Abstract
Patients with cancer who desire to preserve their future reproductive potential but require immediate gonadotoxic treatments (chemo and/or radiotherapy) are left with few options for fertility preservation. These options include (a) cryopreservation of ovarian tissues as cortical strips; (b) dual cryopreservation of both ovarian cortical tissue and cryopreservation, after in vitro maturation, of immature oocytes extracted from the small antral follicles visible within the ovarian cortex at the time of the harvest; and (c) cryopreservation of one whole ovary [1–9]. At the time of this writing, each of these options is still considered experimental by the American Society of Reproductive Medicine (thus requiring institutional review board approval and patient’s informed consent), although it is expected that soon ovarian tissue cryopreservation will no longer be considered experimental.
Introduction
The field of female fertility preservation is based on the ability to successfully cryopreserve ovarian tissue [1], and this can now be offered to a wide range of patients worldwide [2, 3]. Fragments of cryopreserved ovarian cortex can be thawed and autografted to an orthotopic or heterotopic site to restore fertility [4, 5]. The potential of this tissue to restore fertility would be greatly enhanced if immature oocytes contained within the tissue could be grown to mature stages within the laboratory and this would be particularly beneficial for prepubertal girls who currently have fewer options to preserve and restore their fertility than adult women [2, 3].
Cryopreserved tissue contains the most immature stage of oocyte within primordial follicles and the main aim of culturing this tissue is to support in vitro gametogenesis/growth (IVG) to develop immature oocytes entirely in vitro [6]. If this methodology could be demonstrated to be safe, it would maximize the potential of cryopreserved ovarian tissue and have many clinical applications [2, 3]. Complete oocyte development in vitro from the primordial stage has been achieved in mice [7, 8], but the larger size and longer growth period of human follicles has made the inter-species translation of these techniques difficult [6, 9]. Good progress has been made in defining conditions that support different stages of human follicle development in vitro, and these advances bring the prospect of achieving a complete in vitro system that supports oocytes from primordial to maturation closer [10, 11].
This chapter deals with our current understanding of in vitro development of human oocytes and highlights the areas that need to be optimized before a complete in vitro growth (IVG) system for human oocytes can be realized.
Follicle Development
In most mammals oocytes are formed prenatally or shortly after birth. The earliest stage of oocyte development is the primordial oocyte which is held within the primordial follicle [12] (Figure 29.1). Primordial follicles form the population of germ cells from which recruitment for growth will take place throughout the female’s reproductive life [6, 10]. The primordial follicle consists of an oocyte arrested at the dictyate stage of Prophase 1 of meiosis enclosed within flattened somatic (granulosa) cells (Figure 29.1). Primordial follicles represent a resting pool of germ cells that are utilized throughout life until depletion. The regulation of growth and development of primordial follicles is under stage-dependent paracrine and endocrine control [13] modulated by oocyte–somatic cell interactions [14] and microRNAs [15]. In addition to recognized control mechanisms, it is now known that biomechanical forces are important in regulating follicle initiation and growth [16]. Follicle development represents a dynamic process that can be broken down into a series of transition stages: (1) initiation/activation of primordial follicle growth; (2) growth of the activated primordial stage to form multi-laminar pre-antral follicles; (3) morpho-genetic transition of the multi-laminar follicle to form a fluid filled cavity (antrum) with concomitant differentiation of granulosa cells to mural and cumulus cells; (4) a species specific enlargement phase of the antral follicle to the pre-ovulatory or Graafian stage, associated with granulosa cell proliferation and antral fluid accumulation within the basement membrane; and (5) rupture of the Graafian follicle in response to the mid-cycle luteinizing hormone (LH) surge to release the oocyte–cumulus complex at ovulation (Figure 29.1) [6].
Figure 29.1 Diagrammatic representation of follicle stages of development. Primordial follicles represent a pool of nongrowing follicles which are continually initiated to grow throughout reproductive life. Once follicles are activated to grow (primary stage) granulosa cells proliferate to form multi-laminar structures (pre-antral) and then form a fluid filled cavity (early antral) which undergoes expansion (mid-antral) to reach pre-ovulatory stages. During follicular development there is coordinated oocyte growth. Sizes shown illustrate oocyte development
Primordial follicles represent the most abundant stage of development present within the ovary at all ages. During growth and development the oocyte must acquire competence to resume meiosis as well as the capacity to support fertilization and embryonic development (developmental competence) [17]. The fate of 99.9 % of oocytes in vitro is degeneration therefore only 0.1% of the population of oocytes may ever achieve this potential in vivo [18]. Creating conditions in vitro that support the growth and maturation of immature oocytes requires knowledge of the physiological requirements of the oocyte, and each of the somatic cells within the ovary and how they interact [10]. These requirements are dynamic and complex and each follicle/oocyte represents a unique environment dependent upon the quality of somatic cell-oocyte interactions and paracrine/endocrine signaling [19, 20]. In addition, DNA integrity and stability of the oocyte will be affected during development in vivo and this will differ with age [21]. Therefore, the development of culture systems needs to be on the background of a robust understanding of how conditions affect DNA integrity and stability of oocytes [21, 22]. Recapitulating such a complex multi-layered process in vitro represents an enormous technical challenge.
Developing Follicles in vitro
The ability to obtain mature oocytes after IVG of primordial follicles would be particularly beneficial for prepubertal girls undergoing fertility preservation prior to being exposed to damaging chemotherapy [2, 3]. Cryopreservation of ovarian cortical tissue with the potential for subsequent re-implantation is currently the only fertility preservation option available to young girls [2, 3]. In some cases re-implantation of tissue would not be possible so IVG would expand the options available. Given that cryopreserved ovarian material contains predominantly primordial follicles, this would be the most appropriate starting point for a culture system.
Complete in vitro development from primordial stages to fully grown oocytes capable of being in vitro matured (IVM) to resume meiosis and subsequently fertilized in vitro to form embryos that could be successfully transferred has been achieved in the mouse [7, 8]. John Eppig’s group developed a two-step culture system in which primordial follicles activated growth within pieces of ovary from new born mice in step one [7]. After step one oocyte–granulosa cell complexes (OGCs) could be removed enzymatically and after being grown on membranes in step two, mature oocytes were obtained [7, 8]. The initial study demonstrated that complete oocyte development was possible in vitro but only one mouse derived from IVG oocytes was obtained [7]. This result was encouraging but the single mouse obtained from these experiments developed many abnormalities as an adult, although it is not clear if these can be directly attributed to culture conditions [7]. Further development of the culture system led to improvements in the composition of culture medium and the physical environment [8]. These changes resulted in a more robust culture system that could produce several embryos and offspring from oocytes that had been in vitro grown then combined with in vitro maturation (IVM) and in vitro fertilization (IVF) [8]. To date these are the only reports that have demonstrated complete oocyte development from the primordial stage in vitro using a rodent model, this indicates the degree of complexity and technical challenges involved in developing multistep culture systems [7, 8]. These have formed the basis of systems that support complete development of mouse oocytes from stages prior to primordial follicle formation [23, 24]. Developmentally competent oocytes capable of being fertilized and forming embryos have been produced from induced pluripotent stem cells [23] and primordial germ cells [24]. Rodent models have provided proof of principle that complete oocyte development can occur in vitro but the difficulties involved have been emphasized. It is clear that translating these systems to support human oocyte development represents a huge challenge [9].
Human Oocyte Development In Vitro
There are several reports of culture systems that support specific stages of human oocyte development but to date there is only one that reports development of human oocytes from the primordial stage to maturity within a multistep culture system (Figure 29.2) [11]. While this multistep system is not optimal it highlights the sequence of environments that may mimic the conditions required to support human oocyte development in vitro [10, 11]. A multistep culture system is required to achieve complete in vitro development of human oocytes. The follicle functions both as an endocrine structure and as a vehicle to support oocyte growth and development, but in a culture system the focus should be on oocyte development. By focusing on oocyte development and the maintenance of appropriately differentiated somatic cells in contact with the oocyte may avoid the need to maintain large follicular structures in vitro. The multistep approach has been designed to support the changing requirements of the developing oocyte and its surrounding somatic (granulosa) cells and is broken down into three major stages: (1) culturing small pieces of ovarian cortex to support activation of primordial follicles (2) isolation and culture of growing pre-antral follicles to achieve oocyte growth and development to antral stages and (3) aspiration and maturation of oocyte cumulus complexes (Figure 29.2).
Figure 29.2 A multistep culture system to support in vitro growth of oocytes from human primordial follicles through to maturation [11]. Step 1: Flattened strips of ovarian tissue (a) are cultured free floating in medium containing human serum albumin, ascorbic acid and basal levels of follicle stimulating hormone [11]. Once follicles have reached multi-laminar stages they are isolated mechanically using needles, Step 2: Isolated follicles are cultured individually (b) from pre-antral to antral stages (c). Step 3: The final stages of oocyte growth and development are achieved by removing the oocyte–cumulus complex from the antral follicle (d) and culturing the oocyte and its surrounding somatic cells (e). Step 4, oocyte–cumulus complexes placed within medium for in vitro maturation. Oocytes are then analyzed for the presence of a Metaphase II spindle and a polar body. Ultimate goal is to determine whether these complexes can be fertilized and form embryos, but this remains to be determined
Initiating Primordial Follicle Growth In Vitro
Isolated primordial follicles do not activate growth in vitro; therefore, to achieve activation, primordial follicles need to be maintained within small pieces of ovarian cortex containing stromal cells [11, 25–30]. Several culture systems that support the initiation/activation of human primordial follicles have been developed [11, 25–33]. Each of these systems results in activation and growth of primordial follicles but at varying rates which seems to be related to the preparation and architecture of the starting tissue, which indicates the importance of the component cell types and mechanical signaling to cell pathways regulating primordial follicle activation [16].
Understanding how the “resting” population of primordial follicles is regulated is key to developing IVG systems; however, in the human ovary regulatory mechanisms are ill defined [9, 34]. The phosphatidylinositol-3’-kinase (PI3 K-AKT) signaling pathway within the oocyte has been shown to be a key regulator of primordial follicle activation in human [34–37]. The phosphatase and tensin homolog deleted on chromosome ten (PTEN) acts as a negative regulator of this pathway and suppresses initiation of follicle development [38]. Other components of this pathway are dependent on the mammalian target of rapamycin complex 1 (mTORC1), a serine/threonine kinase that regulates cell growth and proliferation in response to growth factors and nutrients and also regulates primordial follicle activation [39]. From knockout mouse data it appears that while PTEN within the oocyte suppresses activation of primordial follicles mTORC promotes it.
Preparing ovarian cortical tissue into micro-cortex fragments for culture results in activation of primordial follicles related to disruption of Hippo signaling [13, 35, 40]. The Hippo signaling pathway controls organ size through regulating cell proliferation and cell death [41]. Fragmentation affects these processes and the role of the Hippo signaling pathway explains differences observed between culture systems where ovarian cortex prepared as dense cubes in vitro results in low levels of follicle activation [26, 27, 30], while significant activation occurs in human ovarian cortex prepared as micro-cortex which has underlying stroma removed and tissue interactions disrupted [11, 29]. In addition to the effect of Hippo signaling, manipulation of the PI3 K pathway results in further activation of follicles [13, 35–37, 40, 42]. The action of PTEN can be inhibited pharmacologically using compounds such as bisperoxovanadium [43]. Increased activation of primordial follicles and increased numbers of secondary follicles can be obtained when a PTEN inhibitor is added to the culture medium for 24 hours [37], but subsequent growth and survival of isolated secondary follicles is compromised [35, 37]. The deleterious effect of PTEN inhibition on isolated secondary follicles may be a consequence of increased DNA damage and reduced repair responses within the oocyte/follicle. It has been demonstrated using cultured bovine ovarian cortex that inhibition of PTEN leads to increased activation of primordial follicle but results in high levels of DNA damage and reduced capacity of DNA repair mechanisms [42]. It is now recognized that oocytes have a good capacity for efficient DNA repair and it is not known how altering this capacity influences final oocyte quality [44].
Once follicle growth has been initiated within cortical tissue they develop to multi-laminar stages but do not survive well within the cortical environment (Figure 29.2). When follicles reach multi-laminar stages the cortical environment becomes inhibitory so individual follicles should be isolated and cultured individually to support further development [11, 29, 31, 45].
Culturing Isolated Growing Follicles
Multi-laminar stages can be removed from the ovarian cortex using enzymes, mechanical isolation or a combination of both. A combination of collagenase and DNase can be used to remove pre-antral follicles from stromal tissue; however, this combination can cause damage which results in poor survival of growing follicles [46]. Highly purified enzyme preparations such as Liberase may reduce the damage that occurs with collagenase [47, 48]. Isolated follicles need theca layers to retain their structure and survive the second stage of IVG and these may be compromised by enzymatic preparations [46]. To avoid the deleterious effects of enzymes, follicles can be isolated mechanically using needles to remove them from the stroma. Mechanical isolation of follicles has the advantage of preserving follicular integrity by maintaining the basal lamina and thecal layers; however, the procedure is laborious and results in a low yield [11, 46].
The challenge of growing multi-laminar structures that could grow up to several millimeters in diameter has led to the application of tissue engineering to develop systems that support growth of isolated follicles [49–51]. Bio-matrices such as Alginate have been used to encapsulate human pre-antral follicles and support their structure and growth in vitro [52]. Encapsulation with alginate mimics the extra cellular matrix in vivo in terms of its ability to facilitate molecular exchange between the follicle and the culture medium while its flexibility accommodates cell proliferation but its rigidity prevents dissociation of the follicle unit [53]. The degree of rigidity of the alginate capsule affects follicle development. Reduction in steroidogenesis and growth have been reported in murine follicles encapsulated in 1% alginate gels [54], whereas fully grown human oocytes have been produced using 0.5% gels [52]. These studies demonstrate that the physical environment of the ovary is a regulating force and that biomechanical signals can be mimicked by an appropriate support matrix [55].
Scaffolds such as de-cellularized ovarian tissue [56] and 3D microporous scaffolds are being developed as matrices to support human pre-antral follicle growth [57]. The production of electrospun patterned porous scaffolds to support follicle development is being explored and these may be more accessible and reproducible than de-cellularized tissue [58]. Engineered scaffolds clearly have great potential and need to be further developed.
No matrices or scaffolds are used to support the growth of isolated pre-antral follicles during the multistep culture system that has been developed for human follicles [11]. Follicular architecture is maintained by growing follicles in V-shaped micro-well plates and this supports growth in vitro while promoting differentiation and antral formation during a relatively short culture period of up to 10 days [11, 29].
Follicles isolated from ovarian cortex and grown in vitro undergo significant growth and development. Secondary human follicles isolated enzymatically from fresh ovarian tissue and cultured in the presence of follicle stimulating hormone (FSH) become steroidogenically active and complete oocyte growth within 30 days [52] and these oocytes are capable of meiotic maturation [59]. Primordial follicles grown within fragments of ovarian cortex to multi-laminar stages, isolated mechanically and cultured individually in the presence of Activin and FSH form antral cavities and become steroidogenic within 10 days of IVG (Figure 29.3A and B) [11, 29].
Figure 29.3 Photomicrographs of in vitro grown human ovarian follicles: A. In vitro grown antral follicles after Step 2 of culture [11]. B. Histological section of in vitro grown antral follicle highlighting the oocyte cumulus cell complex that can be removed after a total of 16–20 days in culture [11]. C. In vitro grown oocyte–cumulus cell complex isolated and cultured on a membrane for further growth (step 3), then placed in maturation medium for 24 hours. D. IVG complex after maturation and formation of a polar body, indicative of progression to metaphase II; this is confirmed in E where the metaphase II spindle of the oocyte is immuno-stained
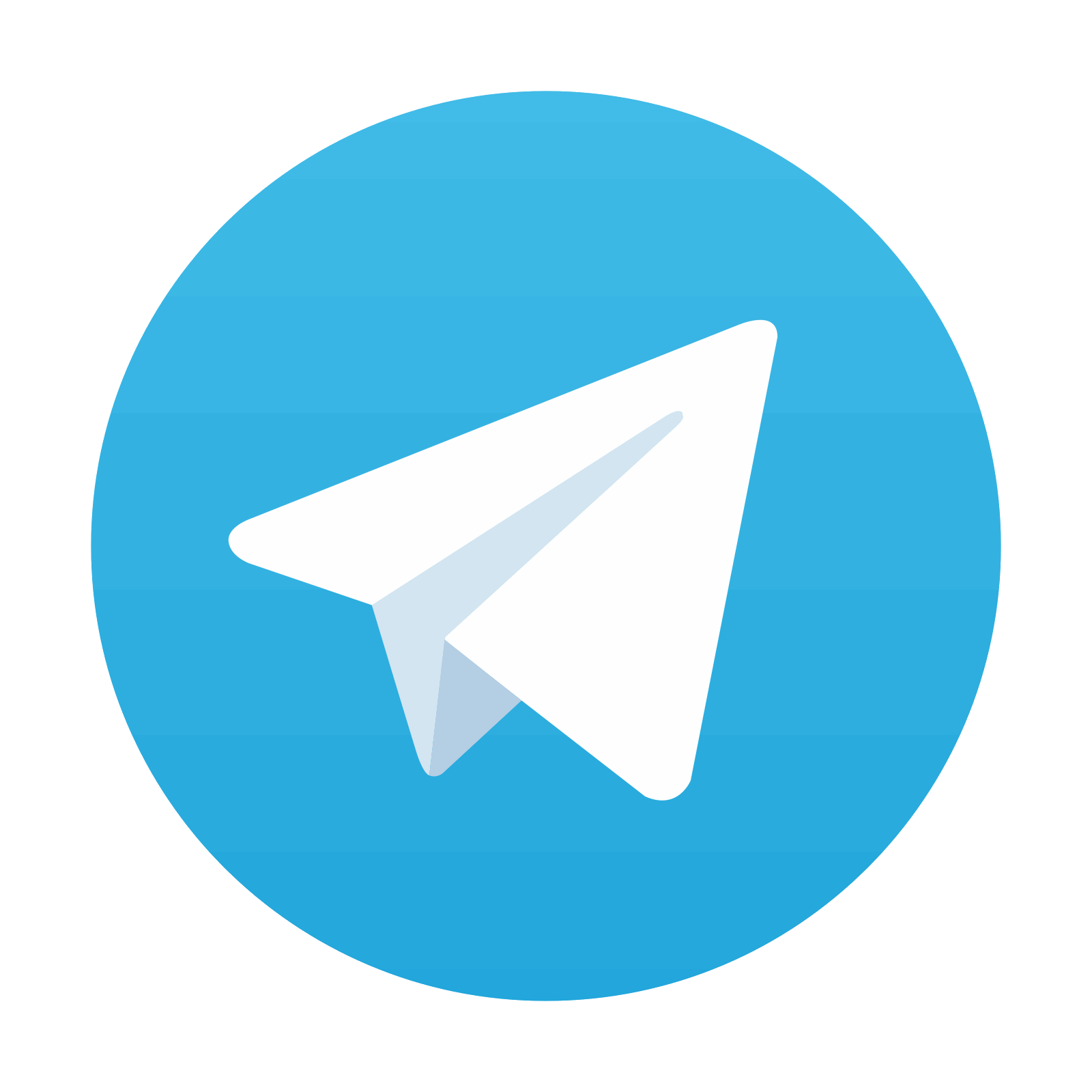
Stay updated, free articles. Join our Telegram channel

Full access? Get Clinical Tree
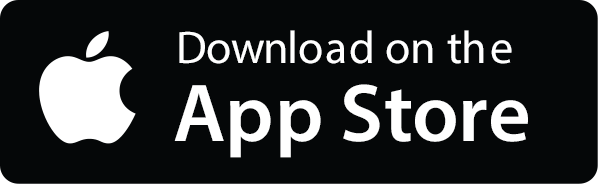
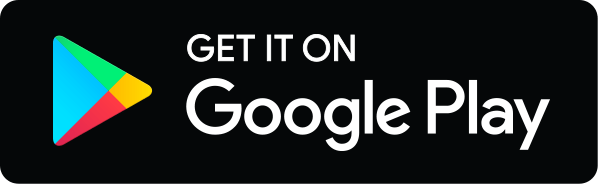
