Abstract
Synchrony is essential for gametogenesis and correct embryo development, and a basic knowledge of reproductive endocrinology is fundamental to understanding synchrony in reproductive physiology. Although sexual arousal, erection and ejaculation in the male are obviously under cerebral control, it is less obvious that the ovarian and testicular cycles are also coordinated by the brain. For many years after the discovery of the gonadotropic hormones follicle-stimulating hormone (FSH) and luteinizing hormone (LH), the anterior pituitary gland was considered to be an autonomous organ, until animal experiments in which lesions were induced in the hypothalamus clearly demonstrated that reproductive processes were mediated by the nervous system. The hypothalamus is a small inconspicuous part of the brain lying between the midbrain and the forebrain; unlike any other region of the brain, it not only receives sensory inputs from almost every other part of the central nervous system (CNS), but also sends nervous impulses to several endocrine glands and to pathways governing the activity of skeletal muscle, the heart and smooth muscle (Figure 2.1). Via a sophisticated network of neural signals and hormone release, the hypothalamus controls sexual cycles, growth, pregnancy, lactation and a wide range of other basic and emotional reactions. Each hypothalamic function is associated with one or more small areas that consist of aggregations of neurons called hypothalamic nuclei. In the context of reproduction, several groups of hypothalamic nuclei are connected to the underlying pituitary gland by neural and vascular connections.
Introduction
Synchrony is essential for gametogenesis and correct embryo development, and a basic knowledge of reproductive endocrinology is fundamental to understanding synchrony in reproductive physiology. Although sexual arousal, erection and ejaculation in the male are obviously under cerebral control, it is less obvious that the ovarian and testicular cycles are also coordinated by the brain. For many years after the discovery of the gonadotropic hormones follicle-stimulating hormone (FSH) and luteinizing hormone (LH), the anterior pituitary gland was considered to be an autonomous organ, until animal experiments in which lesions were induced in the hypothalamus clearly demonstrated that reproductive processes were mediated by the nervous system. The hypothalamus is a small inconspicuous part of the brain lying between the midbrain and the forebrain; unlike any other region of the brain, it not only receives sensory inputs from almost every other part of the central nervous system (CNS), but also sends nervous impulses to several endocrine glands and to pathways governing the activity of skeletal muscle, the heart and smooth muscle (Figure 2.1). Via a sophisticated network of neural signals and hormone release, the hypothalamus controls sexual cycles, growth, pregnancy, lactation and a wide range of other basic and emotional reactions. Each hypothalamic function is associated with one or more small areas that consist of aggregations of neurons called hypothalamic nuclei. In the context of reproduction, several groups of hypothalamic nuclei are connected to the underlying pituitary gland by neural and vascular connections.
Figure 2.1 Hypothalamic–pituitary–gonadal (HPG) axis. Schematic summary of the endocrine control of reproduction in mammals.
Functions of the Hypothalamus in Reproduction
The hypothalamus links the nervous system to the pituitary gland by receiving signals from:
The central nervous system (CNS)
Neurons from other parts of the brain, including the amygdala (involved in emotional response), the visual cortex and the olfactory cortex
Endocrine factors from the testis, ovary and other endocrine glands.
It then releases factors into the hypothalamic–hypophyseal portal veins that stimulate or inhibit synthesis and release of hormones by the pituitary, including (but not only):
Gonadotropin-releasing hormone (GnRH): releases FSH and LH
Thyroid-releasing hormone (TRH): releases thyroid-stimulating hormone (TSH)
Corticotropin-releasing hormone/corticotropin-releasing factor (CRH/CRF): releases adrenocorticotropic hormone (ACTH)
Growth hormone-releasing hormone (GHRH): releases growth hormone (GH).
Gonadotropin-releasing hormone (GnRH) is secreted by groups of hypothalamic nuclei and transported to the anterior pituitary through the portal vessels. GnRH, a decapeptide with the structure (Pyr)-Glu-His-Trp-Ser-Tyr-Gly-Leu-Arg-Pro-Gly-NH2, is the most important mediator of reproduction by the CNS. Any abnormality in its synthesis, storage, release or action will cause partial or complete failure of gonadal function. GnRH is secreted in pulses and binds to specific receptors on the plasma membrane of the gonadotroph cells in the pituitary, triggering the inositol triphosphate second messenger system within these cells. This signal induces the movement of secretory granules toward the plasma membrane and eventually stimulates the pulsatile secretion of LH and FSH. Alterations in the output of LH and FSH may be achieved by changing the amplitude or frequency of GnRH, or by modulating the response of the gonadotroph cells.
The anterior pituitary secretes LH, FSH and TSH, which are heterodimeric glycoprotein molecules that share a common alpha-subunit (also shared by human chorionic gonadotropin [hCG]) and differ by their unique beta-subunit. Only the heterodimers have biological activity. The specific functions of FSH have been studied with targeted deletions in ‘knockout’ mice: FSH-deficient male mice have a decrease in testicular size and epididymal sperm count, but remain fertile. In contrast, homozygous females with the mutation are infertile: their ovaries show normal primordial and primary follicles, with no abnormalities in oocytes, granulosa or theca cells, but the follicles fail to develop beyond the preantral stage. The ovaries also lack corpora lutea, and serum progesterone levels were decreased by 50% compared with normal mice. These studies indicate that, in mice, whereas spermatogenesis can continue in the absence of FSH, follicle maturation beyond the preantral stage is FSH-dependent.
At the onset of puberty, increased activity of the GnRH pulse generator induces maturation of the pituitary–gonadal axis. A progressive increase in the release of gonadotropins from the pituitary stimulates a subsequent rise in gonadal steroid hormones. In males, FSH released in response to the GnRH pulses acts on Sertoli–Leydig cells to initiate spermatogenesis, and LH acts on the Leydig cells to stimulate testosterone production. In females, FSH initiates follicular maturation and estrogen production, and LH stimulates theca cell steroidogenesis and triggers ovulation.
Male Reproductive Endocrinology
The neuroendocrine mechanisms that regulate testicular function are fundamentally similar to those that regulate ovarian activity. The male hypothalamo–pituitary unit is responsible for the secretion of gonadotropins that regulate the endocrine and spermatogenic activities of the testis, and this gonadotropin secretion is subject to feedback regulation. A major difference between male and female reproductive endocrinology is the fact that gamete and steroid hormone production in the male is a continuous process after puberty, and not cyclical as in the female. This is reflected in the absence of a cyclical positive feedback control of gonadotropin release by testicular hormones. The hypothalamus integrates all of these signals and relays a response via the release of the peptide hormone GnRH. The hormone is released in pulses, with peaks every 90–120 minutes, and travels to the anterior pituitary gland, where it stimulates the synthesis and episodic release of gonadotropic hormones to regulate sperm production in the testes. Interestingly and paradoxically, after the pituitary is initially stimulated to produce these gonadotropins, exposure to constant GnRH (or a GnRH agonist) occupies the receptors so that the signaling pathway is disrupted, and gonadotropin release is inhibited.
The process of spermatogenesis is a ‘two-cell’ process, dependent on cross-talk between the Leydig (equivalent to ovarian theca) and Sertoli (equivalent to granulosa) cells via their respective gonadotropin receptors, LH and FSH. Sertoli cells respond to pituitary FSH and secrete androgen-binding proteins (ABPs). Pituitary LH stimulates the interstitial cells of Leydig to produce testosterone, which combines with ABP in the seminiferous tubules, and testosterone controls LH secretion by negative feedback to the hypothalamus, maintaining the high intratesticular testosterone that is appropriate for normal spermatogenesis. Sertoli cells also produce inhibin, which exerts a negative feedback effect on pituitary FSH secretion. It probably also has a minor controlling influence on the secretion of LH.
Although the role of inhibin is less clear in the male than in the female, inhibin-like molecules have also been found in testicular extracts, which presumably also regulate FSH secretion. In humans, failure of spermatogenesis is correlated with elevated serum FSH levels, perhaps through reduced inhibin secretion by the testis. In the testis, LH acts on Leydig cells, and FSH on Sertoli cells; males are very sensitive to changes in activity of LH and are relatively resistant to changes in FSH activity.
1. Follicle-stimulating hormone (FSH): acts on the germinal epithelium to initiate spermatogenesis; receptors are found on Sertoli cells.
Sertoli cells secrete inhibin, which regulates FSH secretion.
2. Luteinizing hormone (LH): stimulates the Leydig (interstitial) cells to produce testosterone.
Gonadotropic stimulation of the testes regulates the release of hormones (androgens) that are required for the development of puberty, and then to initiate and maintain male reproductive function and spermatogenesis. Testosterone is the major secretory product of the testes, responsible for male sexual characteristics such as facial hair growth, distribution of body fat/muscle and other ‘masculine’ features. Testosterone is metabolized in peripheral tissue to the potent androgen dihydrotestosterone, or to the potent estrogen estradiol. These androgens and estrogens act independently to modulate LH secretion. In the testis, the androgen receptor is found on Sertoli cells, Leydig and peritubular myoid cells. Ablating the androgen receptor inhibits spermatogenesis.
Feedback mechanisms are an important part of the reproductive axis; testosterone inhibits LH secretion, while inhibin (secreted by Sertoli cells in the testes) regulates FSH secretion. If negative feedback is reduced, the pituitary responds by increasing its FSH secretion, similar to the situation in women reaching the menopause. Serum FSH levels in the male therefore act as an indicator of testicular germinal epithelial function – i.e., they are broadly correlated with spermatogenesis. Testosterone levels indicate Leydig cell function, and reflect the presence of ‘masculine’ characteristics:
Low levels in boys and castrates (<4 nm/L).
Varies throughout the day in adult males – highest in the morning.
Levels decrease in older men.
Serum LH level is difficult to assess, because it is released in pulses. Prolactin also interacts with LH and FSH in a complex manner, via inhibition of GnRH release from the hypothalamus. In males with hyperprolactinemia, inhibition of GnRH decreases LH secretion and testosterone production; elevated prolactin levels may also have a direct effect on the CNS.
An endocrine profile should be performed in men with oligospermia, or if there are signs or symptoms to suggest androgen deficiency or endocrine disease.
1. Testosterone: normal range is 10–35 nmol/L, but serum levels undergo diurnal variation, with highest levels in the morning. Therefore, the time of the test is important, and borderline levels should be compared with other measurements taken on different days.
2. FSH and LH: normal serum levels of both are <10 IU/L (but normal ranges for specific laboratories should be noted).
Azoospermia with normal T, FSH and LH indicates mechanical obstruction to the passage of sperm.
Elevated FSH indicates germinal cell insufficiency.
Elevated FSH and LH, low T indicates primary testicular failure.
Low FSH, LH and T indicates hypothalamic or pituitary insufficiency.
Female Reproductive Endocrinology
In females, gonadotropin output is regulated by the ovary.
1. Low circulating levels of estradiol exert a negative feedback control on LH and FSH secretion, and high maintained levels of estradiol exert a positive feedback effect.
2. High plasma levels of progesterone enhance the negative feedback effects of estradiol and keep FSH and LH secretion down to a low level.
3. The secretion of FSH, but not LH, is also regulated by nonsteroidal high-molecular-weight (around 30 000 kDa) proteins called inhibins found in follicular fluid: inhibins are found at high levels in late follicular phase plasma of fertile women, and although they have been used as a relative marker of ovarian reserve, the direct correlation is not clear.
In an ovulatory cycle, FSH stimulates the growth of follicles and increases the rate of granulosa cell production, and stimulates the aromatization of androgenic precursors and the appearance of receptors for LH/hCG on granulosa cells. Estrogens enhance the action of LH on the follicle, and also exert a negative feedback effect on the hypothalamus and pituitary to inhibit further release of gonadotropins. The levels of estrogen produced by the granulosa cells increase throughout the follicular phase until a threshold is reached where a separate positive feedback mechanism elicits the LH surge and overrides the negative feedback on gonadotropin secretion; this is the trigger for ovulation.
Inhibin is also secreted by the ovary and reduces pituitary FSH secretion.
LH stimulates theca cells to secrete androgens, which are converted to estrogens by the granulosa cells, and also influences granulosa cell differentiation, i.e., limits their replication and causes luteinization. LH stimulates the formation of progesterone directly from cholesterol in both granulosa and theca cells, and after ovulation the follicle changes rapidly from estrogen to progesterone dominance within a short period of time. Two common genetic variants in the LH beta-chain have been described that affect the molecule’s biological properties (Lamminen and Huhtaniemi, 2001), with potential effects on ovarian steroidogenesis as well as response to hormonal stimulation.
FSH Receptors
FSH acts by binding to a specific receptor in the granulosa cell plasma membrane (FSHR), and normal function of FSHR is crucial for female fertility: FSHR knockout mice are infertile. Binding leads to the elevation of intracellular cAMP through a G-protein-coupled signal transduction mechanism that activates adenylate cyclase (Simoni et al., 2000).
FSHR Polymorphism
The FSHR gene has been localized to chromosome 2p21 and spans 54 kbp. Although naturally occurring mutations in this gene are rare, at least three common single-nucleotide polymorphisms (SNPs) have been found in the receptor: one in its promoter, and two on exon 10. FSH receptor mRNA also undergoes extensive alternative splicing, potentially giving rise to a number of different isoforms. The FSHR genotype is important in determining the action of FSH, and SNPs, either single or in various combinations, can modify endocrine feedback systems and the effects of other hormones. Thus, polymorphism in the FSHR gene results in a variable response to FSH among individuals, ranging from normal function to infertility, and these variations can affect how the ovary responds to FSH stimulation (Perez-Mayorga et al., 2000): follicles cannot grow and mature when FSH action is disrupted. Reduced expression of FSHR on granulosa cells is associated with poor ovarian response (Cai et al., 2007), and women with an FSHR polymorphism may require larger doses of FSH during controlled ovarian stimulation (COS) to achieve an adequate response during ART.
Two-Cell Two-Gonadotropin Theory
The ‘two-cell two-gonadotropin’ theory suggests that both FSH and LH are required for estrogen biosynthesis, and this requires the cooperation of both theca and granulosa cells, with coordinated expression of a cytochrome P450 enzyme triad: cholesterol side-chain cleavage cytochrome P450, 17-alpha hydroxylase cytochrome P450 and aromatase cytochrome P450.
1. The surface of theca interna cells expresses LH receptors, and LH binding to these receptors initiates a G-protein-cell signaling pathway that leads to production of cAMP. cAMP in turn stimulates the synthesis of androgens from acetate and cholesterol precursors.
2. Androstenedione is released from the thecal cell and enters the mural granulosa cells, where P450 aromatase converts it to estradiol (E2), which is released into the follicular fluid and the bloodstream. This reaction is enhanced by FSH.
The secreted estradiol is required for follicle maturation and subsequent follicle survival, and these follicles are referred to as ‘gonadotropin-dependent,’ since they require both LH and FSH in order to carry out their steroid biosynthesis (Figure 2.2).
Anti-Müllerian Hormone (AMH)
AMH is a glycoprotein composed of two identical monomers linked by disulfide bridges, and the hormone circulates as a dimer. It is a member of the transforming growth factor-beta (TGF-beta) superfamily of molecules involved in tissue growth and differentiation. AMH was first identified as a key factor in male sex differentiation, and owes its name to the role it plays in this process (see Chapter 3). At 7–8 weeks’ gestation, Sertoli cells in the fetal testis secrete AMH, which induces regression of the Müllerian ducts. In the early female fetus, the absence of AMH allows these ducts to develop into uterus, fallopian tubes and the upper part of the vagina.
In women, AMH is produced by granulosa cells from growing preantral and antral follicles. It is secreted into the blood and therefore can be measured in serum. Levels are almost undetectable in female babies, and they then gradually increase during the first 2 to 4 years of life, after which they remain stable until onset of puberty. Serum AMH declines gradually through reproductive life as the follicular reserve is depleted, and it is undetectable after menopause. Serum AMH levels reflect the follicular ovarian pool: a reduction in the number of small growing follicles is followed by a reduction in circulating AMH, and this strong correlation may be used to predict the onset of menopause. If the ovaries are surgically removed, AMH is undetectable within 3–5 days, indicating that it is produced only by cells in the ovary (La Marca et al., 2005). This also makes it a useful biomarker in post-ovariectomy follow-up of ovarian cancer patients: granulosa cell tumors have a high incidence of recurrence, and AMH monitoring allows their early detection.
Experiments in the rat show that AMH alters the expression of several hundred genes regulating cellular pathways that inhibit the development of primordial follicles; early stages of follicular development are inhibited via a transmembrane serine/threonine kinase type II receptor signaling pathway (Nilsson et al., 2007).
During the menstrual cycle, the granulosa cells of the growing antral follicles secrete AMH under the influence of pituitary FSH. When a follicle reaches the size and stage of differentiation that determines its dominance prior to ovulation, AMH is no longer expressed (Weenen et al., 2004). In the human, this occurs in antral follicles of 4–6 mm; growing follicles are more sensitive to FSH in the absence of AMH, and the transition from primordial into growing follicles is enhanced in its absence (Durlinger et al., 2001; Visser & Themmen, 2005). It is not expressed in atretic follicles or in theca cells. Polymorphisms in the AMH gene or in its receptor seem to be related to follicular fluid and follicular phase serum estradiol levels, suggesting that it may also have a role in FSH-induced steroidogenesis in the ovary (Kevenaar et al., 2007).
Several studies have investigated variation in serum AMH levels throughout the menstrual cycle. For clinical purposes, the variation between and within cycles appears to be sufficiently low so that basal measurements can be carried out at any time in the cycle (La Marca et al, 2006, 2010). Serum levels are not affected by pregnancy, GnRH agonist administration or short-term oral contraceptive treatment. During COS with gonadotropins, AMH levels in follicular fluid have been found to be threefold higher in small than in large follicles (Fanchin et al., 2005), confirming that AMH production by granulosa cells declines during final follicular maturation, as follicles grow to a stage where AMH is no longer expressed (La Marca et al., 2004).
AMH as a Biomarker of Ovarian Function
The correlation between circulating AMH levels and the number of early antral follicles has led to its measurement as a biomarker of ovarian function in assessing functional ovarian reserve. Very low or undetectable serum levels are found in women with early ovarian aging and premature ovarian failure, and AMH is now used routinely as a basal measurement prior to COS for assisted reproduction. Studies indicate that serum AMH is more useful in predicting ovarian response than the age of the patient or serum Day 3 FSH, estradiol and inhibin B. One of its advantages over the other hormonal markers is that it can be measured at any time of the cycle, since levels seem to have very low inter- and intracycle variation. Levels decrease during FSH stimulation, and therefore assessment must be carried out before starting FSH treatment. Ovarian reserve as measured by AMH level does not appear to be related to the probability of achieving a pregnancy (Steiner et al., 2017).
Polycystic Ovary Syndrome and Ovarian Hyperstimulation Syndrome
Women with polycystic ovary syndrome (PCOS) characteristically have increased development of antral follicles. Histologically, the number of primordial follicles in PCO ovaries is normal compared to that in non-PCO ovaries, but the number of developing follicles is at least doubled (Webber et al., 2003). Circulating AMH levels in women with PCOS are significantly higher than in healthy controls, and this can be used as a diagnostic marker for PCO. Levels are even higher in women with insulin-resistant PCOS and may correlate with the severity of the syndrome. Significantly elevated basal AMH levels are associated with a risk of hyper-response to gonadotropins and ovarian hyperstimulation syndrome (OHSS): treatment can be individualized in order to avoid OHSS by modifying FSH dosage during ART (Pigny et al., 2006). Women with high AMH levels should be informed of their risk of developing OHSS.
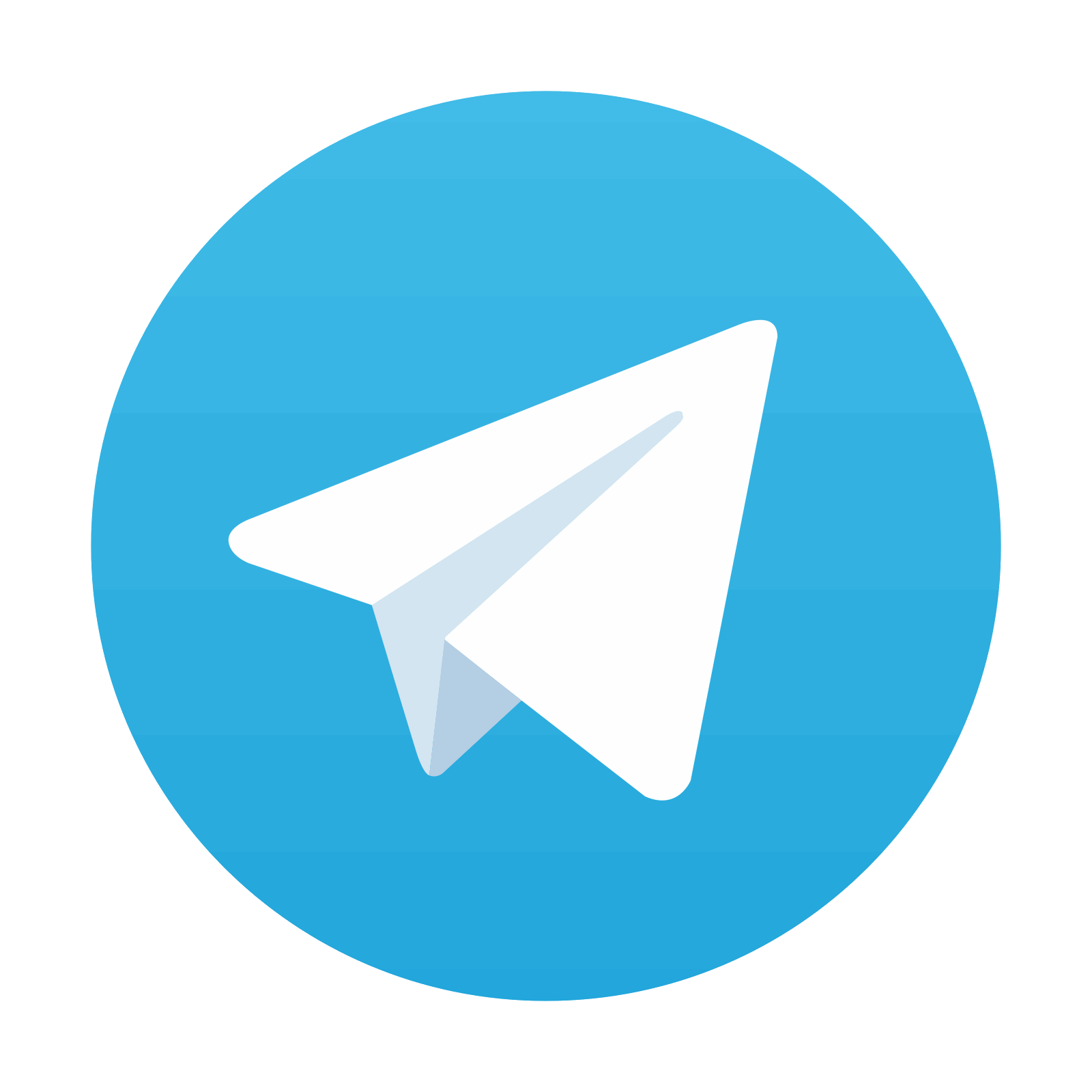
Stay updated, free articles. Join our Telegram channel

Full access? Get Clinical Tree
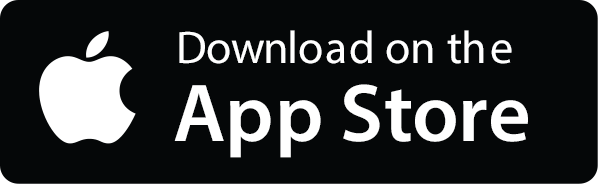
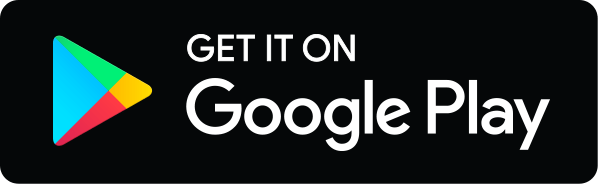
