Abstract
The electrical impulse in the heart is generated by the sinoatrial (SA) node, which is in the right atrium at the entry of the superior vena cava.
1 The Cardiovascular System
1.1 The Conduction System of the Heart
-
The electrical impulse in the heart is generated by the sinoatrial (SA) node, which is in the right atrium at the entry of the superior vena cava.
-
The impulse is then transmitted across both the atria, resulting in atrial contraction.
-
Once the impulse arrives at the atrioventricular node, it is stored for a few seconds to allow ventricular filling.
-
The His-Purkinje system is a specialised conduction tissue, which divides into a left and right branch to innervate both the ventricles.
-
The right bundle is narrow while the left bundle is wide and innervates the muscles of the larger left ventricle. The left bundle block leads to abnormal left ventricular function.
-
Aberrant pathways of conduction can develop, which causes arrhythmias: an example is the Wolff–Parkinson–White (WPW) syndrome.
-
Neurogenic control of the SA node is via sympathetic and parasympathetic nervous system.
-
At rest, the dominant tone is parasympathetic/vagal.
-
There are several factors that affect the heart rate. See Table 16.1.
-
In pregnancy, the two atriums enlarge most: the left atrium increases by 5 mm and the right atrium by 7 mm.
Factors that increase SA node discharge and heart rate | Factors that decrease SA node discharge and heart rate |
---|---|
Thyroxine | Hypothyroidism |
High temperature | Hypothermia |
Beta-adrenergic activity | Beta-adrenergic blockers |
Atropine | Ischaemia (pacemakers are used) |
1.2 Electrocardiogram Changes
In a normal electrocardiogram (ECG):
-
P wave = atrial depolarisation = atrial contraction
-
QRS complex = ventricular depolarisation = ventricular contraction
-
T wave = ventricular repolarisation
-
atrial repolarisation coincides with ventricular depolarisation; hence, is not detected on ECG
-
the speed of the ECG paper is 25 mm/s, so each small square is 0.04 s and each large square represents 0.2 s
-
in the vertical axis, each cm equals 1 Mv
-
prolongation of the PR interval occurs in first-degree blocks when the conduction of electrical impulse between the atrium and ventricle is delayed
-
the PR interval is reduced in faster pathways and in aberrant conduction; a typical example is WPW syndrome where a delta wave occurs
-
normal QRS width is 0.12 s:
-
◦ any longer QRS width is suggestive of a delay in travel in the His-Purkinje system; a typical example is a bundle branch block
-
-
the QT interval is usually 0.30–0.45 s and depends on the heart rate
-
the QT interval is increased in hypocalcaemia, hypokalaemia and rheumatic carditis and with many drugs.
-
the QT interval is decreased in hypercalcaemia, hyperkalaemia and digoxin.
Changes in ECG during pregnancy include:
-
left ventricular hypertrophy and dilatation
-
upwards displacement of the diaphragm
-
the apex is shifted anterior and left
-
heart rate increases by 10–15%
-
left axis deviates by 15 degrees
-
inverted T wave in lead III
-
Q wave in lead III and augmented vector foot (AVF)
-
nonspecific ST changes
1.3 Haemodynamic and Cardiac Events in Pregnancy
See Table 16.2 and Table 16.3.
-
Plasma volume increases from 2600 ml to 3800 ml. This occurs early in pregnancy (6–8 weeks). No further increase occurs after 32 weeks.
-
Red cell mass increases from 1400 ml to 1800 ml with a steady increase until term. This is proportionately lower than the plasma volume increase; hence, the haematocrit and haemoglobin concentration falls.
-
Cardiac output increases by more than 40% (4.5–6 L/min); this occurs early in pregnancy and plateaus at 24–30 weeks. It falls to prepregnancy level after delivery (variable times).
-
Heart rate increases by 10% from 80 beats per minute (BPM) to 90 BPM (late in pregnancy).
-
Stroke volume increases early in pregnancy.
-
Supine hypotension syndrome: this occurs due to pressure of the gravid uterus on the inferior vena cava reducing the cardiac output.
-
Alteration in regional flow occurs with preferential redistribution of blood to the:
-
◦ uterus
-
◦ kidney
-
◦ skin
-
◦ breasts
-
◦ skeletal muscle
-
-
The arteriovenous oxygen gradient falls.
-
At term, the distribution of the 1.5 L of extra blood is as follows:
-
◦ uterus 400 ml/min
-
◦ kidney 300 ml/min
-
◦ skin 500 ml/min
-
◦ gastrointestinal system, breasts and others: 300 ml/min
-
-
Early in pregnancy, extra blood supply is to the skin and breasts.
-
Blood pressure (BP) changes in pregnancy: peripheral vascular resistance falls from 8 to 36 weeks
-
◦ systolic BP falls by 5 mmHg
-
◦ diastolic BP falls by 10 mmHg
-
-
Other factors like position, uterine contractions and drugs affect blood pressure.
-
Blood enters the right heart via the superior and inferior vena cava.
-
Blood from the head via the superior vena cava has less oxygen as the oxygen consumption of the brain is high.
-
The blood that reaches the right atrium is mixed and the mixed venous oxygen concentration in the right atrium is around 60%.
-
The oxygen concentration in the left atrium is about 96%.
-
The left atrium and ventricle have a similar oxygen concentration.
-
True mixed venous blood is best sampled from the pulmonary artery.
-
The fixed reference point for the pressures in the heart is the level of the right atrium. See Table 16.4.
Table 16.2 Changes in the cardiovascular parameters
Stroke volume | +30% |
---|---|
Heart rate | +10–15% |
Systemic vascular resistance | −5% |
Systolic blood pressure | −10 mmHg |
Diastolic blood pressure | −15 mmHg |
Mean blood pressure | −15 mmHg |
Oxygen consumption | +20% |
Table 16.3 Percentage changes in cardiovascular function during pregnancy
Blood volume | +30% |
Plasma volume | +40% |
Red blood cell volume | +20% |
|
|
1.4 Blood Pressure Control in Pregnancy
-
Blood pressure = cardiac output x peripheral resistance.
-
Cardiac output = stroke volume x heart rate.
-
Peripheral resistance is controlled by the autonomic nervous system.
-
Peripheral resistance is also affected by drugs and chemical substances like angiotensin II, serotonin, kinins, catecholamines, adenosine, potassium, hydrogen ion, partial pressure of carbon dioxide (pCO2), partial pressure of oxygen (pO2) and prostaglandins.
-
Blood viscosity will rise hugely when the haematocrit rises above 45%.
-
The receptors involved in blood-pressure control are cholinergic, alpha and beta-adrenergic receptors.
-
Adrenergic stimulation causes vasoconstriction, and vasodilatation is mainly due to a reduction in vasoconstrictor tone.
-
The autonomic system controls blood pressure mainly by the cardioinhibitory and the vasomotor centres.
-
The cardioinhibitory centre is the dorsal motor nucleus of the vagus nerve.
-
The baroreceptors send impulses to the cardioinhibitory centre and cause slowing of the heart rate and a drop in blood pressure.
-
Sympathetic output to the heart and blood vessels is controlled by the vasomotor centre. Input impulses are from baroreceptors and chemoreceptors.
-
Fall in pO2 and pH, or rise in pCO2 stimulates vasomotor centre and increases blood pressure.
-
Rise in cerebrospinal fluid (CSF) pressure will also stimulate vasomotor centre causing a rise in blood pressure (Cushing reflex).
-
Tissue metabolites accumulated following anaerobic metabolism can cause vasodilatation.
-
Autoregulation takes place at a local level with vasodilatation and improved blood flow.
-
Prostaglandins can cause blood vessels to constrict or dilate.
-
Prostaglandin A and prostaglandin E cause a fall in blood pressure by reducing splanchnic vascular resistance, while prostaglandin F causes uterine contraction and bronchial constriction.
-
Prostacyclin causes vasodilatation while thromboxane causes vasoconstriction. See Table 16.5.
Table 16.5 Vasodilators and vasoconstrictors
Substances causing vasodilatation | Substances causing vasoconstriction |
---|---|
Endothelium derived relaxing factor | Angiotensin II |
Prostacyclin | Thromboxane |
Prostaglandin A and E | Prostaglandin F2-alpha |
2 The Respiratory system
2.1 Respiratory Changes in Pregnancy
2.1.1 Lung Volumes
-
Tidal volume: the lung volume that represents the normal volume of air displaced between normal inhalation and exhalation when extra effort is not applied. In a healthy, young human adult, tidal volume is approximately 500 mL per inspiration or 7 ml/kg of body mass.
-
Total lung capacity is 5 L. Of this, 1.5 L remains at the end of a forced expiration called residual volume (RV).
-
Vital capacity: the air that can be inhaled after a forced expiration to a forced inspiration. This is the total of tidal volume, inspiratory reserve volume and expiratory reserve volume.
-
Functional residual capacity (FRC): the volume of air present in the lungs at the end of passive expiration. At the end of a normal expiration, there are opposing effects of the elastic recoil forces of the lung and chest wall, which balance it, and there is no exertion on the diaphragm. However, in chronic obstructive pulmonary disease (COPD), there is a loss of elastic recoil and an increased FRC. Additionally, in chronic emphysema or other airway obstructions, the FRC increases.
-
In pregnancy, due to the pressure of the gravid uterus which moves the diaphragm up, there is reduced FRC and reduced expiratory residual capacity (ERV).
-
ERV: the additional amount of air that can be expired from the lungs by determined effort after normal expiration: FRC = RV + ERV.
The main changes in pregnancy are as follows (see Figure 16.1):
-
Elevation of the diaphragm and altered chest configuration: there is a 5 cm upwards displacement in the resting position of the diaphragm. The chest height reduces, but the rib cage expands outwards hence the total lung capacity remains the same or decreases by 200 ml. Chest compliance reduces, especially in lithotomy. Lung compliance is unaffected.
-
Negative pleural pressure increases, and earlier closure of the small airways causes the reduction in FRC and ERV. This progressively decreases by 20% at term.
-
Increased progesterone directly stimulates the central respiratory centre and causes increased minute ventilation. Ventilation increases by 40% (from the first trimester). This is thought to be due to progesterone, which stimulates the respiratory centre both directly and indirectly. A similar increase in ventilation is also seen in women on progesterone-only pills and in the luteal phase of the menstrual cycle.
-
Breathing is more diaphragmatic than thoracic.
-
Seventy percent of pregnant women experience subjective dyspnoea.
-
Increased risk of pulmonary embolism.
-
Respiratory rate does not change.
-
Increase in tidal volume.
-
Oxygen consumption and carbon dioxide production increases by 20–30% by the third trimester and up to 100% during labour, necessitating increased minute ventilation to maintain normal acid-base status. Oxygen consumption increases (50 ml/min at term):
-
◦ fetus 20 ml/min
-
◦ cardiac output 6 ml/min
-
◦ renal system 6 ml/min
-
◦ overall metabolic rate 18 ml/min
-
-
Respiratory resistance increases: this is measured clinically by the forced expiratory volume in 1 second (FEV1). This is directly dependent on the vital capacity and is expressed as FEV1/forced vital capacity (FVC). The normal ratio is >75%. This ratio falls with age, asthma and lung disease. The resistance is also measured by peak flow rate, which normally is >600 L/min. There is no change in FEV1, or peak flow rate during pregnancy.
2.1.2 Acid-base Balance in Pregnancy
-
The increase in ventilation is greater than the increase in oxygen consumption.
-
Partial pressure of oxygen (pCO2) decreases to 31 mmHg (due to hyperventilation).
-
pO2 increases to 14 kPa during the third trimester and then falls to <13.5 kPa at term (increased cardiac output (CO) is unable to compensate for increased oxygen consumption).
-
Bicarbonate (HCO3) decreases to maintain a normal pH.
-
Sodium (Na) decreases.
-
Osmolarity decreases by 10 mmol/L.
-
The net effect is a mild chronic respiratory alkalosis with a decrease in the arterial pCO2, a slight increase in the pO2 (alveolar gas equation), a slightly elevated pH and a slightly decreased HCO3 (renal compensation).
2.1.3 Summary of Changes in the Respiratory System During Pregnancy
-
tidal volume increases, but not the respiratory rate
-
no change in vital capacity
-
residual volume decreases by 200 ml
-
expiratory reserve volume decreases
-
inspiratory reserve volume decreases early and increases late in pregnancy
-
mild chronic respiratory alkalosis
2.1.4 Composition of Inhaled and Exhaled Air
-
The partial pressures and the composition of dry air, inspired air, alveolar air and expired air differ.
-
In all cases, the relative concentration of gases is nitrogen > oxygen > water vapour > carbon dioxide. The amount of water vapour present in alveolar air (47 mmHg) is greater than that in atmospheric air (5.7 mmHg).
-
Total volume of alveolar air is 2 L. Alveolar ventilation is 350 ml for each breath.
-
Gas exchange occurs at two sites in the body: in the lungs, where oxygen is picked up and carbon dioxide is released at the respiratory membrane, and at the tissues, where oxygen is released and carbon dioxide is picked up.
-
External respiration is the exchange of gases with the external environment, and occurs in the alveoli of the lungs. Internal respiration is the exchange of gases with the internal environment, and occurs in the tissues. The actual exchange of gases occurs due to simple diffusion.
-
Ventilation is the process that moves air into and out of the alveoli. Perfusion affects the flow of blood in the capillaries. Both are important in gas exchange, as ventilation must be sufficient to create a high pO2 in the alveoli. If ventilation is insufficient and the pO2 drops in the alveolar air, the capillary is constricted and blood flow is redirected to alveoli with sufficient ventilation.
2.1.5 Control of Respiration
-
The respiratory centre is responsible for controlling the depth and rhythmicity of the respiration.
-
The respiratory centre is under the influence of chemoreceptors:
-
◦ central chemoreceptors
-
◦ peripheral chemoreceptors around the aortic arch and in the carotid body
-
-
The aortic arch chemoreceptors are innervated by the vagus nerve and the carotid body chemoreceptors by the glossopharyngeal nerve.
-
Carotid body receptors are sensitive to pO2.
-
Both carotid and aortic body receptors are sensitive to changes of pH and pCO2.
2.2 Applied Respiratory Physiology
-
In pregnancy, spirometry remains normal. This includes FVC, FEV1 and peak expiratory flow rate, which remain unaltered. However, lung volumes change.
-
Asthma: corticosteroids should be used promptly to prevent critical illness.
-
COPD: there is a risk of hypoxia and hypercapnia in pregnancy. It leads to severely reduced vital capacity (VC).
-
Pulmonary oedema: this can occur with use of tocolytics or in preeclampsia with the use of magnesium sulphate.
2.2.1 Acute Respiratory Distress Syndrome
-
Nonobstetric causes of acute respiratory distress syndrome (ARDS) include sepsis, pneumonia, intracerebral haemorrhage, blood transfusion reaction and reduced gastrointestinal sphincter tone.
-
Obstetric causes include amniotic fluid embolism, preeclampsia, septic miscarriages, retained placenta and tocolysis.
-
Treatment includes prompt antibiotics, intravenous hydration, mechanical ventilation and extracorporeal life support in refractory ARDS.
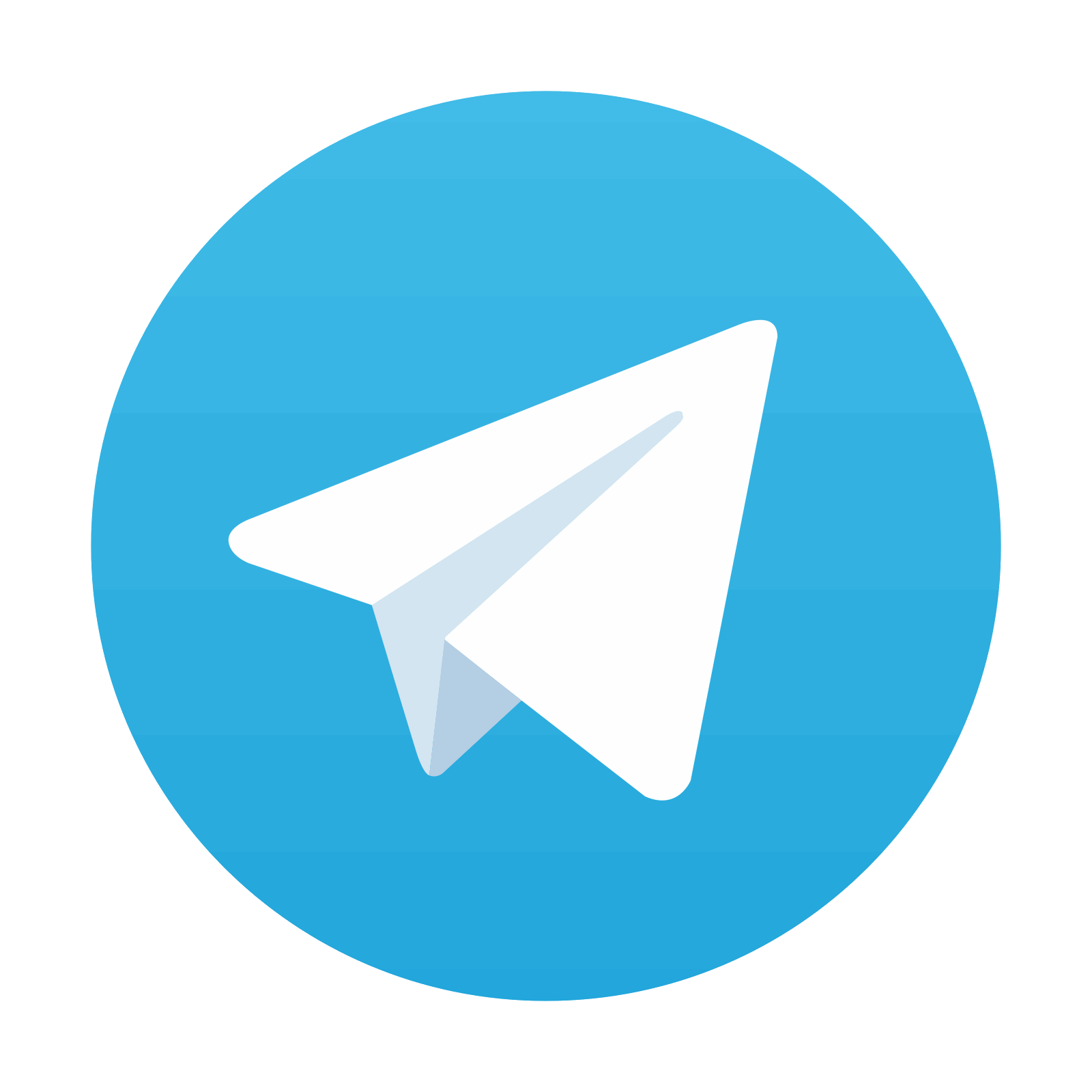
Stay updated, free articles. Join our Telegram channel

Full access? Get Clinical Tree
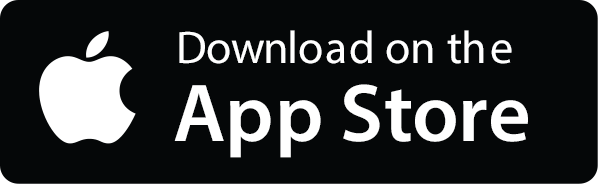
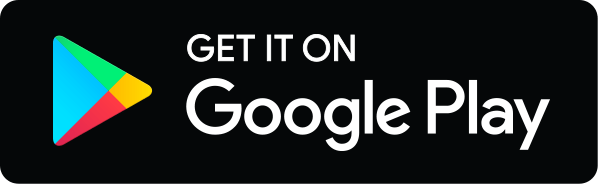