receptors in granulosa cells and maintenance of cumulus cell-to-cell communication. It also stimulates the conversion of plasminogen into plasmin protease that will provoke the rupture of the follicular wall before ovulation (4, 5). Conversely, the LH peak will initiate the ovulatory cascade by promoting the resumption of the meiosis in oocytes and luteinization of the granulosa cells, which will result in reduced estrogen levels and increased progesterone and prostaglandins secretion, essential for follicle rupture (6).
In stimulated cycles with exogenous gonadotropins, final oocyte maturation can only be achieved after artificial induction of a LH surge with GnRH agonist (GnRHa) or through the administration of human chorionic gonadotropin (hCG), which will act directly on LH receptors. In IVF cycles, ovulation is usually triggered when at least two follicles reach 17–18 mm in mean diameter, or the serum estradiol levels are compatible with 200–300 pg/mL per follicle in the final stage of development. Finally, oocyte retrieval is performed 34–36 h after OT.
A synchronous maturation of both the nucleus and the cytoplasm of the oocyte is crucial to yield top-quality embryos. Oocyte maturity is determined by the expansion of the cumulus mass, radiance of corona cells, the size and cohesiveness of granulosa cells as well as the shape and color of the oocyte (7). Nuclear maturation involves germinal vesicle breakdown, normally induced by the LH surge, followed by resumption of meiosis and, ultimately, extrusion of the first polar body (7).
A mature oocyte must be in the metaphase II stage (MII) of the second meiotic division. The final oocyte maturation is achieved after the resumption of the meiosis promoted by the hormonal surge of gonadotropins, either in natural or stimulated cycles (7). Furthermore, the ability of an oocyte to resume and complete meiosis is also related to the mean follicular diameter (8). Up to 30–40 percent of the oocytes are usually immature at the time of retrieval following COS, which reflects the varying size of the follicles at the time of the ovulation trigger (8). An oocyte retrieval can be considered optimal when more than 75 percent of the oocytes obtained are in the MII stage (9).
3.2 Triggering Ovulation in IVF Cycles
There are different OT strategies that may be used in IVF cycles. In order to reach the best maturity rate, an optimized OT strategy must be applied taking into account the type of ovarian stimulation protocol, the type of OT agent, and the timing of the oocyte pick-up after the trigger. Different scenarios deserve a personalized OT approach and clinical decision-making will vary according to the baseline characteristics of patients, response to COS, and embryo transfer schedule.
Theoretically, ovulation may be triggered with distinct agents, such as recombinant LH, kisspeptin, hCG, and GnRHa (10). However, in daily clinical practice only hCG and GnRHa are used. The current formulation of the recombinant LH (75 IU/vial) makes it unpractical to reach the 30,000 IU needed to induce ovulation (11). Regarding the kisspeptin, there is lack of consistent evidence (9, 10).
3.2.1 hCG Trigger
There is no specific receptor for hCG, but its pharmacological structure and biological similarity to LH, allow it to bind to the LH receptors (LHCG-R) and induce ovulation in gonadotropin-stimulated cycles (12). The first evidence on the existence of a LH/hCG receptor was demonstrated several decades ago, by in vitro studies using rat Leydig cells (13). These experiments found similar sets of binding sites and binding capacity for both hormones. Due to the additional 30 amino acids at its unique beta subunit, hCG binding has longer half-life, during approximately 7 days (14). Such a sustained luteotropic activity could lead to severe side effects through the release of vasoactive substances (e.g., vascular EGF) and prostaglandins (Figure 3.1) (10).
Figure 3.1 hCG trigger. Slow luteolysis offers an adequate luteal phase support.
There are two forms of hCG available: a) hCG extracted from the urine of pregnant woman or placental tissue, which is less pure; b) recombinant hCG produced with biomolecular technologies offering high purity and consistency. There are issues regarding the potency and dose equivalency of recombinant versus urinary hCG, but most studies indicate that 250 ug of the recombinant product is comparable with 5,000 to 10,000 UI of the urinary one (15). The most recent Cochrane review and meta-analysis failed to demonstrate any difference in reproductive outcomes and ovarian hyperstimulation syndrome (OHSS) incidence between the use of urinary and recombinant hCG for OT (15).
3.2.2 GnRHa Trigger
The use of GnRH agonist to induce an endogenous LH surge sufficient to trigger ovulation in IVF cycles was first described in 1990 (16). This strategy can only be applied in IVF cycles using a GnRH antagonist-induced downregulation or progestin priming, because of the competitiveness for the same receptor. Due to its greater affinity for the GnRH receptor than the endogenous GnRH, a bolus of agonist will promote a “flare-up” of gonadotropins, enough to induce final oocyte maturation with a much shorter duration than hCG (Figure 3.2) (17).
Figure 3.2 GnRHa trigger. Due to the quick luteolysis, additional drugs for luteal phase support are needed.
Despite the lower LH serum levels following the use of GnRHa, the concomitant release of FSH may add further physiological benefits and reduce adverse events such as OHSS (18). First reports using GnRHa revealed worst reproductive results most likely due to an insufficient luteal phase support after the “flare-up” period (19, 20). Adequate luteal phase support proved to improve outcomes (21). To date, neither large retrospective studies nor randomized clinical trials found significant differences between GnRH agonist trigger and hCG in terms of reproductive outcomes. Considering the faster drop in gonadotropins concentration along with desensitization and blockage of the receptor, appropriate luteal phase support must be employed when GnRHa is used to trigger ovulation (21). Otherwise, it will result in reduced implantation rates and increased miscarriage rates compared to hCG (22).
3.2.3 Dual Trigger
The “dual trigger” combines the benefits of the hCG and GnRHa to trigger ovulation. Theoretically, a low dose of hCG would be sufficient to avoid a suboptimal response to GnRHa trigger while maintaining a more effective luteal phase support without increasing the risk of OHSS (Figure 3.3) (23). In the last decades it has been used in distinct scenarios such as hyperresponders, poor response to COS, empty follicle syndrome or high proportion of immature oocytes in previous cycles, and also in normal responders (9, 24).
Figure 3.3 Dual trigger (GnRHa + hCG at the same time). Combines the advantage of reducing the risk of OHSS while maintaining an adequate luteal phase support and diminishing the incidence of EFS.
A recent systematic review showed that the dual trigger strategy is at least equivalent to GnRH or hCG alone in terms of number of oocytes retrieved, implantation rate and pregnancy rate in normal or hyperresponders (25). Unfortunately, reliable data on the incidence of OHSS with the dual trigger strategy in hyperresponders is lacking, even though most studies reported reduced rates. Concerning the poor responders or those with a high proportion of immature oocytes in previous cycles, the dual trigger seems to ameliorate the number of oocytes obtained, implantation, and pregnancy rates (25). However, there is no consensus on the recommended dose of hCG in the dual trigger approach.
3.2.4 Double Trigger
The double trigger consists of the administration of GnRHa and hCG at 40 h and 34 h before oocyte pick-up, respectively (Figure 3.4) (26, 27). It has been suggested to improve the proportion of mature oocytes retrieved and to overcome some cases of empty follicle syndrome (EFS) (26, 28). Besides prolonging the interval between OT and oocyte retrieval, it offers the advantage of the hCG for luteal phase support. Nevertheless, there is insufficient data concerning the incidence of OHSS and premature ovulation with the double trigger.
Figure 3.4 Double trigger (GnRHa at 40 hours before OPU + hCG at 34 hours before OPU). Follows the same principle as in the dual trigger strategy.
A pilot study on granulosa cells gene expression showed that the double trigger strategy increased the expression of epiregulin and amphiregulin compared to hCG alone to trigger ovulation (29). A previous study demonstrated that the supplementation of the maturation medium with amphiregulin and epiregulin enhanced the maturation rate of human oocytes in vitro (30), which may explain the improved maturation, fertilization, and pregnancy rates among women that received the double trigger in that pilot study (29). Further basic studies may add important knowledge in the underlying mechanisms involved in human oocyte maturation.
3.3 Suboptimal Response to GnRHa Trigger
The resumption of the meiosis in human oocytes occurs 14–18 hours after the onset of the LH surge (6), but the time interval since the onset of the LH surge and the threshold level of LH needed to induce follicular rupture are higher than those to induce oocyte maturation (31). Typically, LH levels greater than 15 IU/L continuing for 14–18 h is required for expanding cumulus cells that lead to ovulation trigger (32).
Low LH levels on the day of the OT is a risk factor for a suboptimal response to GnRHa. A recent study showed that LH levels inferior to 52 IU/L at 12 h after a bolus of GnRHa is suboptimal, while LH levels under 12 IU/L caused a dramatic reduction in oocyte yield and maturity (23). Serum LH levels greater than 15 IU/L at 12 h post-GnRHa trigger has been associated with significantly higher number of oocytes retrieved and increased oocyte maturity rate (23, 33). Furthermore, the lower the LH level after GnRHa trigger, the higher the suboptimal response rate (32). Indeed, LH levels inferior to 0.1 IU/L at the day of final oocyte maturation exhibit a suboptimal response rate nearly to 25 percent (32). However, regarding oocyte fertilization rate and clinical outcomes, a study failed to demonstrate statistically significance differences, as for the number of high-quality embryos and embryos transferred (33). An easier, cheaper and more convenient way to confirm that LH surge did happen after GnRHa trigger would be a urine LH test the morning after the trigger. We had shown that this is a very good alternative to blood sampling, avoiding patients coming again to the clinic, and with an excellent sensitivity (34).
Additional risk factors for suboptimal response to GnRHa trigger have been identified, such as very low basal FSH and LH levels at the start of stimulation and long-term hormonal contraception (32). Situations that lead to hypothalamic-pituitary axis suppression or dysfunction are believed to increase the risk of suboptimal response to GnRHa trigger, and the use of double trigger or dual trigger strategies may improve reproductive outcomes in these cases (32, 35).
In natural ovulatory cycles, the FSH preovulatory peak promotes the expression of LH receptors in granulosa cells and supports nuclear maturation along with cumulus development and expansion (4). Rosen et al. (36) reported significantly higher intrafollicular FSH concentration in follicles containing oocytes. Similarly, Lamb et al. (37), described a statistically significant improvement in oocyte recovery rate when concomitant FSH/hCG trigger injection was used compared to conventional hCG trigger alone. Nevertheless, whether an additional FSH bolus administered at the time of trigger enhances IVF outcomes requires further investigation.
3.4 Empty Follicle Syndrome
The empty follicle syndrome is a rare condition of uncertain etiology in which no oocytes are retrieved after technically correct oocyte pick-up (OPU) procedure, from apparently normally growing follicles during COS for assisted reproductive techniques (38). Although the exact etiology is not fully understood, a deficiency on loosening the cumulus oocyte complex from the follicles wall may play a role (38).
There are two types of EFS differentiated by the hormone levels on the day of OPU. The so-called genuine empty follicle syndrome happens when serum levels of hCG or LH are concordant with the correct administration of the triggering drug, while the false empty follicle syndrome (FEFS) occurs when such hormones levels are remarkably low (39).
The prevalence of EFS has been estimated to be around 2–7 percent (40). Most cases seem to be FEFS, which is commonly ascribed to a patient error in timing, preparation, or administration of the triggering drug, as well as to problems with manufacturing or shelf life (39). Abnormalities in the in vivo biological activity of some batches of commercially available hCG or GnRH and a rapid clearance of the hCG by the liver have also been described (41).
When problems in drug administration or quality were properly excluded, several hypotheses arise: early oocyte atresia due to a dysfunctional folliculogenesis in the presence of an apparently normal hormonal response; biological abnormality in oocyte supply despite normal bioavailability of hCG; and genetic factors such as LH/hCG receptor mutation or a blockage in the LH pathway (39, 40). Moreover, ovarian aging is considered a risk factor for EFS recurrence, probably due to altered folliculogenesis (42). In cases of GnRHa receptor polymorphism, higher doses of agonist are probably necessary in order to activate the receptor (39).
Finally, patients suffering from temporary or permanent hypothalamic-pituitary dysfunction should not receive GnRH agonist trigger, because a sufficient flare-up will not be achieved which will lead to a deficiency in follicular maturation and EFS (38). Borderline patients presenting with low circulating levels of both LH and FSH would also be at risk of developing EFS after GnRHa trigger (40).
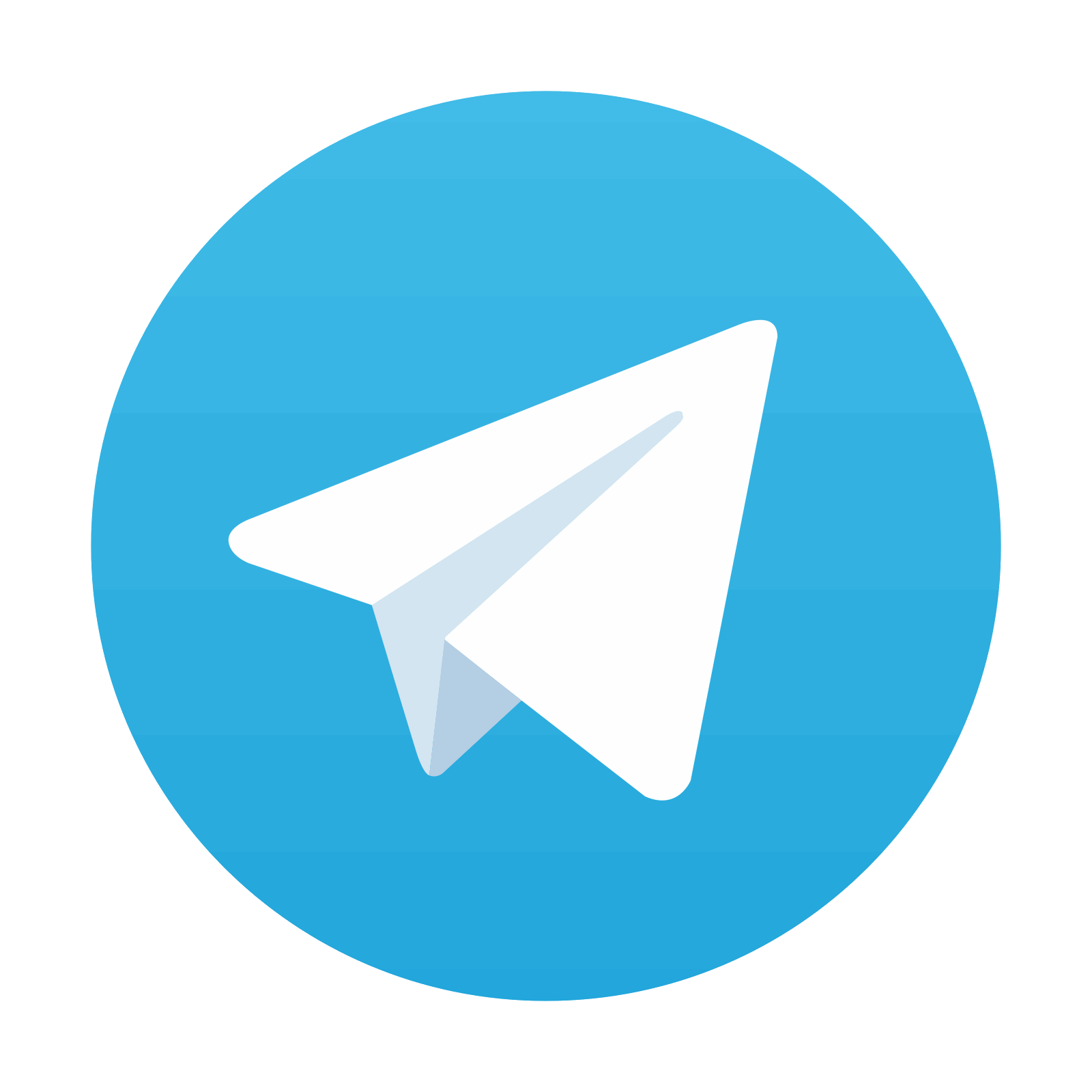
Stay updated, free articles. Join our Telegram channel

Full access? Get Clinical Tree
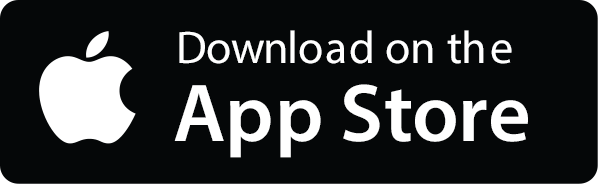
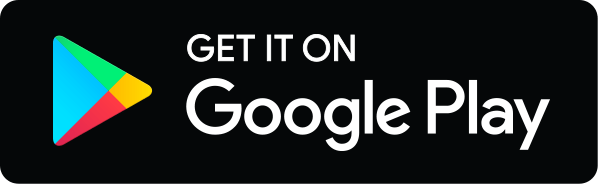