Abstract
Recent reports state that cancer incidence in children, adolescents and young adults has seen a slight increase since the 1970s [1], but death rates in patients aged 0–19 years have continued to fall. Current 5-year overall survival estimates for childhood cancer exceed 83% (around 90% for most childhood hematological malignancies), translating into a growing population of adult survivors of childhood cancer [1, 2]. It is widely known that most cancer treatments like chemotherapy, radiotherapy and bone marrow transplantation are highly toxic to the gonads, putting girls and women of reproductive age at risk of premature ovarian insufficiency (POI) and subsequent infertility [3–8]. Moreover, non-oncological hematological diseases (thalassemia, aplastic anemia), autoimmune disorders (rheumatoid arthritis, systemic lupus erythematosus) [9–11] and other ovarian pathologies [8] often require treatment that may impair future fertility, exponentially increasing the number of women likely to suffer from iatrogenic menopause or POI.
Introduction
Successful live births after transplantation of frozen-thawed human ovarian tissue have been observed since the first report in 2004 [1]. Up to 2017, approximately 130 live births have been achieved [2]. Currently, this procedure is performed for children, adolescents, and young adults with cancer in many countries.
The standard method of ovarian tissue cryopreservation is slow freezing, but rapid freezing (also called “vitrification”) has increasingly been reported as an alternate cryopreservation method in recent years. The present article reviews recent findings with regard to techniques for vitrification of ovarian tissue.
Advances in Vitrification of Fertilized Embryos/Oocytes
To achieve cryopreservation of fertilized embryos, blastocysts or oocytes for reproductive medicine, slow freezing methods have been developed since around 2000 [3, 4]. In 2005, it was reported that vitrification achieves higher viability rates than slow freezing when employed for cryopreservation of fertilized ova (embryos/blastocysts) or oocytes [5], and vitrification has since become a standard technique. In 1971, Whittingham et al. first achieved cryopreservation of mouse embryos by using highly polymerized polyvinylpyrrolidone (PVP) as the cryoprotectant [6]. In 1972, Whittingham’s group established an equilibrium slow freezing method using 1 M dimethyl sulfoxide (DMSO) as the cryoprotectant, in which the specimen was cooled to –80°C at a slow rate of 0.3°C/min and then cooled further in liquid nitrogen. They reported that more stable cryopreservation of mouse embryos was achieved with this technique [7]. This equilibrium slow freezing method prevents formation of intracellular ice crystals, even when the specimen is thawed slowly, because the cells are sufficiently dehydrated and exist in a near equilibrated state. In 1977, Willadsen performed slow cooling of sheep embryos from −30°C to −48°C using 1.5 M DMSO (increased from 1 M compared with Whittingham) as the cryoprotectant and then further cooled the embryos in liquid nitrogen. He found that the embryos were viable after rapid thawing, and thus established the nonequilibrium slow freezing method for embryo cryopreservation [8]. Unlike the equilibrium slow freezing process, nonequilibrium slow freezing leads to formation of ice crystals within the cytoplasm because the concentration of cryoprotectant is lower and super cooling is increased.
In contrast, vitrification employs a high cryoprotectant concentration to achieve rapid dehydration of cells and replacement of intracellular fluid by the cryoprotectant, enabling the specimen to be stored in liquid nitrogen after equilibration of the cryoprotectant (Figure 24.1). While slow freezing requires almost 4 hours, vitrification allows freezing of specimens in about 30 minutes.
Figure 24.1 Cryopreservation of ovarian tissue
Advances in Slow Freezing of Ovarian Tissue
The same technique is employed for cryopreservation of ovarian tissue as for cryopreservation of embryos/blastocysts/oocytes. However, a tissue specimen contains many cells and is not homogenous, leading to variation of the osmotic pressure and extent of equilibration. Therefore, it was anticipated that ovarian tissue cryopreservation would have a lower success rate than cryopreservation of embryos/blastocysts/oocytes. In 1994, Gosden et al. first reported successful live birth after transplantation of thawed sheep ovaries that had been cryopreserved using DMSO as the cryoprotectant [9], and this protocol is currently employed worldwide for ovarian tissue cryopreservation. For slow freezing of ovarian tissue, the common cryoprotectants are DMSO, ethylene glycol, and propanediol. There have been no reports that ovarian tissue cryopreservation employing ethylene glycol as the cryoprotectant is effective. However, it was reported that cryopreservation of ovarian tissue using ethylene glycol as the cryoprotectant led to successful oocyte release and embryo development after transplantation of thawed tissue specimens[10]. Gook et al. performed cryopreservation of ovarian tissue with propanediol as the cryoprotectant, and confirmed by light and electron microscopy that up to 85% of primordial follicles were structurally intact after thawing. They concluded that propanediol, which has a neutral pH, low molecular weight, and good cell permeability, is the most useful cryoprotectant for slow freezing of ovarian tissue [11]. However, it is still controversial whether DMSO, ethylene glycol, or propanediol is the most useful cryoprotectant for cryopreservation of ovarian tissue.
Advances in Vitrification of Ovarian Tissue
Application of vitrification for cryopreservation of ovarian tissue has been studied since around 2005. In 2009, Keros et al. compared morphological changes of human ovaries between the slow freezing method and the vitrification method with different cryoprotectant concentrations [12]. They performed slow freezing with propanediol + ethylene glycol as the cryoprotectant, while their vitrification method used DMSO + propanediol + ethylene glycol at two concentrations (0.75 M or 1.5 M) as the cryoprotectant. Outcomes were compared by histological evaluation after culture of cryopreserved and thawed ovarian tissue for 24 hours. They found that the interstitial region of thawed ovarian tissue was more morphologically normal after vitrification compared with slow freezing, thus providing the first evidence for the feasibility of clinical use of vitrification for ovarian tissue cryopreservation [12]. And then, Isachenko et al. compared morphological changes of human ovaries, in vitro production of hormones, and glyceraldehyde 3-phosphate dehydrogenase gene expression after culture between the slow freezing method and the vitrification method. They found that the molecular biological analysis demonstrated that the GAPDH gene expression in ovarian tissue after vitrification was dramatically decreased in contrast to conventional freezing [13]. Kim et al. evaluated biomarker expression for autophagy, apoptosis, and DNA damage/repair in fresh, slow frozen-thawed, and vitrified-warmed bovine cortical sections to understand potential causes and mechanisms of somatic and germ cell damage due to ovarian tissue cryopreservation [14]. By Western blot analysis, slow frozen tissue showed activation of autophage as indicated by cleavage of LC3B. In contrast, vitrified tissue exhibited acute activation of apoptosis as evidenced by PARP cleavage. They concluded that slow freezing/thawing appeared to elicit autophage (cell survival pathway) initially followed by apoptosis, whereas the pathway to autophage was bypassed upon warming of vitrified tissue possibly due to the effects of high concentration of cryoprotectants [14]. In 2005, Yeoman’s group compared the slow freezing and vitrification methods using simian ovaries by assessing the percentage of intact follicles after thawing [15]. They found no significant difference in the percentage of intact follicles between the two methods, and they concluded that vitrification could be an option for ovarian tissue cryopreservation in the future [15]. In 2009, Kagawa et al. evaluated the percentage of intact follicles in thawed cow and human ovaries after vitrification. Based on the data thus obtained, they developed a freezing device for human ovarian cryopreservation and reported a high rate of intact follicles after vitrification using this device [16]. Subsequently, further reports have been published on vitrification for cryopreservation of ovarian tissue. In 2011, Amorim et al. compared slow freezing and vitrification for ovarian tissue cryopreservation by reviewing the reports published up to that time. They concluded that it was unclear which method was superior for ovarian tissue cryopreservation, but suggested that slow freezing should remain the standard technique for this purpose. It was pointed out that clear conclusions could not be drawn because the published reports varied widely with regard to ovarian morphology and fat content within the same animal species, as well as with respect to the cryoprotectant concentration and composition, equilibration time, freezing device, freezing solution, thawing solution, culture time after thawing, and method of evaluation [17]. In 2011, Ting’s group compared ovarian tissue after vitrification and thawing with unfrozen control ovarian tissue by electron microscopy, HE staining, and immunostaining with PPH3 (a mitosis marker). They found no significant difference between the two tissues and concluded that vitrification was useful for ovarian tissue cryopreservation [18]. In the same year, Sheikhi reported on the results of cryopreserving human ovarian tissue by vitrification using DMSO + 1,2-propanediol + ethylene glycol + PVP as the cryoprotectant [19]. On both light and electron microscopy, there were no notable differences between vitrified/thawed ovarian tissue and unfrozen control tissue, suggesting that vitrification was clinically applicable for ovarian tissue cryopreservation [19].
In 2016, Lee et al. compared transplantation of vitrified/thawed ovarian tissue and unfrozen control ovarian tissue in mice by assessing the blood gonadotropin level, histological follicular development, and neovascularization at 2, 7, and 21 days after transplantation. On day 21, there were no differences between the groups of mice transplanted with vitrified/thawed ovarian tissue or unfrozen ovarian tissue in terms of the primordial follicle density, primary follicle density, apoptosis, follicle-stimulating hormone (FSH) level, and neovascularization rate. They concluded that ovarian tissue vitrification achieved stable follicle viability and their data suggested that ischemic injury after transplantation was more harmful than cryoinjury [20].
In 2017, Shi et al. conducted a meta-analysis of 14 studies that compared slow freezing with vitrification as methods of ovarian tissue cryopreservation [21]. They concluded that these two methods were similar in terms of primordial follicle density, but vitrification led to less DNA fragmentation than slow freezing. Accordingly, they suggested that vitrification may be morphologically more suitable for storage of stromal cells [21].
In 2017, Laronda’s group developed an ovarian tissue vitrification kit that met FDA standards. No significant differences were found when they compared DNA damage between fresh ovarian tissue and vitrified/thawed ovarian tissue after cryopreservation with this kit, and they concluded that their vitrification kit was effective [22]. Over the past several years, multiple reports have been published on PubMed comparing the slow freezing and vitrification methods or comparing cryoprotectants. However, it remains unclear whether slow freezing or vitrification is the superior technique because of differences in the species studied, cryoprotectant composition, and assessment methods, as previously reported by Amorium [17]. Table 24.1 showed the protocols used for human ovarian tissue vitrification from 1999 to 2018. The slow freezing method is the current standard technique for cryopreservation of ovarian tissue. Although transplantation of thawed ovarian tissue after cryopreservation by vitrification is becoming more common, chiefly in Asia, it will require more time to complete evaluation of this method based on restoration of the menstrual cycle and the live birth rate, as was the case for the slow freezing method.
Table 24.1 Protocols used for human ovarian tissue vitrification
Article | Vitrification Protocol Equilibration media1 (➡Equilibration media2) ➡Vitrification Solution | Basal media | Supplemented serum | Main outcome measures after thawing | Background protocol | Comments |
---|---|---|---|---|---|---|
|
| PBS | 10mg/ml HSA |
| Gook et al., 1993 (Ref30) | Highest levels of intactness achieved with slow cooling |
Rahimi et al., 2004 (Ref 33) |
| DPBS | 15% FCS |
| Isachenko et al., 2002 (Ref 32) |
|
|
| Leibovitz L-15 | 25mg/ml HSA |
| Isachenko et al., 2002 (Ref 32) |
|
|
| TCM-199 | 20% SSS |
| Kagawa et al. 2007 (Ref36) |
|
|
| HBSS | 10mg/ml HSA |
|
| |
Amorim et al., 2012 (Ref36) |
| MEM | 20mg/ml HSA |
| Huang et al., 2008 (Ref34) |
|
|
| TCM-199 | 20% SSS |
| Hashimoto at al., 2010 (Ref25) |
|
RT:room temperature, PrOH:propanediol, DMSO:Dimethyl sulfoxide, EG:ethylene glycol, PG:propylene glycol, PVP:polyvinylpyrrolidone,
HSA:Human serum albumin, FCS:Fetal Calf Serum, SSS:Serum Substitute Supplement
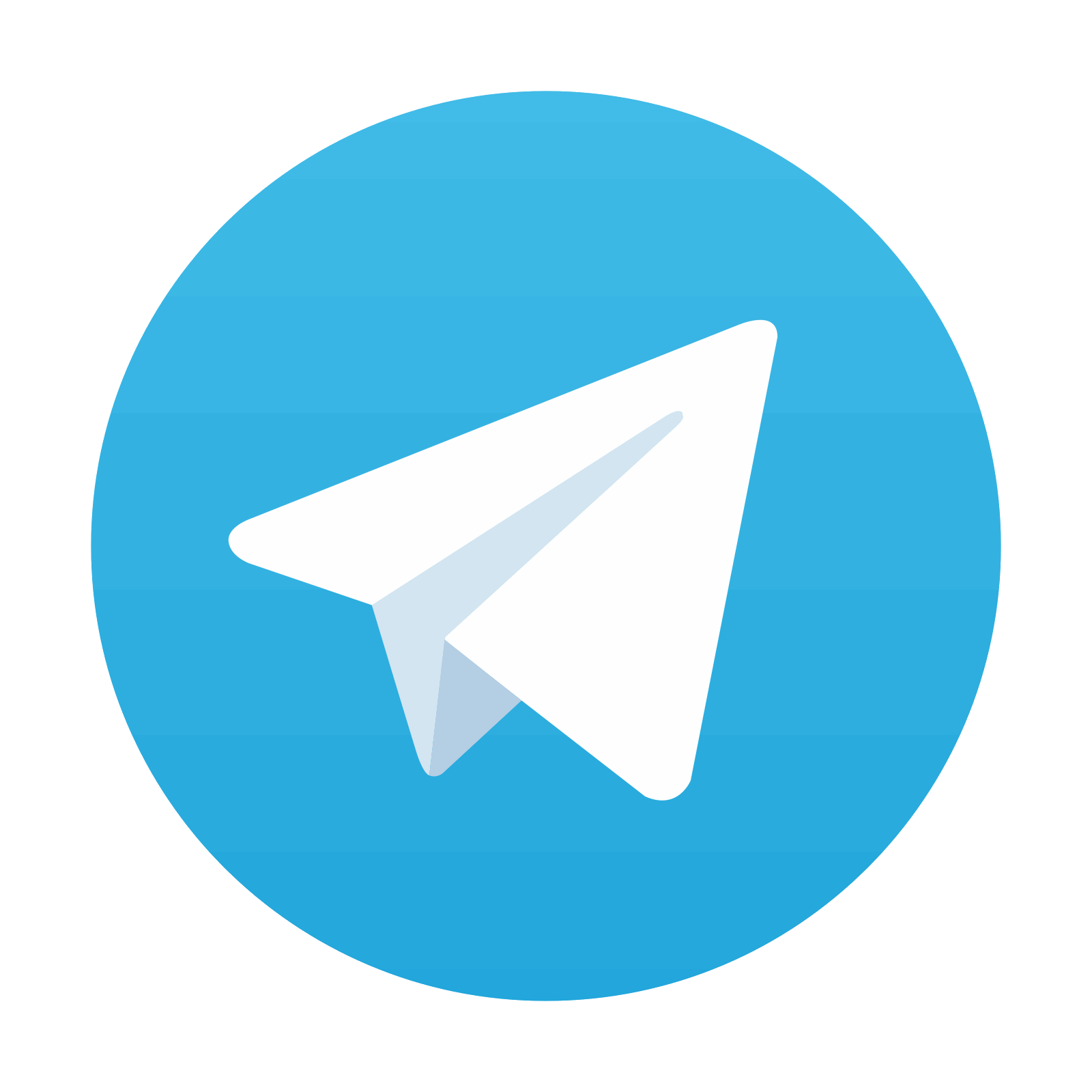
Stay updated, free articles. Join our Telegram channel

Full access? Get Clinical Tree
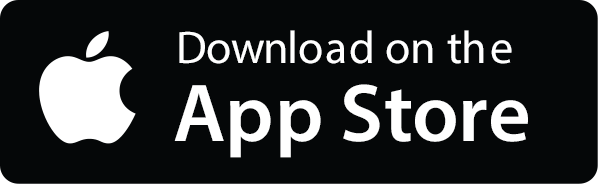
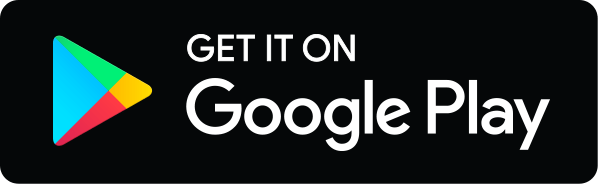
