Abstract
Early detection and aggressive chemotherapy/radiotherapy treatments have improved the long-term survival rates for many young women with various types of cancer. As a consequence of these cytotoxic treatments, their reproductive future can be either short lived or eradicated. For young single women with cancer, oocyte cryopreservation offers the best potential option for achieving a future pregnancy using their own gametes. Unfortunately, the urgent need to commence cytotoxic treatment often does not permit adequate time for cryopreservation of mature oocytes. Conversely, cryopreservation of ovarian tissue eliminates the delay necessary to obtain mature oocytes, but the subsequent potential for establishing pregnancy is currently unknown. Although ovarian tissue cryopreservation is an attractive alternative and frequently used for patients with these conditions, little has been published on the efficacy of various protocols. Cryopreservation of ovarian tissue is more complex than that of gametes or embryos, requiring preservation of multiple cell types, which may vary in volume and water permeability.
Introduction
Patients with cancer who desire to preserve their future reproductive potential but require immediate gonadotoxic treatments (chemo and/or radiotherapy) are left with few options for fertility preservation. These options include (a) cryopreservation of ovarian tissues as cortical strips; (b) dual cryopreservation of both ovarian cortical tissue and cryopreservation, after in vitro maturation, of immature oocytes extracted from the small antral follicles visible within the ovarian cortex at the time of the harvest; and (c) cryopreservation of one whole ovary [1–9]. At the time of this writing, each of these options is still considered experimental by the American Society of Reproductive Medicine (thus requiring institutional review board approval and patient’s informed consent), although it is expected that soon ovarian tissue cryopreservation will no longer be considered experimental.
Ovarian tissue cryopreservation and transplantation [10–11], mostly as orthotopic allografts, has shown reproductive success [12–14] and hundreds of live births from re-transplantation of ovarian cortical tissue have been reported [15–21]. Typically, after re-transplantation it takes about 4–5 months for resumption of endocrine function as evidenced by menses or serological hormonal evaluation. A massive recruitment of primordial follicles after the re-transplant is followed by minimal follicular recruitment to regulate the ovarian reserve [22]. Although the life span of re-transplanted ovarian tissue varies, reports of continuation of function of re-transplanted frozen/thawed ovarian cortical strip for more than 5 years and birth of more than one healthy baby following single grafting provided hope not only for saving the fertility but also for restoration of a woman’s natural endocrine function in a long term expanding of reproductive lifespan [21].
Jensen et al. [23, 24] analyzed outcomes of transplantations of cryopreserved ovarian tissue to 41 women in Denmark from 2003 to 2014 and also the outcome of 86 successful births. They reported the functional life span of the grafts >10 years for two patients, >7 years in another three patients, >4 years in another seven patients, between 2 and 4 years in 15 patients, between 1 and 2 years in seven patients, and less than a year in four patients. Most large follicles in the graft are lost during freezing and thawing. In addition, the follicular density in individual pieces of cortex may vary considerably, making it difficult to know how many follicles are actually present in the grafted pieces.
Several factors can affect ovarian graft longevity, including: (1) ovarian reserve; (2) chemotherapy before cryopreservation; (3) graft size and method of ovarian tissue preparation; (4) nonhomogeneous distribution of follicles in grafted cortical pieces; and (5) angiogenic potential of the graft site influencing the degree of ischemia after transplantation. Silber et al. [22] proposed that careful preparation of the donor tissue as a very thin layer, avoidance of compressing hematoma between the ovarian vascular graft bed, and closely opposing the graft are favorable conditions to promote a more rapid revascularization. By the time neovascularization occurs, the grafts will have already sustained significant ischemic damage resulting in massive loss of primordial follicles [25–28], ultimately responsible for the limited functional life span of the graft [27–28]. This process was illustrated in a sheep model that found that 60–70% of follicles were lost at transplantation, but only 7% of the loss was dependent upon the cyropreservation procedure itself [27].
Cryopreservation of the whole ovary with its intact pedicle and vascular supply has been proposed as an experimental strategy that could potentially overcome the problem of ischemic damage; in these instances, a successful re-anastomosis will provide immediate reperfusion so, theoretically, the ovary should maintain long-term endocrine and reproductive functions [2, 29–37].
This chapter summarizes the technical challenges that had to be overcome for the freezing/thawing of large organs as intact ovaries with animal experiments first and then with humans.
Challenges of Whole Ovary Cryopreservation
Two main problems with whole organ cryopreservation and re-transplantation have caused technical difficulties: the first related to the feasibility of executing a perfect vascular re-anastomosis of the whole organ with re-establishment of a prompt vascular flow; the second was related to the development of a successful cryopreservation protocol.
Experiments with whole fresh ovary re-transplants have proved that the re-anastomosis of the ovarian pedicle is technically possible in rats [31], rabbits [38], sheep [34, 39–41], dogs [42], monkeys [43] and in human [44–47]. Wang et al. demonstrated that cryopreservation and subsequent re-transplantation of the reproductive system en bloc could be performed in rats [37]. To date, two cases of human re-transplant of fresh whole ovaries and one live birth have been reported [47]; however, no cases of frozen/thawed whole ovary re-transplants have been performed yet.
The second challenge, that is, creating a successful cryopreservation protocol for large-sized intact ovaries has proved more problematic due to (a) heat and mass transfer problems; (b) the physical constraints related to the heat transfer between the core and periphery of a large organ; and (c) the establishment of adequate stromal and cortical diffusion of the cryoprotectants [48, 49] to prevent the formation of intravascular ice. Large amounts of ice crystals are a major destructive force for cells and are known to be mechanically disruptive. Inadequate perfusion of the cryoprotectants into the vascular compartment may lead to intravascular ice formation with subsequent vascular injury and irreversible endothelial disruption [50–53]. Slow cooling methods were developed to allow a slow enough process to dehydrate cells to prevent intracellular crystallization but, at the same time, fast enough to minimize osmotic stress to cells. However, as described later, the slow cooling method is not optimal for the cryopreservation of whole human ovaries.
Whole Ovary Freezing in Rat Model
Wang et al. investigated cryopreservation after immersion in liquid nitrogen and re-transplantation with microscopic re-anastomosis of whole rat ovaries [37]. In this landmark study, the authors used adult female rats and removed the right ovary and the upper segment of the uterus en bloc with the ovarian vessels dissected to create short cuffs of aorta and vena cava. Seven dissections were perfused for 30 min at 0.35 ml/min with M2 medium containing 0.1 M fructose and increasing concentrations of dimethyl sulfoxide (DMSO). The treated organs were then cooled slowly in Cryovials and, after overnight storage in liquid nitrogen, were rapidly thawed and the cryoprotectant was removed.
After re-transplantation, four of the seven rats had subsequent follicular development, with corpora lutea indicating recent ovulation, and one animal achieved pregnancy. Importantly, tubal and uterine morphology and architecture were indistinguishable from non-operated controls. However, these rats had higher serum follicle stimulating hormone (FSH) levels, fewer follicles and lower estradiol levels and uterine weights than controls, indicating that the freezing had compromised the ovarian function.
Yin et al. continued this work using a similar model to investigate the long-term longevity of ovarian grafts, as well as to assess the effect of ischemia after cryopreservation for 24 hours prior to transplant [51]. Graft survival with endocrine function was seen at 2 months, and ovulatory response to FSH was seen at 4 months, suggesting good graft survival after vascular anastomosis. These grafts, however, had fewer surviving follicles than the controls, emphasizing that even minimal ischemia time significantly reduces the follicular pool.
Whole Ovary Freezing in Sheep Model
To improve the technique of freeze-thaw and transplantation of whole ovaries, adult female sheep have become the preferred animal model to study both slow cooling and vitrification methods. Sheep have ovaries that are similar in size to humans and are therefore ideal animal models. Despite human ovaries having different vascular pedicle anatomy, the sheep ovaries have dense fibrous stroma and a relatively high primordial follicles density in the cortex like human ovaries [52].
Slow Cooling
In 2002, Jeremias et al. attempted orthotopic transplantation of a whole ovary in an adult sheep by anastomosis of the vascular pedicle [40]. After bilateral laparoscopic oophorectomy, ovaries were autotransplanted into the abdominal wall, and microsurgical vascular anastomosis of the ovarian to the inferior epigastric vessels was performed. After noting promising resumption of endocrine function post-transplant and a high follicular count, the authors concluded that, in conjunction with an improved protocol for cryopreservation, “ovarian auto-transplantation with vascular anastomosis may be superior to ovarian tissue banking and grafting techniques” [40].
The same team also performed one of the first transplants of an intact frozen-thawed ovary via microvascular anastomosis [48]. After laparoscopic dissection, the whole ovaries were immediately perfused with heparin, followed by perfusion and immersion in a bath containing Leibovitz L-15 medium, 10% fetal bovine serum (FBS) and 1.5 M DMSO.
Ovaries were perfused via the ovarian artery with the cryoprotectant solution at a rate of 1.3 ml/min. After perfusion, the ovaries were transferred into a Cryovial and cooling began at 4°C and at 2°C/min until ice nucleation was induced at –7°C. The temperature was then reduced by 2°C/min until –35°C and, subsequently, by 25°C/min until –140°C after which the Cryovials were plunged into liquid nitrogen. Thawing occurred 1 week later, and was achieved by first plunging and swirling Cryovials in water bath at 37°C. The ovaries were then immediately perfused with Leibovitz L-15 and 10% FBS for 20 min, thus gradually eliminating the cryoprotectant. After microvascular transplantation immediate patency was documented in 100% of the grafts. However, after 8–10 days, 77% of the ovaries showed complete occlusion of the anastomosis. While no significant differences were found in the mean values of apoptosis and follicular viability compared to ovarian cortical strip cryopreservation and autotransplantation by TUNEL assay and histology, postoperative FSH levels were much lower in the whole ovary graft group (P = 0.03) and like preoperative values in animals with patent vessels [48]. After establishing long-term patency of the anastomosis, the next objective was to assess whether the post-transplanted ovaries could respond to in vivo stimulation with FSH and produce viable oocytes. Grazul-Bilska et al. treated ewes for approximately 5 months post-transplant with FSH and then the ovaries were removed [53]. In all ovaries, primordial, primary, secondary, antral and pre-ovulatory follicles were found along with fully functional vascularization, which was manifested by the expression of factor VIII, vascular endothelial growth factor (VEGF) and smooth muscle cell actin (SMCA). Proliferating cells were detected in follicles, and the rate of apoptosis was minimal. One ewe had four visible follicles from which three oocytes were collected, but none fertilized. The morphology of autotransplanted and control ovaries was similar. Using both in vitro and in vivo methods, Arav et al. showed no significant difference in follicular survival between fresh ovaries and frozen-thawed ovaries, as well as similar histological morphology and normal immunohistochemical expression of factor VIII, suggesting normally restored vascular pedicles [54]. In addition, six oocytes were aspirated from two sheep, and subsequent fertilization and embryo development occurred (Figure 27.1 [54]). Two of the sheep continued to show normal hormonal cyclicity by progesterone levels up to 36 months post-transplantation, and follow-up MRI studies confirmed normal ovarian size and intact vasculature. Furthermore, in a very recent study, Arav et al. demonstrated that the follicular and endocrine function lasted up to 6 years post re-transplantation [55]. This is the longest documented functional survival of a frozen/thawed whole ovary. The experiments performed by the Arav group used 8- to 12-month-old sheep where, after dissection of the right ovarian artery and vein via laparotomy, the ovaries were removed and perfused through the ovarian artery with 4°C University of Wisconsin (UW) solution containing 10% DMSO for 3 min. Freezing was performed using a novel freezing device, the Multi-Thermal-Gradient (MTG), which utilizes a directional freezing gradient [52, 54, 55]. By advancing the freezing test tube at a constant velocity of 0.01 mm/s through predetermined temperature gradients, freezing was performed at 0.6°C/min until a seeding temperature was reached, and then at 0.3°C/min until –30°C, after which time, the tubes were plunged into liquid nitrogen.
Thawing and grafting was performed 3–14 days later by plunging the Cryovials into a 68°C water bath for 20 s and then into a 37°C water bath for 2 min. The cryoprotectant was removed by reperfusion of the ovarian artery with UW supplemented with 0.5 M sucrose and 10 IU/ml heparin. Ovarian re-transplantation was performed via end-to-end anastomosis of the ovarian artery and vein to the contralateral (the left side) ovarian vascular pedicle of the same sheep via repeat laparotomy. Successful re-anastomosis was documented in five of nine sheep. Progesterone cyclicity was seen 34–71 weeks after transplantation, thus documenting the functional survival of primordial follicles after the freeze-thaw transplant process. Imhof et al. first explored cryopreservation by cannulating the ovarian artery from freshly retrieved porcine ovaries, flushing with RPMI-1640 solution containing 1.5 M DMSO and 10% human albumin for 30 min on ice [56]. The ovaries were transferred to a programmable freezer at a starting temperature of 4°C, and cooled at 2°C/min to 0°C. The temperature was then lowered by 1.5°C/min to –9°C and then by 0.5°C/min to –40°C. Cooling was then continued at 10°C/min to –150°C.
The vials were then plunged into liquid nitrogen. Thawing was performed 3 weeks later by rewarming in air for 2 min before the ovaries were immersed in a water bath at 25°C, and the cryoprotectant was removed from the tissue by washing in saline and fresh medium [52]. Light and electron microscopy were used to evaluate follicular and oocyte survival. Of the primordial follicles, 84.4% in the frozen-thawed ovaries appeared histologically, with 73% of the follicles looking like those from the unfrozen contralateral ovary [56].
After success using the porcine model, Imhof et al. then cryopreserved and re-transplanted whole ovaries in sheep [57]. Using a similar method for cryopreservation, the authors performed a second laparotomy 3–5 weeks later, and the frozen–thawed ovary was autografted by microvascular end-to-end anastomosis to the contralateral pedicle. Ischemia time before complete re-anastomosis was 30 min. The FSH and progesterone levels were used to evaluate ovarian function. Initially, FSH levels kept rising for 3 months but reached normal physiological levels about 6 months after transplantation. Progesterone was first detected 12–14 months after implantation and two of nine sheep resumed normal ovarian function. One sheep achieved a spontaneous pregnancy with delivery of a healthy lamb [57].
Wallin et al. conducted a study to assess methods for the evaluation of viability and function of frozen-thawed whole ovaries [41]. Histology and viability assays were used to evaluate the ovaries. Fourteen ewes underwent oophorectomy via laparotomy and their ovaries were frozen using the slow cooling method.
A solution containing 1.5 M propanediol, 0.1 M sucrose and 2% human serum albumin in Leibowitz L-15 medium was used as the cryoprotectant in one group of ovaries. Ovaries were stored between 1 week and 9 months. No antral follicles were seen in the cryoprotectant group and edema was noted within the stroma. There was no histological difference between the two groups.
Vitrification
While many attempts have been made to cryopreserve whole ovaries by slow freezing techniques, an increasing number of experiments are utilizing vitrification. This method is becoming increasingly recognized as an alternative to organ and tissue preservation by slow freezing as it circumvents the mechanical damage cause by ice crystal formation [58, 59].
Fahy and colleagues have described two vitrification solutions that may be useful for whole ovary cryopreservation. The first, VS1, contains 20.5% wt/vol. DMSO, 15.5% wt/vol. acetamide, 10% wt/vol. propylene glycol and 6% wt/vol. polyethylene glycol in a modified Dulbecco’s saline (HB1) [59]. The second, VS4, contains 2.75 M DMSO, 2.76 M formamide and 1.97 M propylene glycol diluted in BM1 medium [60]. The two solutions, VS1 and VS4, were compared by Courbiere et al. in a sheep model of whole ovary cryopreservation for toxicity to primordial follicles and vessels by collecting ovaries with intact pedicles from 5- to 6-month-old lambs [61]. Each ovary was perfused via the ovarian artery with heparinized Ringer’s solution, followed by perfusion and immersion in a bath with VS1 or VS4 solution. Perfusion rate was performed at 0.35 ml/min with a stepwise increase in concentration of cryoprotectant. After perfusion, ovaries were transferred into cryobags containing the cryoprotectant mix and then plunged into liquid nitrogen. After storage, the vitrified samples were rapidly rewarmed in a 37°C water bath, and the cryoprotectant was removed by a reversed concentration gradient perfusion, and then washed in BM1 medium for 5 min [61].
Histologically, the percentage of normal primordial follicles fell after vitrification, with 25.2%, 7.0% of follicles remaining normal with VS1 and 53.5%, 3.2% remaining normal with VS4. There were also more post-vitrification cytoplasmic anomalies with VS4 (P < 0.05) but more nuclear and combined anomalies with VS1 (P < 0.05). Fractures occurred in vessels during thawing in three of five cases with VS1 and in 8 of 10 cases with VS4, although the authors noted that the catheters used to re-perfuse the ovarian artery were fitted without difficulty. This study showed that whole sheep ovaries can survive vitrification with good immediate follicular viability via histological evaluation; however, the blood vessels were cryodamaged. Similar to previous slow-freezing studies, Courbiere et al. performed a follow-up study in 2008, attempting vascular anastomosis to the contralateral pedicle in sheep ovaries either fresh or after vitrification [62]. Successful microsurgical transplantation was performed in both groups, but, not surprisingly, the median ischemia time was significantly longer in the cryopreservation group. Only one out of five ewes undergoing ovarian vitrification recovered endocrine function six months after transplantation compared to four out of five in the fresh transplant group. However, histological evaluation showed total follicle loss in the vitrification group, suggesting that attempts at cryopreservation with vitrification were unsuccessful, despite technical feasibility [62].
Whole Human Ovary Cryopreservation
Despite the many technical challenges involved in the choice of cryoprotectants and tissue viability after freezing and thawing, there have been several attempts at whole ovary cryopreservation in humans (Table 27.1 [63, 64]). In 2004, Martinez-Madrid et al. tested the feasibility of freezing intact human ovaries using a passive cooling device [29]. Ovaries from three premenopausal women undergoing oopohorectomy were resected with their vascular pedicle intact. The ovarian artery was cannulated and the ovary was perfused first with isotonic heparinized solution, and then with a solution of Leibovitz L-15, 10% DMSO and 2% human serum albumin for 5 min at 2.5 ml/min. The ovary was then placed in a Cryovial and cooled at a rate of 1°C/min to –80°C, at which time it was transferred to liquid nitrogen. For thawing, the Cryovial was directly transferred to a water bath at 60°C.
Table 27.1 Summary of whole human ovary cryopreservation experiments listed according to type of freezing method, cryoprotectant used and outcomes measured to indicate post-thaw viability
Study | N | Surgical method | Freezing method | Cryoprotectant | Outcomes measured |
---|---|---|---|---|---|
Martinez-Madrid et al. [29] | 3 | Laparoscopy | Slow cooling | DMSO | Follicle, stromal cell, vascular viability, histological morphology |
Bedaiwy et al. [30] | 2 | Laparoscopy | Slow cooling (ovaries bisected) | DMSO | Follicle viability, apoptosis |
Martinez-Madrid et al. [35] | 3 | Laparoscopy | Slow cooling | DMSO | Apoptosis, ultrastructural assessment |
Jadoul et al. [50] | 9 | Laparoscopy | Slow cooling | DMSO | Technical feasibility of oophorectomy and freezing |
Patrizio et al. [63, 67] | 11 | Laparoscopy, laparotomy | Slow cooling | EG | Apoptosis, histological morphology |
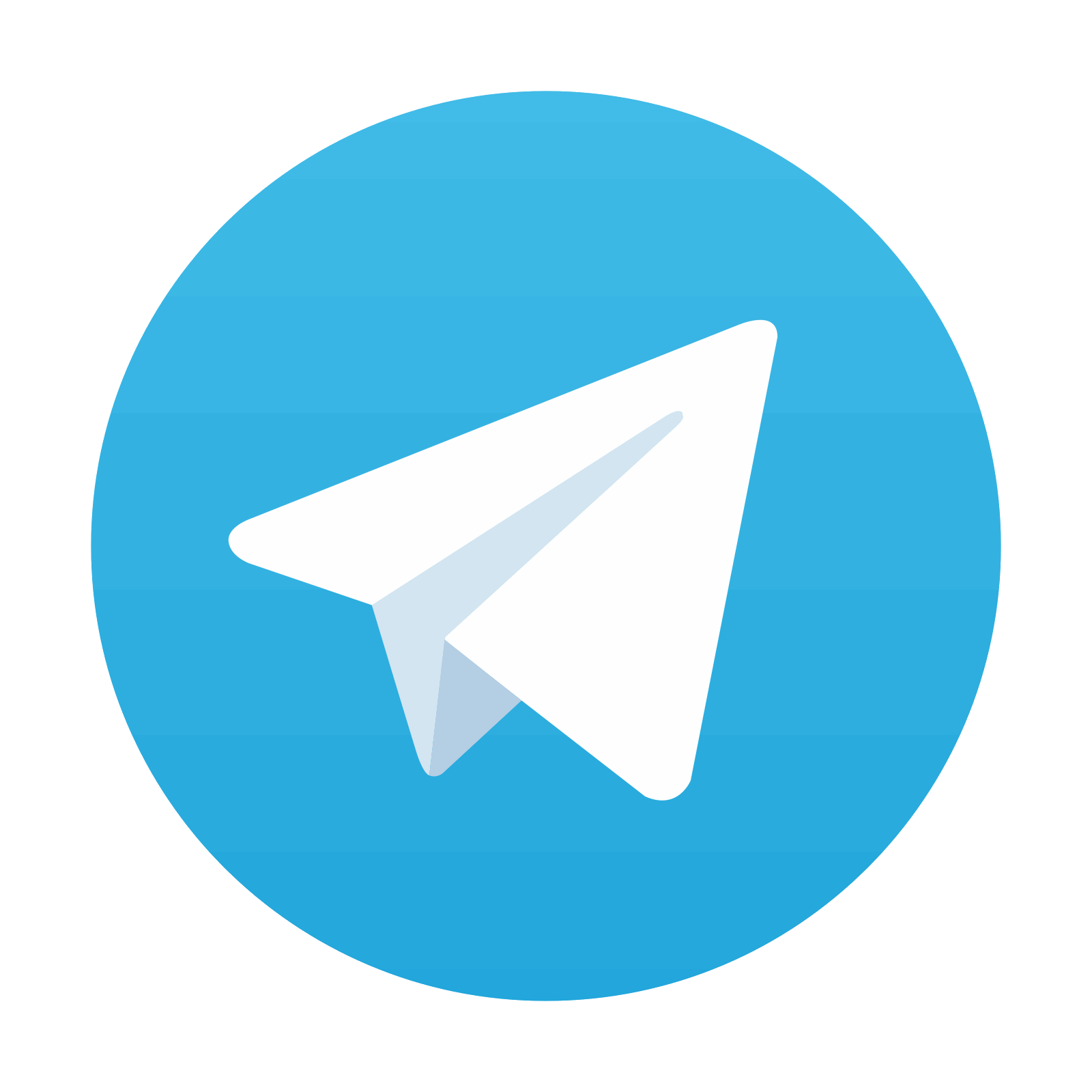
Stay updated, free articles. Join our Telegram channel

Full access? Get Clinical Tree
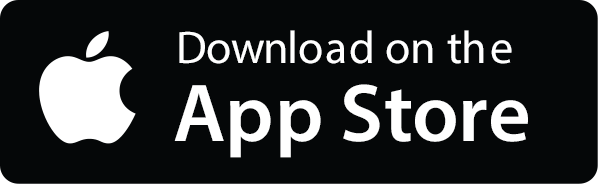
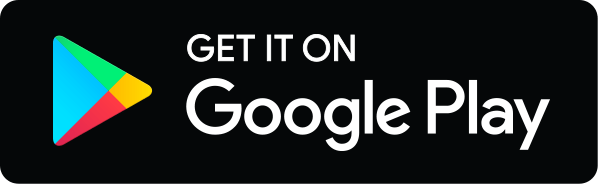
