Abstract
Human reproduction is an inefficient process. Still today is no clear definition for recurrent implantation failure (RIF). It is a condition arising from the failure of a successive number of in vitro fertilization cycles (IVF) in which theoretically the pregnancy should have already been achieved. Due to the disparity in the implantation and pregnancy rates among different assisted reproduction centers, a consensus definition has not been achieved. Technological and scientific advances in the field of ART have led to better reproductive outcomes. Despite all the efforts, around 30 percent of healthy euploid embryos still fail to implant today ().
10.1 Introduction
Human reproduction is an inefficient process. Still today is no clear definition for recurrent implantation failure (RIF). It is a condition arising from the failure of a successive number of in vitro fertilization cycles (IVF) in which theoretically the pregnancy should have already been achieved. Due to the disparity in the implantation and pregnancy rates among different assisted reproduction centers, a consensus definition has not been achieved. Technological and scientific advances in the field of ART have led to better reproductive outcomes. Despite all the efforts, around 30 percent of healthy euploid embryos still fail to implant today (1).
Disruptions in maternal immune tolerance have been invoked as one cause of implantation failure. The original theory was that immunosuppression was required to tolerate the presence of the allogeneic fetus. Owing to repeated failed cycles even after gamete donation, we all have witnessed increasing patient demand for immune tests and “immune treatments.”
The role of the immune system in recurrent miscarriage (RM) and recurrent implantation failure (RIF) is one of the most controversial issues in ART (2). Controversy is partly due to the fact that most studies of the immune system in reproduction have focused on finding markers of peripheral blood mostly based on the number or percentage of specific immune cells (3, 4) and on the use of immunosuppression (5, 6). The main reason why immune treatments have failed so far, and why immune tests (peripheral blood natural killer [pbNK] or uterine natural killer [uNK] cell testing) have shown very weak or no predictive values, is basically poor study design and great patient heterogeneity (7).
Immune system cells have an important role in immune defense and reproduction. Natural killer (NK) cells have become a central element in immunological studies for women suffering recurrent miscarriage (RM) and recurrent implantation failure (RIF). They specialize in killing virus-infected and tumor-transformed target cells by the balanced action of both activating and inhibitory receptors (8). Several studies have tagged NK cells as killers in charge of rejecting the embryo, and have associated this function with reproductive failure (4).
Uterine NK cells (uNK) are phenotypically distinct from those circulating in blood; they are CD56bright, CD16- whereas 90 percent of blood NK cells are CD56dim, CD16+ (9). The minor CD56bright population in blood also differ from those in the uterus morphologically and for many other surface markers. These differences mean that measurements of any NK parameters in NK cells from blood will not be informative in relation to function of uNK (5).
Uterine NK cells proliferate and differentiate in the mucosa. They are not present premenarche or postmenopausally and their dependence on the ovarian hormone, progesterone, is obvious from the great surge in proliferative activity after ovulation. This is mediated by increased expression of IL-15 from stromal cells in response to progesterone. The presence of uNK in the decidua is maximal in the first trimester and numbers thereafter fall so although they are still present at term they are not abundant (10).
Killer immunoglobulin-like receptors (KIRs) determine the NK cell function in the context of other receptor-ligand interactions and permutations of 28 NK cell receptors, which result in at least 10,000 different NK cell subsets in a given individual (11). Furthermore, the KIR repertoires of uNK and pbNK differ when taken from the same woman at the same time.
The activity of the uterine NK cells, CD56brightCD16-, is influenced by the KIR repertoires, and differ absolutely from pbNKs in phenotype markers and functional activity (12). The uNK killing function is very weak compared with pbNK. With infections, this can change as the CMV infection elicits a different uNK effector function. uNK cells are capable of controlling cytomegalovirus (CMV) infection and acquiring the cytotoxic phenotype against CMV-infected decidual fibroblasts by means of receptor repertoire modulation (13), but this process has not been shown against a healthy embryo.
The number and function of pbNK and uNK cells show wide variability depending on the patient’s clinical condition, e.g., infections, autoimmunity, or tumor or the day of menstrual cycle. Studies of NK cells and reproductive issues have not taken this NK cell physiologic variation into account, nor differences in pbNK and uNK cell receptors, whose activation is essential for its functions.
Thus, the increased number of uNKs in the secretory phase of the normal menstrual cycle and pregnancy (90 percent of local immune cells in the first trimester of pregnancy) is a physiologic process that focuses on helping embryo implantation and is not a marker of “embryo rejection.”
However, this physiological maternal–fetal immune tolerance could be imbalanced with a negative impact over embryo implantation and placentation. The maternal–fetal tolerance failure, a tissue-restricted process, does not have a systemic impact on the peripheral immune cells and tests based on the percentage or number of peripheral blood immune cells, Th1 cytokine, or NK cytotoxicity assays did not reflect the immune endometrial imbalance.
The expression of maternal immune system dysregulation could be
-
1. a lack of activation with negative signals into the maternal–fetal tolerance and impaired embryo implantation or placentation
-
2. an over reactivity with an increased inflammatory uterine environment and damage on the trophoblast cells
10.2 Maternal Adaptation to the Fetus
Successful maternal adaptation to the semiallogenic fetus occurs in the uterus at the site of placentation. The key of materno-fetal tolerance process is the remodeling of the spiral arteries, with destruction of the media by invading extravillous trophoblast (EVT) cells.
Most immune cells in the endometrium are tissue-resident cells and their number, type, and activation state are highly dependent on the hormonal environment. Maternal uterine immune cells actively respond to fetal antigens, promoting maternal–fetal immune tolerance as observed in a normal pregnancy. Uterine NK cells (uNK) regulate trophoblast invasion and enhance vascular remodeling by extravillous trophoblast (11) and Treg cells (FoxP3+Treg), promoting maternal–fetal immune tolerance. Human trophoblasts express a battery of immune inhibitory molecules predominantly targeting T cells (CD4+ or CD8+), such as Fas ligand or indoleamine 2,3-dioxygenase (IDO), which are potent inducers of T cell apoptosis as a protective mechanism of maternal–fetal tolerance. The interaction between trophoblast HLA-G dimers and LILRB1 receptors expressed by macrophage and dendritic cells also promotes maternal–fetal tolerance.
In terms of allorecognition, interactions between members of the killer immunoglobulin-like receptor (KIR) family expressed by uNK cells binding to trophoblast HLA-C molecules are of particular interest, as both maternal KIR and fetal HLA-C genes are highly polymorphic. This means that there will be different maternal/fetal genetic combinations in each pregnancy. The variability in the KIR gene family is both at the level of presence/absence of a gene and the individual allelic variability at each KIR locus.
Placentation is regulated by interactions between maternal KIRs expressed by uNKs, and fetal HLA-C molecules, expressed by EVTs. Hiby et al. (14) showed that invading EVTs are the principal site of HLA-C expression in the decidua basalis and that both maternal and paternal HLA-C allotypes are presented to KIRs. Insufficient invasion of the uterine lining by trophoblasts and vascular conversion in the decidua are thought to be the primary defect in disorders such as recurrent miscarriages (RM), preeclampsia, and fetal growth restriction (FGR).
Several studies conducted in natural pregnancies by Hiby and Moffett (15) showed that women who have a KIR AA genotype (two KIR A haplotypes) are at risk of preeclampsia and other pregnancy disorders when the fetus has more HLA-C2 genes than the mother and when additional fetal HLA-C2 alleles are of paternal origin.
Also, they described that protection from preeclampsia is likely mediated by activating KIR2DS1 (B haplotype), which also binds HLA-C2. Thus, depending on the particular KIR–HLA-C interaction, trophoblast cell invasion is regulated.
Assisted pregnancies differ from medically unassisted pregnancies. These patients receive sometimes more than one embryo per transfer, and also donor oocytes, sperm donor or embryo donation, are often used. After double embryo transfer (DET), the expression of more than one paternal HLA-C per trophoblast cell is induced. In oocyte donation cycles, an increasingly demanded treatment due to advanced maternal age, the oocyte-maternal HLA-C, which is genetically different from the mother’s receptor, behaves as a paternal HLA-C and this induces that more nonself HLA antigens are presented to the mother’s KIR (per transfer) compared with “normal” pregnancies.
When donor oocytes or embryos are used, the embryo shares no maternal “self” genes, as both sets of chromosomes are derived from “nonself” individuals. In other words, the oocyte HLA-C allele is genetically different from the surrogate mother’s HLA-C alleles, and thus the oocyte HLA-C allele represents an additional “paternal” or nonself HLA-C, increasing the number of foreign HLA-C alleles to be faced by the mother immune uterine cells.
Previous research has highlighted the risk for women and their babies in oocyte donation pregnancies. Consistent findings are the increased risk of pregnancy-induced hypertension and other great obstetrical syndromes (preeclampsia and fetal growth restriction, FGR), even when controlling for age and singleton pregnancies.
For the first time in 2014 (16), our group reported a significantly decreased LBR per cycle after a DET with donated oocytes in KIR AA patients when compared to KIR AB and KIR BB.
The increased expression of paternal HLA-C after DET is associated with more pregnancy disorders than single embryo transfer (SET) in mothers with an inhibitory KIR haplotype (AA).
The decreased LBR after DET in donor oocyte cycles in mothers KIR AA may be due to increased expression of nonself HLA-C (paternal and oocyte donor HLA-C). In this case 4 “paternal” HLA-C per trophoblast cells/DET: 1 paternal and 1 oocyte donor HLA-C per trophoblast cell and embryo since oocyte donor HLA-C behaves as “paternal” nonself HLA-C. Expressing 4 “paternal” HLA-C is more likely to find at least one paternal or oocyte donor HLA-C2 than in own oocytes and SET, and probably implantation or placentation failure occurs in mothers who are KIR AA.
This new findings show that the maternal KIR haplotype and fetal HLA-C have an impact on the live birth rate after IVF cycles, especially when donor oocyte and DET are used. Expressing four paternal HLA-C in the EVT cells after DET with donor oocytes is more likely to result in at least one nonself HLA-C2 (even by HLA-C2 allelic frequency on Caucasian population) than with one’s own oocyte after SET, and implantation or placentation failure probably occurs in mothers with the KIR AA haplotype. Therefore, selecting HLA-C1 among oocyte and/or sperm donors for patients undergoing egg donation and inhibitory KIR haplotypes could be more efficient and safer (17).
The combination of maternal KIR haplotype and parental, donors HLA-C, could predict which couple can benefit for the selection of SET/DET, or donor selection by HLA-C in ART, in order to increase the LBR/cycle and it would facilitate the reduction of embryos that are being transferred, facilitating the increase of SET with an impact over pregnancy complication (preeclampsia, FGR, etc.) too. Therefore, selecting HLA-C1 among oocyte and/or sperm donors for patients undergoing to egg donation ART and inhibitory KIR, could be more efficient and safer as identified by epidemiological studies (15, 19, 20) (see also Figures 10.1 and 10.2).
Figure 10.1 Maternal–fetal immune recognition in own oocytes single embryo transfer. (A) Correct activation through maternal KIR with a matched fetal HLA-C. (B) Inhibitory KIR signals after a contact with a mismatch fetal HLA-C.

Full access? Get Clinical Tree
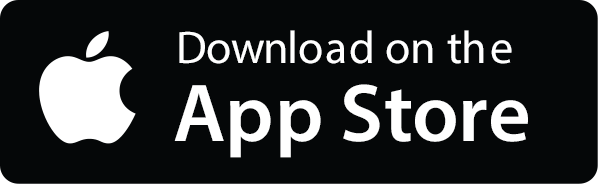
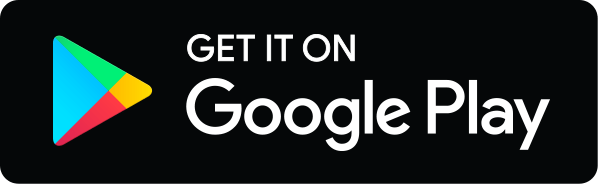