Abstract
Male infertility is a common condition estimated to be a contributory factor in as many as 50% of couples experiencing problems with conception. It is also a key catalyst for the increasing uptake of assisted reproductive technologies (ART). Traditionally, andrological practice has relied on the use of ostensibly descriptive criteria; seeking to define a male’s fertility status based on the number of motile, morphologically normal spermatozoa present in their ejaculate. Notwithstanding the widespread adoption of such measures, over time we have come to appreciate their limitations and that fertility is more accurately predicted on the basis of sperm quality. This realisation poses the fundamental question of what constitutes a high-quality, fertilisation competent spermatozoon. Here, we consider recent advances in our understanding of the mechanistic basis of sperm function that are driving innovations in our ability to diagnose and treat male infertility, thus optimising outcomes for the management of male fertility.
2.1 Introduction
Infertility is a distressingly common condition that afflicts up to 15% of couples of reproductive age. Male factor infertility is directly implicated in approximately 30% of couples experiencing problems with conception and is a contributory factor in as many as 50% of cases [1]. In the 40 years since the first description of successful human in vitro fertilisation (IVF), substantial improvements have been made in the efficacy of treatments for male subfertility. One such advance has been the introduction of gamete micromanipulation techniques such as intracytoplasmic sperm injection (ICSI), which have had a transformative impact on the treatment of individuals with compromised sperm parameters, effectively dispensing with the need for spermatozoa to display progressive motility or have the potential to recognise an oocyte and complete an acrosome reaction [2]. So profound has the impact on clinical andrology been, that ICSI has become the favoured choice for fertilisation irrespective of the male factor, now featuring in as many as two-thirds of IVF cycles undertaken in countries such as Australia [3]. However, despite technological advances, both worldwide clinical pregnancy and live birth rates remain relatively modest at approximately 26.8% and 20% per started cycle, respectively [2]. Notwithstanding confounders, recent epidemiological data has documented the potential for an elevated risk of birth defects and perpetuation of defective semen profiles in children conceived via ICSI [4,5]. Such data encourage caution in the utilisation of ICSI beyond its intended application for achieving pregnancy in couples with severely compromised semen parameters and highlight a pressing need to explore new horizons for diagnostic tools to assist with patient stratification, sperm selection and therapeutic treatment options for males suffering from subfertility and infertility. Here, we review the current state of the art and future directions in the field of male infertility with the goal of addressing the long-standing question of how best to approach the clinical management of these individuals. Out of necessity, we have also sought to direct the reader to a number of excellent reviews that critically appraise our current armoury of diagnostic and therapeutic strategies.
2.2 Diagnostic Andrology
Without question, there are many different pathologies captured under the umbrella of male infertility and each of these presents different implications for the clinical management of the patient. Until recently, our approach to diagnostic andrology has been based upon the tenet that fertility can be defined in terms of a routine descriptive semen profile that places emphasis on sperm count, morphology and motility. Regrettably, these conventional criteria reveal little about the underlying aetiology of the infertility or the functionality of the sperm, have proven to be relatively insensitive indicators of fertilisation success, and are therefore of limited utility in selecting the optimal clinical management regimen [6]. Indeed, we now appreciate that stalwarts of andrological assessment such as sperm number represent relatively weak criterion for the prediction of fertility. Likewise, despite progress towards automation of gross morphology assessments and the advent of ultra-high magnification (i.e. motile sperm organelle morphology examination [MSOME]) to standardise the detection of even very subtle morphological defects, limitations remain in terms of the ability to discriminate those morphological elements that define the functionality of a given sperm cell. Thus, whilst the combination of MSOME in tandem with ICSI (i.e. intracytoplasmic morphologically selected sperm injection [IMSI]) has a theoretical potential to improve reproductive outcomes, there is currently limited evidence supporting the positive effect of IMSI on live birth or miscarriage rates, and the veracity of evidence citing improved clinical pregnancy following IMSI remains questionable [7]. It has yet to be determined whether such deficiencies may be overcome through the application of selective stains to highlight particularly important attributes of sperm structure and quality, such as those reflecting sperm viability, acrosomal status, capacitation, mitochondrial membrane potential, reactive oxygen species (ROS) generation, lipid peroxidation, apoptotic markers and DNA damage.
As the final component of the routine descriptive semen profile, sperm motility holds promise, particularly when undertaken with the aid of objective computer-aided semen analysis (CASA) systems to accurately measure sperm trajectories [8]. Accordingly, positive correlations have been established between the motility and fertility of human spermatozoa [9]. Regrettably, owing to the fact that the motility profile of sperm is a constantly changing entity influenced by the differing physiological environments encountered by the cell, these correlations are often weak. Indeed, during their transport to the site of fertilisation, spermatozoa are variably characterised by forward progressive motility, complete quiescence associated with the formation of a storage reservoir in the isthmic region of the fallopian tubes, and the induction of hyperactivated motility characterised by high‐frequency, high‐amplitude, asymmetric flagellar waves. Whilst the measurement of these various forms of movement can be achieved by CASA [8], problems associated with the intermittent nature of these profiles render them difficult diagnostic criteria to apply in a clinical setting. Moreover, the selection of sperm on the basis of their motility using “swim-up” techniques has failed to deliver superior pregnancy rates to that of the most widely adopted sperm preparation procedures utilising colloidal silica density gradients [10].
2.3 Improvements in Diagnostic Andrology
In order to alleviate the limitations and variability inherent in current methodologies used for fertility diagnosis and selection of spermatozoa in assisted reproductive technology (ART), new modalities are urgently required. In terms of driving advances in this field, we have much to learn from the study of the mechanistic basis of sperm dysfunction and, conversely, the characteristics of those sperm cells that are able to participate in the cascade of events that underpin natural conception. In this setting, it is evident that only relatively few sperm cells successfully navigate the female reproductive tract to reach the site of fertilisation in the oviduct. This highly selected population not only are endowed with the motility needed to penetrate through cervical mucus and gain entry into the uterus and the oviducts, but also complete the process of capacitation, which primes the cells for their interaction with the outer vestments of the oocyte [11], the cumulus oophorus and zona pellucida. Such interactions are coordinated by specialised sperm domains overlying the anterior region of the sperm head. These domains are formed during the latter phases of spermatogenesis before being dynamically modified upon passage through both the male and female reproductive tracts [12]. Accordingly, the development of bioassays that seek to impose the stringency of natural sperm selection barriers have been receiving increased attention (Figure 2.1).
Figure 2.1 Tools under consideration for the diagnosis of male infertility. (A) As sperms enter the reproductive tract they transit through the uterus, navigate the uterotubal junction and progress through the isthmus and ampulla of the oviduct to contact the ovulated oocyte. (B) To achieve fertilisation, spermatozoa must first undergo an extensive period of membrane remodelling in the female reproductive tract, termed capacitation, where dynamic protein phosphorylation events give rise to important physiological changes in motility (hyperactivated motility). These dynamic protein changes also facilitate the presentation/unmasking of key receptors on the sperm surface that facilitate sequential cumulus matrix (hyaluronan) and sperm–zona pellucida binding and penetration. Spermatozoa are required to undergo exocytosis of their acrosomal contents and acrosomal membrane remodelling events prior to cell–cell membrane fusion and fertilisation. Post-fertilisation, there is mounting evidence that sperm-borne small non-coding RNAs (sncRNAs) influence pre-implantation embryogenesis. (C) Currently, routine protocols for the diagnosis of male infertility and subfertility include basic and advanced morphology, motility and sperm concentration assessments (including computer-assisted sperm analysis (CASA), World Health Organisation (WHO) assessment criteria and motile sperm organelle morphology assessment (MSOME). In addition, there are now several tools available to determine cell quality, DNA integrity and fertilisation capacity (including hyaluronan-based detection methods, high-throughput flow cytometry assays for the oxidised base 8-hydroxyl-2-deoxyguanosine (8-OHdG) and the sperm chromatin structure assay (SCSA). Finally, the advance of omics technology is permitting the development molecular biomarkers that can stratify infertile and fertile sperm populations and aid our understanding of the genetic causes of male infertility.
2.3.1 Hyaluronic Acid Binding
Illustrative of strategies designed to mimic physiological sperm selection are protocols based on the hyaluronic acid (HA) binding properties of spermatozoa. Such techniques seek to exploit the principle that functionally mature spermatozoa express hyaluronic acid binding sites capable of adhering to, and digesting, the hyaluronic acid-rich matrix of the cumulus oophorus [13]. Two systems are currently marketed based on hyaluronic acid sperm selection, differing with respect to their use of either immobilised HA (i.e. physiological intracytoplasmic sperm injection (PICSI)) or HA suspended in a viscous medium (i.e. SpermSlow), which theoretically immobilises or retards mature sperm movement respectively, and is thus permissive of their selection away from immature counterparts in preparation for downstream ICSI [13]. Despite the promise of such interventions, meta-analyses have concluded that current evidence is insufficient to support their utility in terms of improved clinical pregnancy rates, miscarriage rates and, perhaps more importantly, in live birth rates from ART [14]. Available evidence is also insufficient to preclude adverse effects or establish differences in the efficacy of the PICSI versus SpermSlow HA binding systems [14]. By way of a biological explanation for the lack of a clear consensus regarding the efficacy of HA-based sperm selection, there is mounting evidence that human spermatozoa experience a dynamic, capacitation-associated remodelling of their surface architecture [11]. This process is characterised by an apparent reduction in the number of spermatozoa with hyaluronidase enzymes (i.e. sperm adhesion molecule 1, (SPAM1)) superficially exposed on their surface. Conversely, capacitated populations of human spermatozoa are characterised by an increase in the proportion of cells with zona pellucida (ZP) receptors presented on the outer leaflet of their plasma membrane [15]. One such receptor is arylsulphatase A (ARSA), an enzyme with affinity for the sulphated sugar ligands that decorate the ZP, and which is almost exclusively expressed on the surface of capacitated spermatozoa. Thus, HA adhesion may in fact be selecting the sub-population of spermatozoa that have failed to complete capacitation, but the extent to which this is reflective of underlying lesions in capacitation-associated signalling remains to be determined.
2.3.2 Zona Pellucida Binding
Downstream of HA binding, spermatozoa encounter the ZP, an acellular matrix that surrounds the ovulated oocyte and serves as a tenacious physiological barrier to fertilisation. Accordingly, a failure to bind and penetrate the ZP is a relatively common defect encountered in the spermatozoa of infertile patients. Indeed, approximately 10% of men with idiopathic infertility exhibit a failure of sperm–ZP recognition [16]. For most infertile males, however, failures of sperm–ZP recognition are a matter of degree and when carefully quantified using a hemizona assay (HZA) provide an accurate assessment of overall fertility, both in vivo and in vitro [17]. Thus, the HZA has historically been found to provide the highest discriminatory power for fertilisation success/failure of any sperm parameter assessed [17]. Such findings raise the prospect that the ZP may possess the ability to discriminate superior quality spermatozoa, a notion supported by demonstrations that the ZP selectively binds sperm with normal motility, morphology and high levels of nuclear chromatin DNA integrity [18]. This phenomenon may be attributed, at least in part, to the fact that each of these functional parameters are highly sensitive to physiological insults, such as those arising from elevated levels of ROS, which the sperm of infertile individuals commonly encounter [19].
As an extension of these observations, biological selection of sperm for ICSI on the basis of their ZP binding affinity has been shown to produce higher quality embryos and contribute to improved implantation and clinical pregnancy rate compared to sperm selected by conventional subjective approaches [18]. Despite the biological importance of ZP binding, the fact that this barrier can now be readily breached through ICSI has contributed to a situation whereby the molecular basis of sperm–ZP recognition remains poorly characterised [12]. What we do appreciate is that this interaction involves both lectin-like and protein–protein interactions, raising the prospect of utilising these ligands as unique molecular signatures with which to discriminate high-quality, fertilisation competent spermatozoa. Whilst resourcing and ethical limitations prohibit the use of native ZP for this purpose, it has long been known that complex carbohydrate substrates such as fucoidan or neoglycoproteins terminated with the sialyl-Lewisx (sLex) sequence can competitively inhibit human sperm–ZP binding [20,21]. These findings support the hypothesis that selective carbohydrate motifs could be employed for sperm selection. As an extension of this principle, the prospect of developing advanced sperm selection strategies based on surface properties that differentiate viable mature spermatozoa such as negative charge, exposure of apoptotic markers, or birefringence properties have also been trialled and are briefly discussed below. It is important to note, however, that while each of these sperm selection strategies has shown promise, more detailed randomised evaluation of their efficacy is needed to support their widespread adoption in clinical practice [14].
2.3.3 Sperm Surface Properties
As with all cell surfaces, the spermatozoon is adorned with a dense glycocalyx matrix comprising a heterogeneous array of complex carbohydrates. This extracellular coat is subjected to substantial temporal remodelling during the successive phases of sperm maturation, with an uptake of glycoproteins rich in terminal sialic acid residues being a key hallmark of those cells that have completed their epididymal maturation [22]. Thus, a gradient of increasing sialylation is associated with the attainment of full sperm fertilising ability and has also been linked to the masking of the allogeneic sperm cell from immune surveillance upon entering the female reproductive tract [22]. However, in addition to its protective role, the attendant increase in electronegativity associated with sialylation has been exploited as a means by which to electrophoretically separate mature sperm away from contaminating leucocytes, bacteria and immature germ cells, all of which can exert deleterious effects on sperm function, prior to their use in either IVF or ICSI. In recent developments, novel electrophoretic approaches have seen application for the rapid isolation of populations of motile, viable, morphologically normal spermatozoa exhibiting high levels of DNA integrity [23]. Clinical utility has also been demonstrated using a range of difficult starting materials (biopsies, cryostored semen and snap-frozen sperm suspensions), culminating in the first reported human pregnancy following electrophoretic sperm isolation [23]. In a similar context, electrokinetic properties such as the zeta potential (i.e. the charge that exists across the plasma membrane, which in mature spermatozoa is ~ −16 mV to −20 mV) has also been used to separate mature sperm cells via adherence to a positively charged solid support such as a glass slide or centrifuge tube [24]. Such methods have been reported to select for spermatozoa with enhanced levels of maturity, DNA integrity, normal morphology and kinematic parameters that are each associated with increased fertilisation and pregnancy success following ART [25].
One of the leading causative agents of male infertility is oxidative stress originating from elevated levels of ROS within the male germ line [19]. Spermatozoa are particularly susceptible to such stress, not only because they are enriched in vulnerable substrates (i.e. polyunsaturated fatty acids, proteins and DNA), but they also possess little capacity to protect themselves from oxidative attack or to effect any repair, should damage occur [19]. Upon the induction of oxidative stress, spermatozoa revert to an apoptotic cascade resulting in a loss of their fertilisation potential and the externalisation of phosphatidylserine, the latter being a late onset event that may facilitate the phagocytosis of senescent spermatozoa without the accompanying generation of an inflammatory response within the female reproductive tract [26]. Accordingly, the appearance of phosphatidylserine residues on the external surface of the apoptotic sperm membrane has been used for the development of negative selection protocols to effectively eliminate these moribund cells from within an ejaculate prior to ART interventions. Applications formulated on the basis of this strategy include magnetic activated cell sorting (MACS) and glass wool separation technologies [14]. However, whilst systematic review and meta-analyses of prospective randomised trials allude to improvements in pregnancy rates when MACS is used in tandem with standard sperm selection methods (i.e. density gradient centrifugation or swim-up techniques), at present no differences have been established in regard to implantation and miscarriage rates, and further studies of suitable quality are required before advocating for the use of this sperm selection technique in clinical practice [14].
Aside from physical changes in the sperm surface that correlate with their functional status, the maturing cells also experience changes in an optical property known as birefringence. Birefringence refers to a substrate having a refractive index, which is influenced by the polarisation and propagation direction of light. The anisotropic optical characteristics of the spermatozoon are attributed to a combination of longitudinally oriented protein filaments housed within the cell’s nuclear, subacrosomal and axonemal domains. Accordingly, based on similar principles to the MSOME approach described in Section 2.2, the application of polarisation microscopy has been proposed as a novel diagnostic tool for structural evaluation of the maturity of a given sperm cell population, and as a promising method for selection of viable sperm cells with normal morphology and reduced levels of DNA fragmentation in preparation for ICSI [27].
2.3.4 Sperm Biomarkers
With the limitations inherent in existing strategies for defining male fertility, attention has increasingly been directed towards the identification of alternative non-invasive biomarkers with the sensitivity to discriminate signatures of the underlying pathophysiology giving rise to a particular infertility phenotype and/or to accurately diagnose the likelihood of fertilisation success. Indeed, the advent of advanced “omic” platforms has been the catalyst for a recent proliferation in studies seeking to differentiate the macromolecular composition (e.g. proteomic, metabolomic and epigenomic) of spermatozoa and seminal fluids from fertile individuals versus those produced by their subfertile/infertile counterparts [28].
In the absence of de novo protein synthesis, the maturation of spermatozoa is largely dependent on the acquisition of new proteins encountered during their transit of the male reproductive system and the post-translational modifications of their intrinsic protein complement during their post-ejaculatory capacitation [29]. These features highlight the utility of proteomic analyses in terms of characterising the changes that confer functionality on the male gamete. Accordingly, considerable effort has been devoted to defining the sperm proteome, as well as that of the acellular fraction of seminal fluid and the trillions of extracellular vesicles it contains [30]. In terms of the spermatozoon, this expanding resource has recently been consolidated into an inventory of some 6,198 unique proteins, a comprehensive reference library representing approximately 80% of the estimated 7,500 total proteins that constitute a human sperm cell [31]. Among the key challenges that remain in harnessing the transformative potential of this information, is to characterise the targets impacted by post-translational modifications, investigate the protein interactome, and define anomalies in protein expression associated with specific lesions in sperm function [29].
In this context, exciting advances have been made in sophisticated comparative and quantitative approaches that enable analysis of the proteomic signature of spermatozoa in different functional states (immature vs mature, non-capacitated vs capacitated, fertile vs infertile). These techniques are helping to define which specific elements of the proteome are of functional significance and to improve our understanding of the post-translational modifications (e.g. phosphorylation, glycosylation, acetylation, proteolytic cleavage) involved in generating a fertilisation competent spermatozoon [29]. Illustrative of the application of these technologies, mass spectrometry based proteomics has been used to map defects in human spermatozoa associated with failure to participate in ZP interaction. Among a small handful of significant alterations, the molecular chaperone heat shock protein A2 (HSPA2) was identified as being significantly under-represented in the spermatozoa of infertile patients [15]. Such findings accord with independent evidence that the overall levels of HSPA2 present in mature human spermatozoa provide a robust discriminative index of the success of the cumulus–oocyte interactions and reflect fertilising potential [13]. In accounting for these observations, HSPA2 has been implicated in the process of sperm plasma membrane remodelling associated with spermiogenesis and capacitation [15]. Thus, an under-representation of the protein likely comprises the formation of ZP binding domains on the surface of the mature spermatozoon. Moreover, the loss of HSPA2 from the sperm proteome has been causally linked to elevated levels of oxidative stress in various models [32], reinforcing the notion that the efficacy of sperm–oocyte interactions provide a highly sensitive readout of the legacy of this form of physiological insult.
Aside from sperm proteins, mounting interest has focused on alternative macromolecules such as the specific sperm RNAs and/or the overall integrity of the cell’s DNA as predictive markers of fertilisation success [33,34]. Despite their transcriptionally and translationally inert status, it is now apparent that mature sperm cells are furnished with a complex cargo of RNA transcripts, including both mRNA and several species of small non-coding RNAs (sncRNA) [35]. Moreover, a subset of these transcripts has been implicated in regulating the initiation and/or trajectory of embryo development, as well as exerting influence over the lifetime health of the offspring [36]. Thus, far from being inconsequential remnants of the spermatogenic process, the sperm-borne RNA profile is gaining traction as a sensitive prognostic indicator for evaluating sperm quality and male infertility. There is also mounting interest in sperm-borne RNAs as a diagnostic readout of a male’s exposure to reproductive toxicants as well as a surrogate to evaluate the fidelity of the spermatogenic and post-testicular development that gives rise to the mature spermatozoon [33,35]. In this context, compelling evidence now exists linking paternal exposure to a wide range of environmental stressors (e.g. dietary perturbations, corticosterone administration, psychological stress, cigarette smoke and environmental pollutants) to pronounced alterations in the sncRNA profile that the exposed spermatozoa carry. The clinical significance of these data rests with the fact that each of these changes represents a source of potential sperm dysfunction. Accordingly, a growing body of literature indicates that males with idiopathic infertility exhibit a specific sperm sncRNA expression profile that is clearly differentiated from that of fertile individuals [35]. The potential impact of such differences on the individuals’ reproductive competence is highlighted by aberrant embryo miRNA expression detected in human blastocysts derived from patients with male factor infertility [37]. Notwithstanding the interest these studies have generated, the unique biology of a spermatozoon presents a number of challenges in seeking to characterise their RNA cargo for diagnostic purposes. Not least of these are the relatively low yield of RNA per spermatozoon and the absence of 18S and 28S ribosomal RNA subunits with which to rigorously assess the fidelity of sperm RNA isolation protocols. The continued refinement of isolation protocols that reliably yield high-quality sperm RNA will undoubtedly help to circumvent these limitations [38], aiding in the translation of data collected from animal models, as well as enhancing the reproducibility of clinical assessments of male factor fertility using RNA molecular biomarkers. In time, the assembly of a human sperm RNA inventory, equivalent to that already achieved for the proteome, may provide the opportunity to evaluate and monitor the effects of chemical, physical and environmental factors on semen quality.
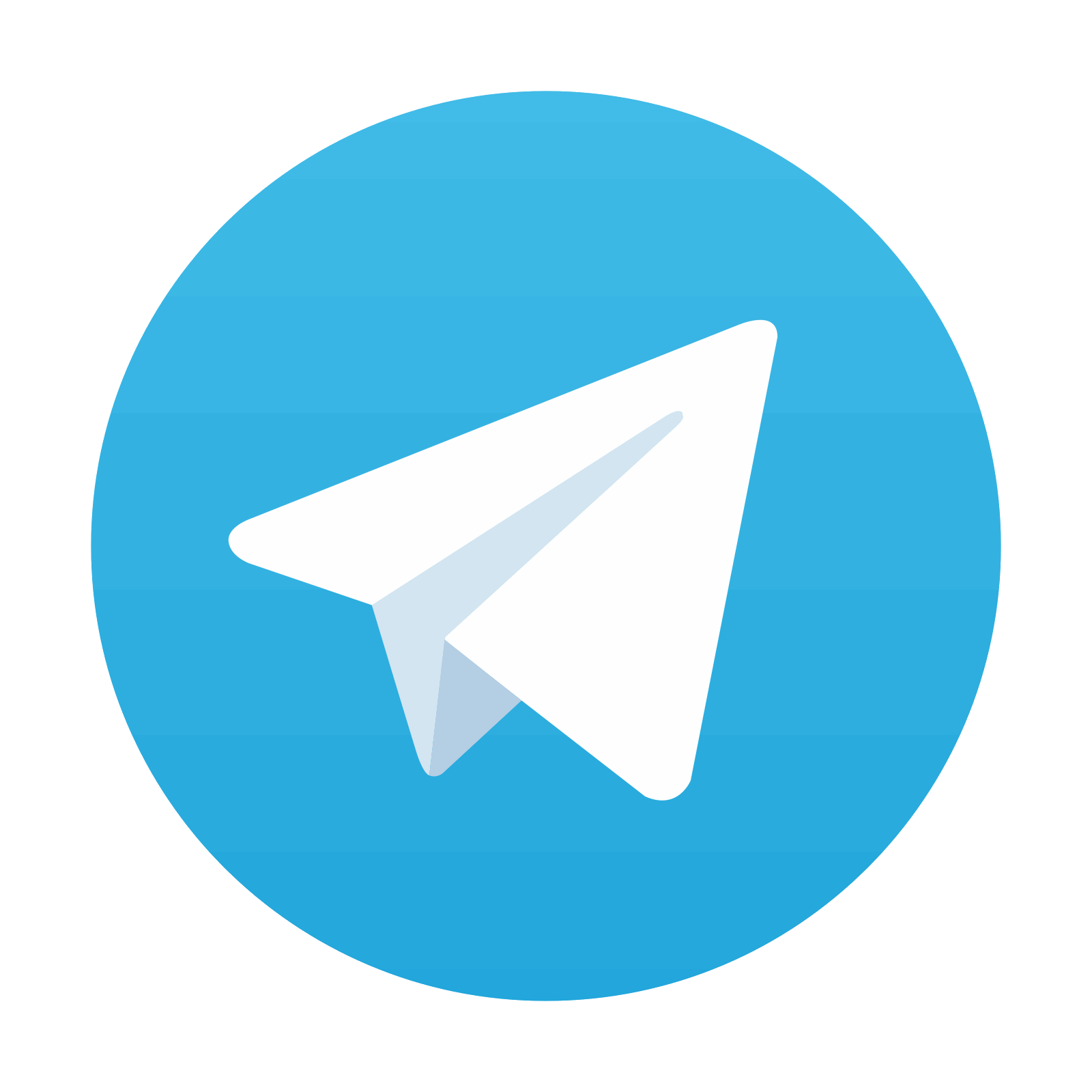
Stay updated, free articles. Join our Telegram channel

Full access? Get Clinical Tree
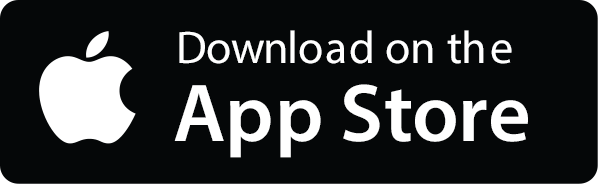
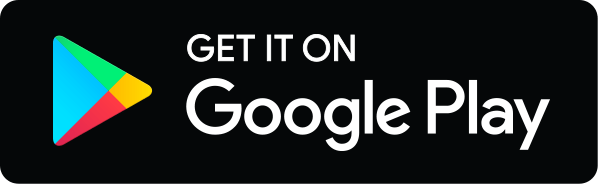
