Abstract
Cancer is one of the most important public health problems all around the world. It is the second leading cause of death globally. Thousands of young women are diagnosed with different types of hematological and solid malignancies every year, and exposed to cytotoxic chemotherapy regimens and radiation [1]. In the United States, the ten leading cancer types in females are breast, lung and bronchus, colon and rectum, thyroid, melanoma, non-Hodgkin’s lymphoma (NHL), pancreas, leukemia, and kidney and renal pelvis [2]. In Europe, hematological malignancies account for around 9% of all cancers and are the fourth most frequently diagnosed cancer in both men (after prostate, lung, and colorectal) and women (after breast, lung, and colorectal) [3]. For all cancers combined, the five-year relative survival rate is 68%. The survival is highest for prostate cancer (99%), melanoma of the skin (92%), and female breast cancer (90%) and lowest for cancers of the pancreas (8%), lung (18%), and liver (18%)
Introduction
Cancer is one of the most important public health problems all around the world. It is the second leading cause of death globally. Thousands of young women are diagnosed with different types of hematological and solid malignancies every year, and exposed to cytotoxic chemotherapy regimens and radiation [1]. In the United States, the ten leading cancer types in females are breast, lung and bronchus, colon and rectum, thyroid, melanoma, non-Hodgkin’s lymphoma (NHL), pancreas, leukemia, and kidney and renal pelvis [2]. In Europe, hematological malignancies account for around 9% of all cancers and are the fourth most frequently diagnosed cancer in both men (after prostate, lung, and colorectal) and women (after breast, lung, and colorectal) [3]. For all cancers combined, the five-year relative survival rate is 68%. The survival is highest for prostate cancer (99%), melanoma of the skin (92%), and female breast cancer (90%) and lowest for cancers of the pancreas (8%), lung (18%), and liver (18%) [2].
Unfortunately, modern combination chemotherapy and radiotherapy regimens have a substantial negative impact on reproduction. Premature ovarian failure and other poor reproductive outcomes subsequent to cancer therapies are being recognized as long-term sequelae of cancer therapies. Chemotherapy drugs exert cytotoxic effects systemically and can damage the ovaries, resulting in infertility, premature ovarian failure, and to lesser extent spontaneous abortions. They have very limited or no deleterious effects on the uterus that can be recognized clinically. By contrast, radiation is detrimental to both the ovaries and uterus, thereby causing a greater magnitude of adverse effects on the female reproductive function. These include infertility, premature ovarian failure, miscarriage, fetal growth restrictions, perinatal deaths, preterm births, delivery of small for gestational age infants, preeclampsia, and abnormal placentation [4]. Therefore, preservation of ovarian and uterine function and fertility has become one of the major quality-of-life issues for cancer survivors at reproductive ages. In this chapter, first we will outline the mechanisms of ovarian and uterine damage occurring after exposure to chemotherapy drugs and radiation commonly used in the treatment of hematological and gastrointestinal malignancies, and then discuss fertility preservation issues in patients with these malignancies.
Chemotherapy
Ovary is the most sensitive organ to chemotherapy drugs in the female reproductive system (Figure 11.1). By contrast, uterine function does not appear to be compromised by chemotherapy agents. Chemotherapy agents of alkylating category are the most detrimental to the ovary, which cause massive follicle loss by inducing genomic damage in the oocyte and somatic cells of primordial follicles [5, 6] . Emerging data suggest that there are some other mechanisms that contribute to the gonadotoxic effects of chemotherapy drugs on the ovary in addition to their direct toxic effects on the oocytes. One of these proposed mechanisms involves vascular damage. It was shown by different groups that chemotherapy drugs injure the blood vessel in the cortical and medullary portions of human ovary, which results in obliteration, fibrosis, and decreases in the expression of VEGF and microvascular intensity [7, 8]. These pathological changes may further aggravate follicle loss and accelerate aging of the ovary. The second mechanism is the so-called burn out phenomenon. It was hypothesized that cyclophosphamide exposure activates phosphatidylinositol 3-kinase signaling pathway, which in turn causes premature activation of primordial follicles and hence “burn out’’ or early depletion of follicle pool [9]. It was also suggested as a third possible mechanism, at least in the mouse ovary that chemotherapy drugs may induce different mechanisms of follicle loss. Exposure to cisplatin caused oocyte-specific damage, whereas doxorubicin preferentially induced damage to the mitotic granulosa cells of secondary follicles. In support of the burn-out theory, investigators also observed that primordial follicles were lost after exposure to both drugs and only a few of them were positive after staining with apoptosis marker TUNNEL, suggesting that the reduction in primordial follicle numbers after chemotherapy exposure is due to accelerated growth initiation rather than a direct toxic effect of the drugs [10]. Last, autopsy series of young girls who had been treated with cytotoxic drugs for leukemia and other childhood cancers demonstrated no development or arrested follicle growth, atresia of small-sized follicles along with a marked decrease in the size and number of antral follicles [11]. Taken together these findings suggest that there could be multiple distinct pathogenetic mechanisms underlying chemotherapy-induced gonadotoxicity and ovarian failure.
Figure 11.1 The impact of chemotherapy drugs and radiation on the female reproductive system. Chemotherapy drugs mainly impact the ovary. Genomic damage in the oocyte and surrounding granulosa cells result in apoptotic death of the follicle, leading to a decrease or total exhaustion of the follicle pool depending upon the extent of ovarian damage. Radiation impacts not only the ovary but also uterus and hypothalamic–pituitary ovarian (HPO) axis. Uterine exposure to radiation during pelvic, spinal, abdominal, or total body irradiation causes irreversible damage to endometrium, myometrial layer, and vascular structures, causing a wide range of adverse reproductive and obstetrical outcomes
Alkylating agents such as busulfan, cyclophosphamide, lomustin, and procarbazine have the highest gonadotoxic potential. Cytotoxic actions of the drugs at this category are not cell-cycle specific. Therefore, they are able to produce a greater magnitude of damage by affecting the cells at every stage of cell cycle. They alter base pairs, produce DNA cross-links (guanosine adducts), and cause single and double strand breaks in the DNA [12]. Unfortunately, many of these alkylating drugs are included in the first-line therapy of many solid and hematological malignancies such as Hodgkin’s lymphoma (HL) [1]. High-dose cyclophosphamide (120–200 mg/kg) with and without busulfan (8–16 mg/kg) is commonly used as conditioning therapy before bone marrow transplantation (BMT) [13]. DNA interstrand cross-linking drugs (cisplatin and carboplatin) closely follow the alkylating agents with regard to gonadotoxicity and are called “alkylating like agents.” Drugs in these categories destroy both primordial follicle pool and growing follicle fraction in human ovary, thereby creating more extensive gonadal damage [8]. By contrast, microtubule depolymerization inhibitors (Taxanes) and DNA intercalating agents (Anthracyclines) are less toxic than alkylating agents and platinum compounds, and therefore considered to have moderate gonadotoxicity. The members of the antimetabolite group such as methotrexate and 5-fluorouracil and vinca alkaloids (vincristine and vinblastine) have minimal or no gonadotoxicity. Growing follicle fraction, pre-antral, and antral follicles with their mitotic granulosa cell layer are more sensitive to the cytotoxic actions of mitosis-specific drugs possibly due to their higher mitotic rate and increased metabolic demand. Chemotherapy drugs are generally administered in combinations, precluding one from estimating individual effects of each drug in a combination regimen. Total dose, dose-density, and ovarian reserve of the patients prior to initiating chemotherapy are other confounders that modify the gonadotoxic effect of a given drug. In other words, drugs that are thought to have minimal or moderate gonadal toxicity can induce a significant degree of ovarian damage and jeopardize future fertility if they are used for longer period of time and/or at higher doses especially in patients with poor ovarian reserve prior to initiating chemotherapy.
These modalities have a detrimental effect on ovarian function. Ionizing radiation and chemotherapy agents induce genomic damage and apoptosis in both oocytes and the surrounding granulosa cells, culminating in apoptotic death of the follicular apparatus [1, 14]. The clinical manifestations of chemotherapy-induced ovarian damage may range from temporary menstrual irregularity to amenorrhea, infertility, and premature ovarian failure depending upon the extent of the damage in the ovarian follicle pool. Resting primordial follicles, the earliest form of follicles constitute 90% of the follicle pool (ovarian reserve) with the remaining 10% belonging to the growing follicles at primary stage and beyond [15]. The probability of developing such adverse reproductive outcomes depends on multiple factors such as the age and ovarian reserve of the patient, and the type, dose, and duration of the chemotherapy regimen. Younger patients (age <40) have larger ovarian reserve (higher number of primordial follicles in their ovaries), and therefore are more likely to retain or regain menstrual function than those older than 40, after exposure to chemotherapy drugs (22–56% vs. 11%) [16]. A chemotherapy agent that preferentially targets the primordial follicles may result in the diminishment or total exhaustion of ovarian reserve depending upon the toxicity, dose, and duration of the therapy. If the loss of ovarian function develops during or shortly after the completion of cancer therapy, it is termed “acute ovarian failure” (AOF). AOF generally reflects the destruction of growing follicle fraction, especially antral follicles and pre-ovulatory follicle, which are the main sources of sex steroid secretion. AOF may be reversible depending upon the extent of the damage in the follicle stockpile. But it should be remembered that return of menses does not guarantee a normal reproductive life span since the loss of primordial follicles may occur insidiously without any warning sign or menstrual abnormalities. This also explains why women with critically diminished ovarian reserve may continue to menstruate regularly. For survivors who retained or resumed ovarian function after the completion of cancer treatment, a subset will go on to experience menopause before 40 years of age, which is classified as having premature menopause.
Radiation
Direct cytotoxic action of radiation on the genome of the cell is the predominant mechanism of damage for particle radiation. Genomic damage mainly occurs as double strand breaks in DNA and leads to irreversible loss of vital homeostatic functions and eventual death of the cell. There are also indirect cytotoxic actions of radiation that come from its interaction with other substances in the cell such as water. Radiolysis of cellular water leads to formation of free radicals which further amplifies the damage in the DNA and organelles of the cell. This mechanism is particularly true for ionizing radiation such as X-rays. Mitotically active cells are more vulnerable to cytotoxic actions of radiation because of active DNA replication, whereas those with low metabolic and division rates and hypoxic cells are more resistant to it. Oocyte is one notable exception to this principle. Even though cell cycle is arrested in human oocyte at the diplotene stage of prophase-I of the first meiotic division, it is somehow exquisitely sensitive to radiation. Lethal dose (LD50), the radiation dose required to destroy 50% of the human oocytes is ~2 Gray (Gy) [17]. It is currently unclear why such an interphase nucleus is so sensitive to radiation. It was believed in the past that oocyte does not have an enzymatic repair capacity to counteract the modifications caused to its DNA by ionizing radiation and/or chemicals. But emerging evidence from animal studies in the mouse and guinea pig ovaries showed that mammalian oocyte is indeed able to repair DNA damage induced by radiation and that radiosensitivity of ovarian follicles differs depending upon their developmental stages [18, 19]. There is also evidence that like other mammalian species human oocyte expresses several DNA repair genes [20], but their role in repair of radiation-induced genomic damage in human oocyte is still unclear. Radiation induces genomic damage in the oocyte and the surrounding granulosa cells leading to atresia of the follicle. Depending upon the extent of damage, ovarian follicle pool is either decreased or totally exhausted. Autopsy series of the young girls who had been treated with radiation for abdominal tumors showed that their ovaries are severely damaged, the proportion of atretic small follicles are increased, and follicle growth is arrested in comparison to healthy girls who died of accidents [21]. Wallace et al. utilized the Faddy–Gosden model to predict the age at which ovarian failure is likely to occur after exposure to radiation by considering age and radiation dose. The model incorporates decay as an instantaneous rate of temporal change based on the remaining population pool. He showed that the effective sterilizing dose (ESD), or dose of fractionated radiotherapy at which ovarian failure occurs immediately after treatment in 97.5% of patients, decreases with increasing age at treatment. The estimated ESD at birth was 20.3 Gy; at 10 years, 18.4 Gy; at 20 years, 16.5 Gy; and at 30 years, 14.3 Gy. This model can be a helpful guide to estimate the likelihood of ovarian failure from birth to 50 years after exposure to radiation at any given dose. It should be noted that there is a considerable individual variability in ovarian reserve between patients at the same age. In other words, chronological age of a given patient may not necessarily match her ovarian age. This fact accounts for the differences in the onset of premature ovarian failure between patients treated at the same age.
Ovarian damage occurs by its direct exposure to radiation during pelvic or low abdominal, lumbo-sacral, spinal and total body irradiation [22]. Furthermore, scatter radiation may induce significant ovarian damage even if they are not within the radiation field. The risk of ovarian damage and premature ovarian failure increases with incremental doses of radiation. Single dose appears to be more toxic than fractionated doses [17]. The probability of pregnancy in female survivors is 0.56 if ovaries are exposed to 5–10 Gy radiation. It further decreases to 0.18 if ovarian radiation dose exceeds 10 Gy [23]. Unfortunately, detrimental effect of radiation in the female reproductive system is not limited to the ovary. Uterus is also targeted by radiation. Radiation-induced damage in the endometrium, myometrium, and vascular structures in the uterus can significantly compromise its functions and potentially lead to infertility and adverse pregnancy outcomes such as miscarriages, still births, fetal growth restrictions, preeclampsia, and preterm deliveries in the survivors exposed to uterine radiation during childhood [22, 24–26] (Figure 11.1). Different pathophysiological mechanisms play a role in the development of uterine dysfunction after exposure to radiation. First, radiation causes damage, sclerosis, and obliterations in the vascular structures of the myometrial and endometrial layers of the uterus leading to decreased uterine blood flow. If pregnancy occurs, this will potentially impair the invasion of cytotrophoblast in the placental bed resulting in defective placentation, decreased fetal-placental blood flow, and fetal growth restriction [22, 27]. Radiation-induced damage in endometrium may also prevent normal decidualization and lead to disorders of placental attachment, such as placenta accreata if pregnancy can be achieved [27–31]. Second, uterine elasticity and volume can be decreased from radiation-induced myometrial damage, which can lead to preterm labor and delivery [28]. Third, radiation-induced damage in the glandular and stromal components of endometrium with particular destruction of progenitor basal cell layer may cause infertility by inducing endometrial atrophy and preventing implantation [31]. Survivors of childhood cancers are more likely to have increased clinical infertility and prolonged time to achieve pregnancy (relative risk [RR] 1.48 [95% CI 1.23–1.78]; p < 0.0001) than their siblings even though they retain ovarian function after exposure to radiation [32]. Pathological changes in uterus induced by radiation may at least in part account for subfertility observed in these survivors.
Gastrointestinal Tumors
The treatment of the cancers of the gastrointestinal system often involves radiotherapy and chemotherapy as (neo)/adjuvant setting in addition to surgery. Oxaliplatin, 5-FU, Capecitabine, Irinotecan, cetuximab, bevacizumab, panitumumab, or Ziv-aflibercept are used pre- or postoperatively as adjuvant therapy in the treatment of colorectal cancers [33–36]. Among these drugs oxaliplatin is more detrimental to the ovary than the others. Oxaliplatin and other platinum-containing cancer drugs cause the DNA strands to cross link, which impair DNA replication and mitosis, and ultimately induce apoptosis. 5-FU and its orally administered pro-drug form, capecitabine, have the least or no ovarian toxicity at all [1]. Oxaliplatin is combined with 5-FU and leucovorin, known as FOLFOX regimen. In a study of 73 females under age 50 who were treated with this regimen, 41% (n = 20) experienced amenorrhea during chemotherapy. Sixteen percent had persistent amenorrhea 1 year after completion of chemotherapy. The incidence of amenorrhea during chemotherapy trended higher in patients aged older than 40 compared with patients aged 40 and younger (59% vs. 31%; p = 0.075). There was no statistically significant difference in persistent amenorrhea between the two age groups (24% vs. 13%; p = 0.42) [37]. A similar study compared the incidence of amenorrhea in 95 premenopausal women with colon carcinoma and 67 premenopausal women with rectal carcinoma aged 40 years or younger. Patients with stage II or III colon cancer were treated with adjuvant FOLFOX (5-fluorouracil, leucovorin [folinic acid], oxaliplatin), XELOX (capecitabine, oxaliplatin), or capecitabine after optimal surgery. Patients with stage II or III rectal cancer were treated with neoadjuvant or adjuvant chemoradiotherapy. The incidence of amenorrhea was no different between the women receiving adjuvant FOLFOX and those receiving XELOX (4.9% vs. 5.6%; p = 0.913). Of the 51 patients with rectal cancer, 48 patients (94.1%) experienced a cessation of menses during chemoradiotherapy, and no patient resumed menses after the completion of chemoradiotherapy; Only three (5.9%) patients maintained menses after the completion of treatment. Thus, the incidence of amenorrhea was significantly lower in patients with colon cancer (4.2%; 3 of 72) than in patients with rectal cancer (94.1%; 48 of 51) (p < 0.01) [38].
Limited data on the gonadotoxicity of irinotecan and other topoisomerase suggest that they are more toxic to the ovary than antimetabolite drugs. Irinotecan-induced apoptosis of the granulosa cells of the ovarian follicles by up-regulating FasL expression [39, 40]. Cetuximab is an epidermal growth factor receptor (EGFR) inhibitor. Panitumumab is a fully human monoclonal antibody specific to the epidermal growth factor receptor. Zivaflibercept is an inhibitor of vascular endothelial growth factor. These drugs are used for the treatment of metastatic colorectal cancer and no data is available on their ovarian toxicity. Bevacizumab is an angiogenenesis inhibitor drug. In 2011 FDA added a warning label to this drug because of ovarian toxicity documented in a prospective study conducted by the developer company. In that study, 179 premenopausal women were randomized to receive chemotherapy with or without bevacizumab; the incidence of ovarian failure was higher in the bevacizumab arm (34%) compared to the control arm (2%). After discontinuation of bevacizumab and chemotherapy, recovery of ovarian function occurred in 22% (7/32) of these bevacizumab-treated patients (FDA) [41].
Imatinib mesylate is an inhibitor of the oncogenic tyrosine kinases BCR-ABL and c-kit used in the treatment of chronic leukemias and gastrointestinal tumors, respectively. There are some case reports that show gonadotoxic effects of this drug on human ovary [42, 43]. Animal studies assessing the protective role of this drug against cisplatin-induced ovarian toxicity yielded conflicting results [44, 45]. A recent study utilizing human ovarian xenograft model showed that imatinib had gonadotoxic effects on human ovary and does not confer any protection against cisplatin-induced follicle death [46].
Docetaxel, cisplatin, oxaliplatin, 5-FU, capecitabine, epirubicin, and irinotecan are commonly used before or after surgery as adjuvant therapy in the treatment of resectable or recurrent gastric carcinomas [47]. Cisplatin and oxaliplatin pose greater risk of ovarian damage than the others. Data on ovarian toxicity of docetaxel are largely derived from breast cancer patients receiving taxane-based chemotherapy protocols. For instance, PACS01 trial comparing six cycles of FEC vs. three cycles of FEC + three cycles of docetaxel showed that patients receiving six cycles of FEC vs. three cycles of FEC followed by three cycles of docetaxel had similar rates of amenorrhea at the end of chemotherapy (93% vs. 92.8%) [48]. However, one year later more patients in the 3FEC/3D arm than in the 6FEC arm recovered menses (35.5 % vs. 23.7 %; p < 0.05), and had premenopausal hormone levels (43% vs. 29%). There was an increased incidence of reversible amenorrhea (i.e., resumption of menses or recovery of premenopausal hormone values) in the taxane-containing arm for patients aged more than 40 years (20.5% vs. 10.5%; p = 0.025), whereas there was no difference in patients below 40 years of age suggesting that the addition of docetaxel to FEC regimen may increase the gonadotoxic potential of the regimen in older patients. The Breast Cancer International Research Group (BCIRG) 01 trial showed that the incidence of amenorrhea was higher in the docetaxel, doxorubicin, and cyclophosphamide (TAC) arm compared with the fluorouracil, doxorubicin, and cyclophosphamide (FAC) arm (51.4% vs. 32.8%, respectively) [49]. In the light of these data, it can be concluded that docetaxel has a moderate gonadotoxic potential.
Similar to other anthracyclines, epirubicin acts by intercalating DNA strands. Intercalation results in complex formation which inhibits DNA and RNA synthesis. It also triggers DNA cleavage by topoisomerase II, resulting in mechanisms that lead to cell death. Binding to cell membranes and plasma proteins may be involved in the compound’s cytotoxic effects. Epirubicin also generates free radicals that cause cell and DNA damage [1]. Anthracyclines are far less toxic than alkylating agents and platinum drugs. Amenorrhea was reported by 80% of premenopausal patients who received anthracycline-based regimens for breast cancer. However, none of the patients under 30 years of age had menstrual abnormalities, whereas 96% of those 40–49 years of age developed amenorrhea. Amenorrhea was permanent for most women over 40, but it was reversible for 50% of patients under 40 years of age [50].
Pre- and postoperative radiation were demonstrated to decrease local recurrence, and to increase not only local control but also disease-free and overall survival with concurrent chemotherapy in colorectal cancers (1985; [52–56]. The radiation dose increases up to 50 Gy, which has a substantial negative effect on the pelvic organs. To prevent radiation-induced ovarian failure, infertility, and premature ovarian failure, ovarian transposition can be considered if the patient is young and childbearing is not completed yet. However, it should be remembered that the clinical consequences of uterine exposure to radiation can be devastating.
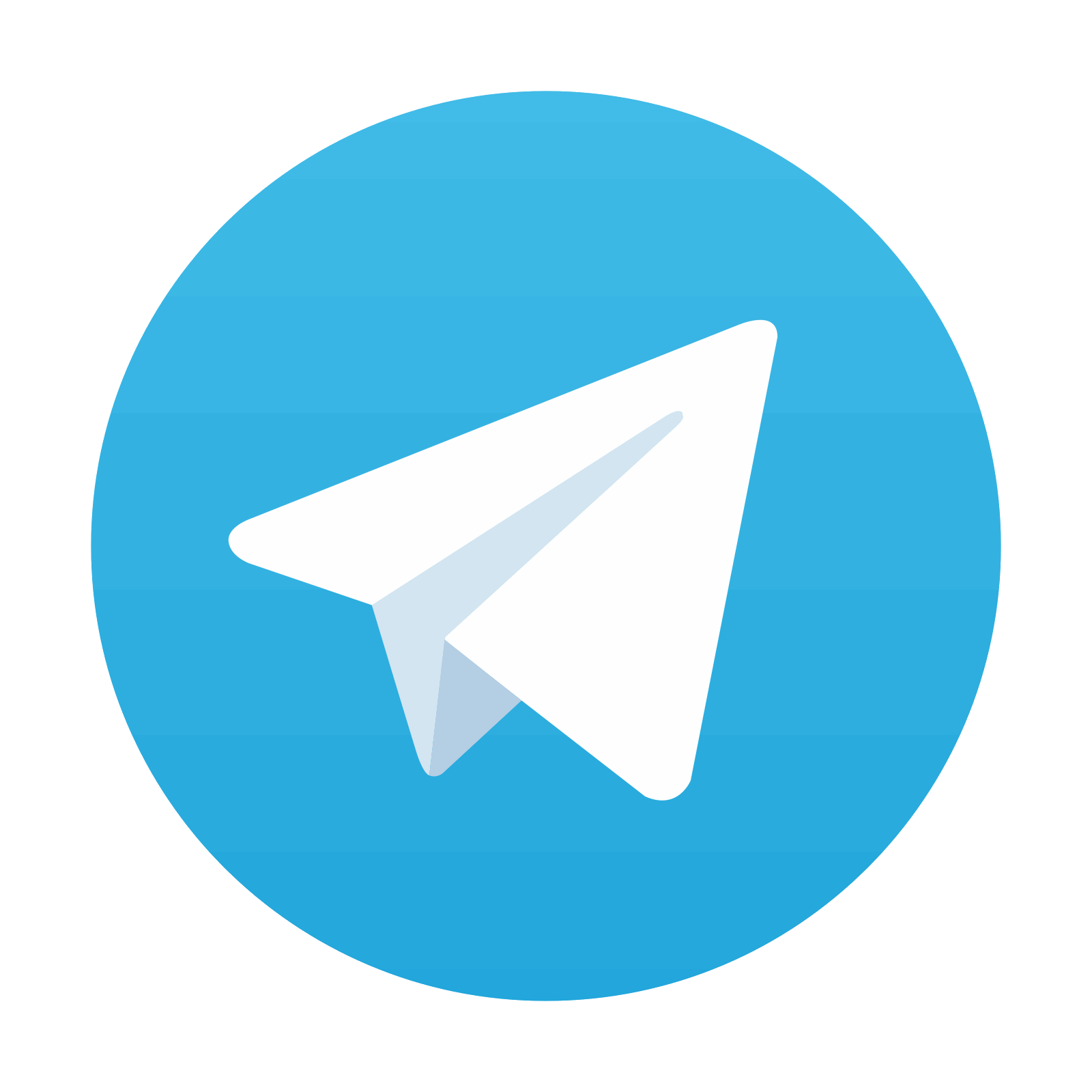
Stay updated, free articles. Join our Telegram channel

Full access? Get Clinical Tree
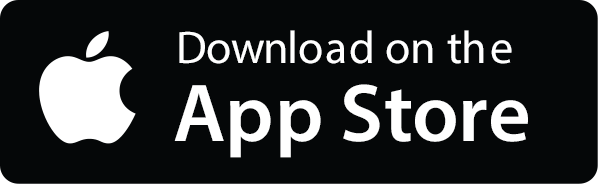
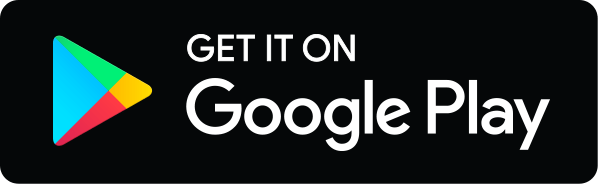