Abstract
Cryopreservation is a fundamental adjunct for any in vitro fertilisation (IVF) programme for both gametes and embryos. Sperm can be frozen for use in donor cycles, treatment cycles or to preserve fertility. After the first successful frozen-thawed embryo live birth was reported in 1983, embryo cryopreservation is routinely used to store supernumerary embryos, to improve cumulative pregnancy rates and to help effectively implement a single embryo transfer policy. However, oocyte cryopreservation has proved to be more challenging as the survival rates and pregnancy rates were initially low.
1 Introduction
Cryopreservation is a fundamental adjunct for any in vitro fertilisation (IVF) programme for both gametes and embryos. Sperm can be frozen for use in donor cycles, treatment cycles or to preserve fertility. After the first successful frozen-thawed embryo live birth was reported in 1983, embryo cryopreservation is routinely used to store supernumerary embryos, to improve cumulative pregnancy rates and to help effectively implement a single embryo transfer policy. However, oocyte cryopreservation has proved to be more challenging as the survival rates and pregnancy rates were initially low.
Improvements though in cryopreservation techniques for both oocytes and embryos have increased the effectiveness and efficiency and therefore widened the application of these techniques. For oocyte cryopreservation there has been a shift from it being classed as experimental to it being offered as a mainstream treatment option. The improvements to embryo cryopreservation and a greater understanding of endometrial receptivity have now changed the perception that fresh embryos have higher success rates than cryopreserved embryos. Further evidence shows that controlled ovarian stimulation cycles have poorer obstetric and perinatal outcomes [1] and that ‘freeze all’ cycles can reduce the complication of ovarian hyperstimulation syndrome (OHSS). Subsequently this may see a shift to performing more frozen embryo transfers than fresh embryo transfers. Centres therefore need to optimise their cryopreservation programmes to ensure the best outcomes for patients.
This chapter will review the current techniques and applications for cryopreservation.
2 Cryopreservation Methods
2.1 Use of Cryoprotectants
Although discovered by accident in 1949, the use of cryoprotectants for both gametes and embryos is essential to maximise survival and ensure viability is preserved. They reduce intracellular ice formation by facilitating dehydration of the cell to minimise intracellular water. Permeating cryoprotectants such as glycerol, propandiol (PROH), dimethyl sulphoxide (DMSO), or ethylene glycol (EG) enter into the cell to displace water. Non-permeating cryoprotectants (e.g. sucrose) do not permeate the cell but increase the extracellular osmolarity to enhance dehydration by generating an osmotic gradient across the cell membrane. Although used very successfully, caution needs to be exercised because if intracellular concentration of cryoprotectants becomes too high there may be a toxic effect.
2.2 Rapid Freezing
Rapid freezing is a simple method which is used for sperm cryopreservation where after drop-wise addition of cryoprotectants and equilibration, the straws are placed in direct contact with liquid nitrogen vapours before plunging into liquid nitrogen [2]. Although this method is routinely used, there is a lack of reproducibility and control of overcooling rates.
2.3 Slow Freezing
Slow freezing is a well-established method for cryopreservation. The first protocol for embryo cryopreservation was reported in 1985 [3] with more recent modifications only really being applied to the concentrations of cryoprotectant in the media. Slow dehydration is achieved by using programmable equipment set to initial cooling rates of -0.3 to -0.5 oC/min for embryos and -0.5 to -1 oC/min for sperm. This utilises relatively low concentrations of cryoprotectants; the concentration of the permeating cryoprotectants is approximately 1.5 M (usually PROH), and the concentration of non-permeating cryoprotectants is 0.1–0.3 M [4]. Dehydration is achieved by exposing the cells to hypertonic solutions of cryoprotectants at supra-zero temperatures followed by a progressive increase in solute concentration of the extracellular phase due to extracellular ice formation. Ice nucleation (seeding) which is induced at -5 to -8 oC controls ice formation and therefore prevents release of latent heat which could disrupt the cooling rate. During a slow rate freezing programme when dehydration of the cellular environment is almost complete (after -40 oC) the rate of cooling is increased and cells can be placed into liquid nitrogen [4]. The majority of embryo cryopreservation until more recently was carried out using this methodology with survival rates being in the region of 60–80%.
2.4 Vitrification
Boldt [5] describes vitrification as a process by which an aqueous solution is converted to a solid, glass-like amorphous substance by rapid changes in temperature of the solution. This process which is being increasingly used for oocytes, early cleavage embryos and blastocysts involves usually a 10–15 minute pre-equilibration period with low concentrations of cryoprotectants (1–2 mol/l) followed by a short exposure time to high permeating cryoprotectant concentrations (5–6 mol/l) and non-permeating cryoprotectants (0.5–1.0 mol/l). Rapid cooling is then induced (>20,000 oC/min). The high concentrations of cryoprotectant increase viscosity to prevent ice nucleation.
There are multiple commercially available devices for vitrification which can be classified as open or closed. Open systems have direct contact with liquid nitrogen during cooling and storage. Although successful, concerns have been raised regarding contamination risks from liquid nitrogen. Closed systems circumnavigate this problem but the disadvantage is that they cannot achieve a high cooling rate and may have the potential toxicity effects of longer exposure to high concentrations of cryoprotectants. However, studies have shown that closed systems are as equally effective as open systems [6].To ensure vitrification is a success, warming rates must also be considered to prevent ice nucleation.
Edgar and Gook [7] suggested in their critical appraisal of cryopreservation methods that vitrification shows higher survival rates than slow freezing and therefore should be the method of choice for oocytes and blastocysts, but either method can be used equally effectively for cleavage stage embryos.
3 Sperm Cryopreservation
Cryopreservation of human sperm was first described more than 50 years ago and is now used routinely for a range of clinical applications: treatments with donor sperm, fertility preservation and IVF treatment. More recently there has been an increase in the use of surgically retrieved sperm and having the ability to successfully store testicular sperm negates the need for further surgery. A meta-analysis [8] concluded that there was no difference between fertilisation rates and clinical pregnancy rates when using fresh versus cryopreserved-thawed testicular sperm which makes sperm cryopreservation for azoospermic patients an effective option.
However, it is notable in the laboratory that parameters such as motility, velocity and viability are altered after cryopreserved samples are thawed. However, more subtle changes may occur such as damage to the structure and function of sperm, which in turn may affect the fertilisation potential of the sperm.
A study [9] combined examining the ultrastructural changes with sperm parameters after cryopreservation. The group reported that viability significantly decreased which was thought to be related to the physical and chemical environment during the process including the high solute content of intracellular fluid following dehydration, toxic effects of cryoprotectants and damage to the membranes by rapid changes in osmolarity and temperature. Motility was also significantly affected with an increase in the percentage of immotile sperm after thawing. By using scanning and electron microscopy it was found that the percentage of normal morphological sperm decreased after thawing. Furthermore, after examining organelles it was found that the acrosome, which is vital for the process of fertilisation, was the most severely affected. This study found no differences relating to chromatin and nuclear content morphology. However, there is no consensus on this final point as cryopreservation was found to induce chromatin decondensation [10] and cause an increase in sperm DNA fragmentation [11].
There is still much work to do before the optimal method of human sperm cryopreservation is determined. The preferable technique may be to use methods which do not require cryoprotectants to reduce osmotic and toxic effects. Ozkavukcu [9] suggested that different methods may work best for different groups, for example, simple vapour freezing for donor sperm but more complex methods for sub/infertile men. There is evidence more recently that specific devices can be used effectively for very low sperm counts and individual sperm [12]. Small volume vitrification devices are also being developed which negate the need for permeating cryoprotectants [13].
Whilst sperm banking for post-pubertal men is routinely offered, preserving fertility for prepubertal boys who do not yet produce sperm cells has been impossible. However, advances in spermatogonial stem cell storage followed by transplantation in animal models may provide hope for a future solution [14].
4 Oocyte Cryopreservation
Renewed interest in oocyte cryopreservation, especially in countries where the creation of supernumerary embryos is forbidden, has led to improvements in survival and pregnancy rates such that now oocyte cryopreservation is considered a routine treatment option for medical, social and legal reasons.
Initially poor survival rates were attributed to the surface area/volume ratio and its relationship with the cell permeability due to the large size of the oocyte [15]. However, zygotes with almost identical surface/volume ratios can be successfully cryopreserved and therefore it is the differences in membrane permeability not surface/volume ratio which influence survival rates of oocytes. However, oocytes are susceptible to cryodamage and may be damaged due to chilling injury which could lead to ultrastructural damage. However, since the introduction of vitrification, spindle recovery has been shown to have improved; there is less cortical granule loss and vacuolisation and increased survival rates have been reported. Furthermore a systematic review and meta-analysis [16] concluded that there was no difference between vitrified and fresh oocytes in terms of fertilisation, cleavage and clinical pregnancy rates, although there was a reduced ongoing pregnancy rate in the cryopreserved group.
Further studies are required especially where the same end points are defined to ensure patients are provided with evidence-based statistics so they can make an informed choice, especially if they are choosing to delay childbirth for social reasons. However, for patients considering oocyte cryopreservation for fertility preservation reasons, it offers realistic hope and overcomes the ethical issues if embryos are frozen and a partner withdraws consent for the embryos to be used in a treatment.
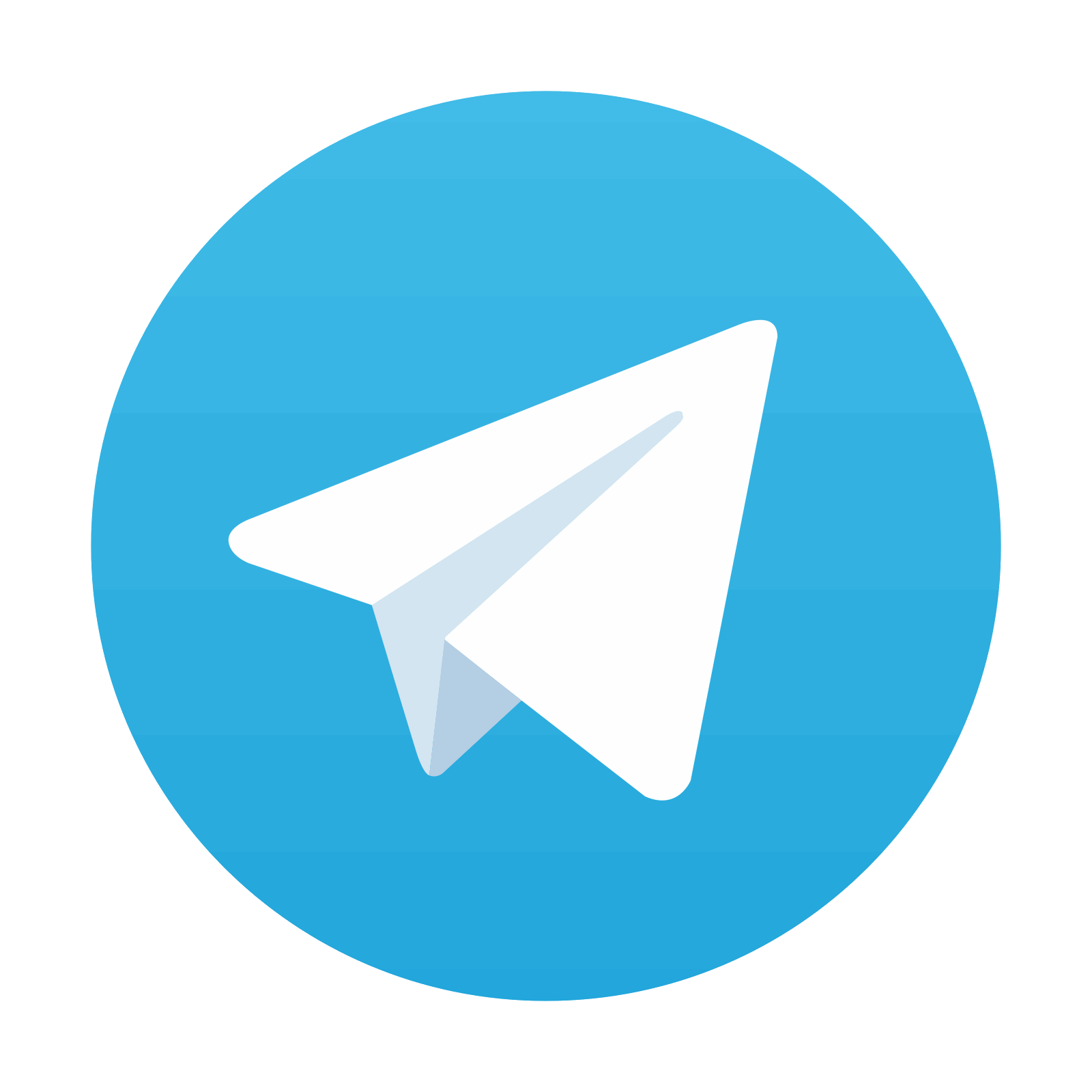
Stay updated, free articles. Join our Telegram channel

Full access? Get Clinical Tree
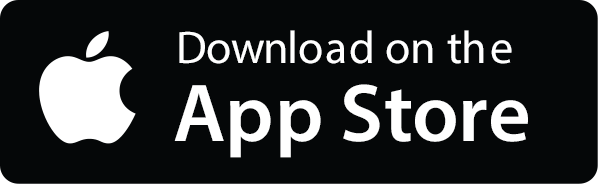
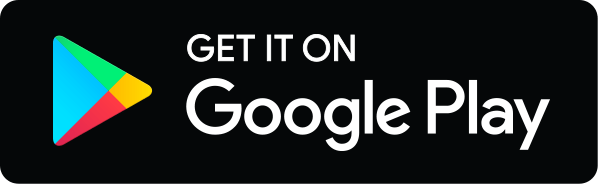