Abstract
Over the past three decades survival rate in children and adolescents with cancer has doubled as a result of significant improvements in medical treatments [1]. Since more than 80% of children and adolescents diagnosed with cancer will survive ≥5 years beyond their diagnosis [2], one of the important concerns are the adverse effects of chemo- and radiotherapy on gonadal hormonal function and spermatogenesis [1]. Moreover, 80% of cancer survivors are intending to father their genetically own children, with only 10% acceptance of using donor sperm [3]. This scenario renders fertility preservation a pivotal aspect for the treatment of cancer in prepubertal boys and adolescents.
Spermatogonia are extremely susceptible to damage induced by chemo- or radiotherapy [4], and the extent of insult depends on the regimen and the cumulative dosage of treatments used [2]. Consequently, restoration of fertility following childhood cancer treatment will be dependent on the survival of sufficient numbers of spermatogonial stem cells (SSCs). In primates these can be recognized as the type Adark subtype which acts as regenerative reserve [4].
Introduction
Over the past three decades survival rate in children and adolescents with cancer has doubled as a result of significant improvements in medical treatments [1]. Since more than 80% of children and adolescents diagnosed with cancer will survive ≥5 years beyond their diagnosis [2], one of the important concerns are the adverse effects of chemo- and radiotherapy on gonadal hormonal function and spermatogenesis [1]. Moreover, 80% of cancer survivors are intending to father their genetically own children, with only 10% acceptance of using donor sperm [3]. This scenario renders fertility preservation a pivotal aspect for the treatment of cancer in prepubertal boys and adolescents.
Spermatogonia are extremely susceptible to damage induced by chemo- or radiotherapy [4], and the extent of insult depends on the regimen and the cumulative dosage of treatments used [2]. Consequently, restoration of fertility following childhood cancer treatment will be dependent on the survival of sufficient numbers of spermatogonial stem cells (SSCs). In primates these can be recognized as the type Adark subtype which acts as regenerative reserve [4]. Following gonadotoxic insult SSCs will become mitotically active and transform into differentiating spermatogonia (type Apale) that act as self-renewing progenitor population [5]. Although spermatogenesis initiates at puberty, there is a constant turnover of spermatogonia in prepubertal testes. These cells undergo apoptosis early during differentiation [4, 5]. This may explain why the prepubertal state does not offer protection against deleterious effects of chemo and/or radiotherapy [2]. While low-dose chemo- or radiotherapy will cause temporary damage by depleting and subsequent replenishment of Apale spermatogonia, high-dose treatment can lead to complete depletion of all spermatogonia, rendering the patients permanently infertile [4]. Furthermore, the direct effects of anticancer therapy on the supporting somatic cell environment are poorly understood in prepubertal testes [6, 7].
Indications for Fertility Preservation in Prepubertal Boys and Adolescents – a Need for Strategic Planning
Evaluating the risk for the degree of anticipated infertility appears crucial for decision on the most appropriate fertility protection strategy. Due to high individual variation toward the effect of the gonadotoxic exposure and the underlying disease, it is difficult to predict which patients would benefit from the fertility-sparing service [8]. Infertility risk assessment should account for both intrinsic and extrinsic factors (reviewed in [9]), and when combined patients can be assigned into groups of high, medium, or low risk [9, 10]. Extrinsic factors focus on treatment modality, which depends on type and stage of disease. Intrinsic factors related to patients’ health status will define their susceptibility to the specific therapy. Primarily, the pubertal status reflecting the testicular developmental stage and the degree of spermatogenic induction are relevant intrinsic factors when assessing the expected degree of treatment-induced gonadal damage. Currently, there are at least seven academic centers in Europe offering fertility preservation programs for prepubertal boys and adolescents [2, 62]. These treatments are at present performed as experimental procedures under institutional review board approval. The inclusion criteria for testicular tissue cryobanking differ between centers (Table 13.1). Only Belgium has recently declared testicular tissue retrieval and cryobanking as a standardized procedure. It was also decided to cover the costs for testicular tissue retrieval and cryobanking in boys and adolescents in approved clinical settings. Legal frameworks differ between European countries and may undergo significant changes in coming years.
Table 13.1 Survey results of selection criteria and practices for testicular tissue cryobanking for fertility preservation in boys and adolescents across European academic centers
Country | Service started | Inclusion criteria | Age range (years) | Number of patients with collected and stored samples | Cryopreservation protocol | Further notes | Source of information |
---|---|---|---|---|---|---|---|
Sweden, Finland and Iceland (NORDFERTIL program) | 2014 | Very high risk of infertility; testicular volume <10 ml | 0.7–13.1 | 37 | Keros et al. [11] |
| Report from questionnaire; Stukenborg et al. [12] |
United Kingdom (Edinburgh and Oxford Fertility Preservation program) | 2016 | High risk of infertility (>80%); unable to produce sample by masturbation; no clinically significant pre-existing testicular disease; informed consent (parent and, when possible, patient) negative HIV, syphilis, and hepatitis serology | 0–16 | 151 | Wyns et al. [13] Keros et al. [11] | NA | Report from questionnaire; Anderson et al. [9] |
Netherlands (Amsterdam UMC locatie AMC; Radboud UMC) | 2011 | As indicated in Skinner et al. [7] | 0–16 | 98 | Keros et al. [11] | No previous anticancer therapy; 15% of sample biopsy used for research after separate informed consent | Report from questionnaire |
Germany (Androprotect program, Muenster) | 2010 | Oncological and nonmalignant conditions (e.g., thalassemia, sickle cell disease, cryptorchidism, Klinefelter’s syndromea) prior and after to polychemotherapy/radiotherapy or conditioning chemotherapy for bone marrow transplantation; unable to produce sample by masturbation or there is no chance to find sperm in mTESE | 0.5–17 (up to 20 for Klinefelter’s syndrome patients) | 75 |
|
| Report from questionnaire; Heckmann et al. [8] |
Belgium (University Hospital, UZ Brussels) | 2002 | Malignant and nonmalignant diseases (incl. Klinefelter’s syndromea) with high risk of infertility (>80%) induced by treatment |
| 115 | Baert et al. [14] |
| Report from questionnaire |
Belgium (Cliniques Universitaires Saint-Luc, Louvain) | 2005 | No gonadotoxic pre-treatment; low, medium and high risk of infertility (malignant diseases and conditioning chemotherapy for bone marrow transplantation) | 0–16 | 62 (May 2005-June 2010) | Wyns et al. [15] | <5% of testicular volume biopsied; patients with; some patients had previous urogenital history (e.g., epispadias, circumcision, orchidopexy) | Wyns et al. [16] |
a Fertility cryopreservation service is no longer provided or is to be terminated for Klinefelter’s syndrome patients due to questionable benefit for the patients [17]. NA – not applicable; mTESE – microdissection testicular sperm extraction; LN2 – liquid nitrogen.
Patients Assigned to Gonadotoxic Treatment
Since the surgical recovery of testicular tissue is an invasive procedure, inclusion criteria for testicular tissue cryobanking should be restricted to patients at significant risk of treatment-induced testicular damage and subsequent infertility. These include total body irradiation and chemotherapy conditioning before allogeneic or autologous hematopoietic stem cell transplantation (HSCT), radiation localized to the testis or treatment with high dose alkylating agents [7, 9]. However, only a small proportion of patients with the most common hematological malignancies in boys and adolescents (i.e., acute leukemia and Hodgkin’s lymphoma, respectively) will receive conditioning therapy for HSCT [2, 10]. As conventional chemotherapy for these conditions is associated with low risk of infertility, most patients with haematological malignancies do not meet the criteria for testicular tissue cryopreservation at the time of initial diagnosis [18]. Nevertheless, treatment failure or relapse may result in reclassification of these patients to high risk of subfertility group following assignment of sterilizing therapy regimens [18]. Evaluation of spermatogonial quantity per tubular cross section (S/T) and tubular fertility index (% tubular cross-sections containing spermatogonia) in testicular biopsies retrieved for cryobanking can be predictive of spermatogenic recovery and thus help in refining infertility risk assessment [19]. It will also allow to account for the effects of the disease per se and of any anticancer treatment administered before biopsy recovery. Two recent studies looked at these parameters in biopsies from prepubertal patients with malignant and nonmalignant conditions who received chemotherapy or were not treated prior biopsy [12, 20]. Spermatogonial quantity was significantly compromised in patients treated with alkylating agents, even in low doses, compared to normative reference values and values for patients, treated with non-alkylating agents. Surprisingly, similar spermatogonial depletion was observed in patients with nonmalignant diseases (e.g., sickle cell disease) treated with hydroxyurea [12]. Anticancer drugs are administered as multidrug regimens. The relative contribution of every individual drug is difficult to ascertain and the combined treatment may have cumulative damaging effects [7]. Therefore, ex vivo animal studies (tissue fragments culture and grafting experiments) using clinically relevant doses and regimens of anticancer treatments can be helpful in determining fertility potential and predicting spermatogenesis recovery [21, 22].
In light of emerging evidence, patient selection criteria should be revisited. To provide better parameters for classification leading to a more accurate fertility restoration prognosis may reclassify patients from low/intermediate risk to high risk group.
Male Genetic Disorders Causing Testicular Degeneration
Fertility preservation could potentially be considered for Klinefelter’s syndrome (KS) patients, since this sex chromosomal disorder causes germ cell depletion during development and hence, azoospermia in >90% cases [23]. Although prepubertal KS patients were initially included in the fertility preservation programs of some European centers ([2]; Table 13.1), compiling evidence has led to a change in clinicians’ decision. Many centers have stopped offering invasive fertility preservation service for KS patients prior to puberty (reviewed in [23]). Since significant germ cell depletion is suggested to occur mainly during prepuberty [8, 17], testicular biopsy for cryobanking could compromise the future endocrine and spermatogenic function of KS testes [17, 23].
Immature Testicular Cell Retrieval and Tissue Cryopreservation
Currently, sperm cryobanking is the only clinically validated technique available for safeguarding future fertility in boys and adolescents who are able to produce semen via masturbation or, if needed, by assisted ejaculation [2]. The only option for prepubertal boys, however, is the cryopreservation of a small testicular biopsy. An algorithm of recommended steps and procedures for retrieval of testis tissue from boys of different developmental stages has been proposed by the European Society of Human Reproduction and Embryology (ESHRE) Task Force on fertility preservation [2] and adapted to specific countries like the UK [9, 63] and Belgium. Recent developments in clinical practices and patient management to preserve and restore fertility of prepubertal boys at high risk of fertility loss are discussed in the updated version of ESHRE Task force on Fertility preservation. Cryopreservation of immature testicular tissue fragments is proposed and favored against the cryopreservation of testicular cell suspensions. The most significant issue with digestion of the tissue is the loss of seminiferous tubule integrity. The stem cells that are removed from their somatic stem cell niches during digestion cells may be lost [24]. Cell suspensions will allow creation of options for germ transplantation or expansion of SSCs in vitro. However, these methods are still not clinical routine and will need optimization.
Another critical issue is the choice of cryoprocedures for cell suspensions or tissue fragments. An up-to-date summary of reports analyzing the effects of different cryopreservation strategies (i.e., uncontrolled or programmed slow freezing, vitrification), cryoprotectants, and supplements to cryopreservation media on functionality of SSCs testicular cell suspensions was recently published by Onofre et al. [24]. Highest recovery of viable cells (63±11%) was achieved when 1.5 M dimethyl sulfoxide (DMSO), 10% fetal calf serum and controlled slow freezing were applied on immature mouse testis cell suspensions [26]. Of the few studies that employed cryopreservation of adult human testicular cell suspensions, the highest total cell viability after thawing was 66% and 56±24% following programmed freezing and vitrification, respectively [14, 24]. However, the recovery of functional SSC and effects of cryopreservation on human testicular cell suspensions is unknown as no functional assays were performed.
However, the procedures for tissue retrieval and protocols for cryostorage are still experimental. Although the biopsy procedure is invasive, follow-up studies 12 months post-surgery provide evidence on its safety regarding postsurgical testicular growth and minimal adverse effects [25]. Ultimately, optimal cryopreservation protocols should ensure the tissue integrity, high post-thaw survival and functional capacity of the SSCs to restore fertility.
Cryobanking of testicular fragments is superior to cell suspensions since it preserves the native cell–cell interactions, the stem cell niche and epithelial barriers, and the exposure to the basement membrane and its extracellular matrix. Since isolation of SSCs can also be performed on the cryobanked material after thawing, it offers more versatile options regarding post-thaw fertility restoration approaches (i.e., tissue grafting and SSC in vitro procedures as well as germ cell transplantation). Tissue integrity, endocrine and paracrine activity, germ cell marker expression, and self-renewal were maintained when DMSO (0.7–1.5 M) in combination with patient serum or human serum albumin, HSA (5–10%), and without or with added sucrose were used to cryopreserve adult human testicular tissue (summarized in [24]). This was validated by post-thaw functional assessment via tissue fragment organotypic culture or single-cell suspension culture [14, 26, 27]. To date, three cryopreservation protocols for immature human testicular tissue have been reported, two of which [11, 13] are used to cryostore patients’ tissue in the academic European fertility preservation centers. Programmed slow freezing and cryoprotective agents that range from 1.5 M ethylene glycol, HSA, and sucrose [28], 0.7 M DMSO and HSA [11], or 0.7 M DMSO, HSA and sucrose [13, 15] were described. These protocols hold promise, since they preserved seminiferous epithelium and interstitial compartment integrity, and maintained spermatogonia numbers and their proliferative potential. However, the methods to evaluate the full reproductive potential of human testicular tissue after cryostorage are yet to be validated. Therefore, the technique is considered experimental and none of the currently used protocols for testicular tissue from prepubertal boys/adolescents is proven to be more efficient than the others.
Fertility Restoration Using Cryopreserved Testicular Tissue
Evidence-based clinical practice guidelines on fertility preservation and restoration strategies for adolescents and children with cancer are issued by multidisciplinary teams of pediatric oncologists and endocrinologists, oncologists and fertility specialists [2, 29–31]. Although fertility restoration strategies are still experimental in man, they are accepted as potentially relevant to male childhood cancer survivors. One method is autotransplantation of an enriched suspension of SSCs by injection into the testis to regenerate spermatogenesis, which was the topic of Chapter 12. There is increasing body of evidence for the clinical implementation potential of auto-/xenografting of frozen-thawed testicular tissue back into the testis or in ectopic sites [32, 64].
Autologous transplantation of testicular tissue pieces back to the adult childhood cancer survivor is a promising fertility restoration strategy, only when there is no risk of reintroducing cancer cells contained in the tissue. The first success of complete spermatogenesis regeneration with this technique was described when prepubertal testicular pieces from mice, goats, and pigs were allo- (same species, another individual) or xeno- (another species) transplanted under the back skin (ectopically) of castrated immunodeficient mice [33]. This technique proved its feasibility to support complete spermatogenesis in fresh and cryopreserved grafts from adult- or prepubertal animals when those grafts were auto- or xenotransplanted into mouse hosts. A comprehensive review of studies on cryopreserved testicular tissue grafting in various animal models of different ages is provided by Onofre et al. [24]. Primate sperm were obtained after xenotransplantation of fresh or cryopreserved testicular tissue from cynomolgus and rhesus macaques [34–36]. The clinical potential of the technique was demonstrated when healthy offspring developed after performing intracytoplasmic sperm injection (ICSI) with spermatozoa that had developed in juvenile macaque ectopic xenografts [32]. The first proof of principle for the imminent translational value of autologous grafting of cryopreserved prepubertal testicular tissue came with the birth of rhesus macaque female baby Grady (graft-derived baby) following ICSI by using live sperm retrieved from autologously transplanted grafts. Complete spermatogenesis and sperm recovery were achieved after 8–12 months post-grafting in castrated immature rhesus macaques (under the skin and in the scrotum), both from fresh and cryopreserved grafts. As yet no human sperm production was reported using this technique. Blockade of germ cell differentiation was observed after ectopic autologous transplantation in marmosets [37]. Besides the phylogenetic distance between species regarding germinal epithelium organization and stem cell system, other factors might impact the limited development of human and marmoset xenografts. Potential effects of different factors (e.g., host endocrine environment, maturational stage of the donor tissue, and grafting procedure) on the outcomes of testicular tissue transplantation are reviewed in [3, 38, 39]
While complete spermatogenesis was successfully achieved in ectopic grafting with several mammalian species, the site for transplantation in primates, including human, can affect graft survival, spermatogonial recovery, and reinitiation of spermatogenesis. Thus, autologous grafting to the scrotum, but not to ectopic sites (back), supported complete spermatogenesis in immature marmosets [40, 61]. Similarly, complete spermatogenesis and spermatozoa were observed following intratesticular xenotransplantation of immature marmoset tissue in nude mice. Another study investigating optimal grafting locations in irradiated prepubertal macaques reported generation of sperm, when testis tissue was autologously transplanted in the scrotum [36]. However, a strikingly low number of grafts were recovered. Significant improvement in graft survival and spermatogenesis efficiency was demonstrated when neonatal murine fresh and cryopreserved testis tissue was transplanted under tunica albuginea, in testicular parenchyma (intratesticular grafting) [41]. Initial xenotransplantation studies with fresh adult and prepubertal human testis tissue demonstrated poor graft survival, with major germ cell loss and hyalinized or Sertoli cell-only tubules, following short- and long-term ectopic grafting [42, 43]. However, ectopic xenotransplantation of fetal human testis tissue supports progression of germ cell differentiation from gonocytes to pre-spermatogonia and maturation initiation of Leydig, peritubular, and Sertoli cells [44–46, 60]. Orthotopic grafting (in scrotum or intratesticular) resulted in high graft recovery and seminiferous tubular integrity, 55–98.3%, in prepubertal human xenografts as well [13, 47, 48]. A summary of human fetal and (pre)pubertal testicular grafting studies is provided in Table 13.2. Although xenografting studies with immature human testis tissue report different spermatogonia recovery rates, those rates were comparable between fresh and cryopreserved grafts within each study [13, 43, 47–49]. Thus, spermatogonia recovery rate in fresh grafts (number of spermatogonia per tubule in fresh grafted tissue/number of spermatogonia per tubule in fresh non-grafted tissue) were 3.0–84.7% [49], 3.4% [48], 7.5–80% [49] and 93.7% [47] after 4, 6, 9, and 12 months of grafting, respectively. Interestingly, the higher recovery rates for each time point of graft analysis was observed in tissue from donors at pubertal age (12–13 years old) [47, 49]. Similarly, spermatogonia recovery rates in cryopreserved grafts were 9.1–24% [49], 3.7–4.1% [13, 48], 27.3–60.4% [49], and 83.9 % [47] after 4, 6, 9 and 12 months of grafting, respectively. Nevertheless, differentiation of spermatogonia did not progress beyond the stage of primary pachytene spermatocytes in none of the studies published so far. Since steroidogenesis potential of Leydig cells was qualitatively confirmed by IHC and Sertoli cells expressed markers of functionality (Table 13.2), it could be implied that the inefficient outcomes with human testis xenografts are due to affected germ cells. It was further suggested that hypoxia insult during the avascular period directly post-grafting and/or inappropriate graft environment render the SSCs pool susceptible to accelerated depletion [50].
Table 13.2 Overview of human testis tissue xenografting
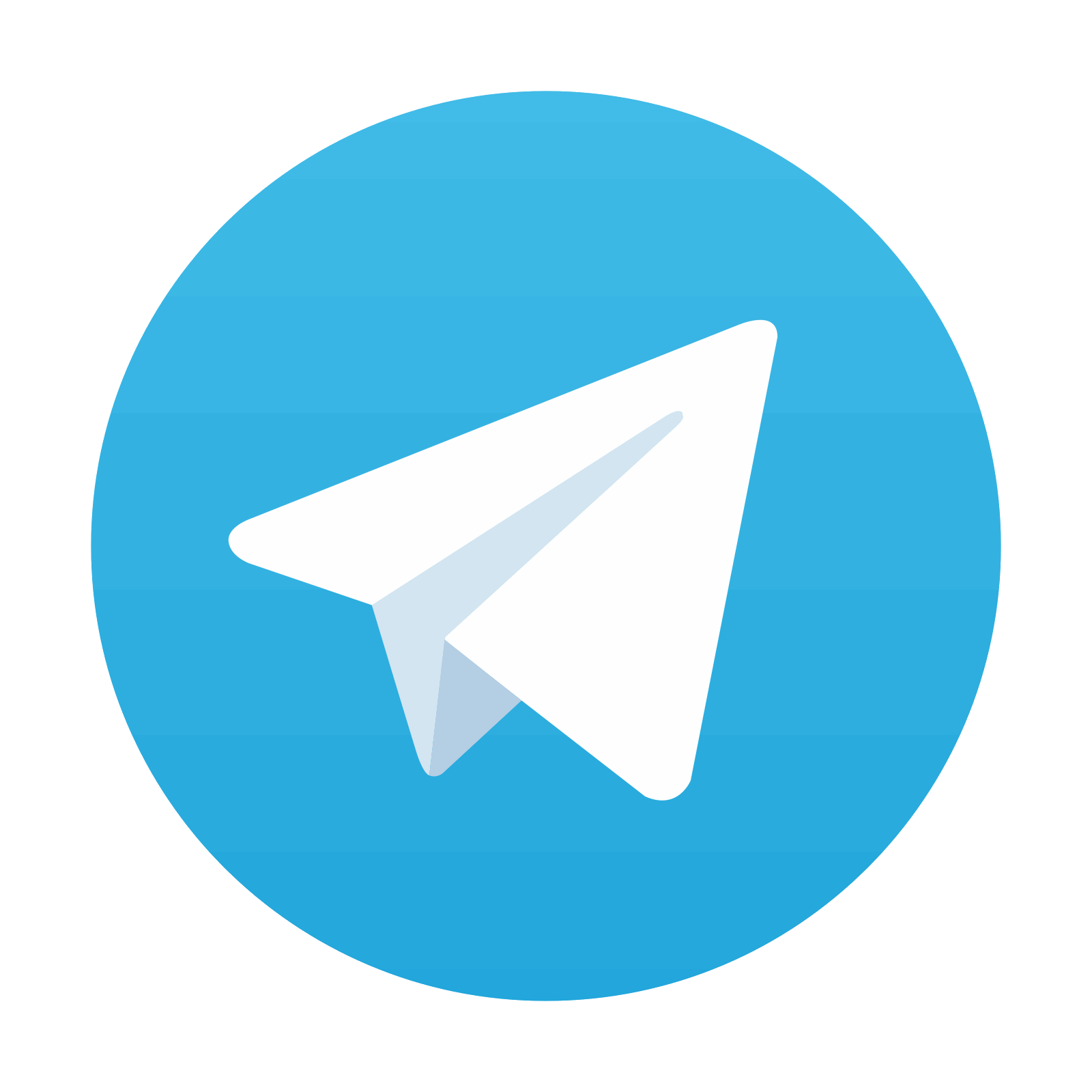
Stay updated, free articles. Join our Telegram channel

Full access? Get Clinical Tree
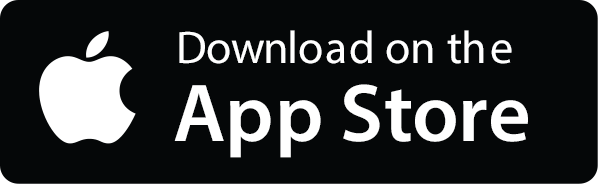
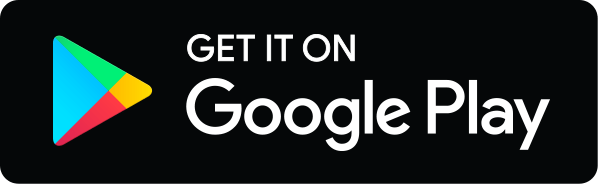
