Abstract
This chapter describes techniques for the preparation of human spermatozoa for assisted conception. Traditional swim-up techniques depend on the intrinsic motility of human spermatozoa and have been used as the basis for new microfluidics devices that exploit the capacity of these cells for chemotaxis, rheotaxis or thermotaxis. Unfortunately, such self-migration strategies tend to lose effectiveness with pathological samples exhibiting poor levels of motility and/or diminished counts. With such compromised starting material, active selection of spermatozoa can be achieved by exploiting differences between sperm populations in their density or charge. Density gradient centrifugation selects spermatozoa with minimal residual cytoplasm that are highly motile and have good morphology, even though the induction of sperm DNA damage in certain patients may be problematical. Alternatively, methodologies based on differences in sperm charge, appear to hold promise for the rapid, efficient isolation of highly motile human spermatozoa that consistently exhibit low levels of DNA damage.
1.1 Introduction
One of the great challenges in assisted conception is to ensure that only the highest quality gametes are selected for fertilization. This is necessary not only to optimize the chances of successful conception but also to ensure the normality of any offspring generated as a consequence. In our species, around 200 million spermatozoa are normally released into the female reproductive tract at insemination and, in the journey that follows, all but a small minority of these cells will perish. The subpopulation of spermatozoa that reaches the surface of the oocyte in the ampullary region of the fallopian tubes in vivo is therefore highly selected. The selection process appears to be dependent on the intrinsic motility of the spermatozoa and their capacity to evade detection by the host’s immune system. The outcome is to select spermatozoa that are highly motile, are morphologically normal and exhibit a clear ability to express all of the features of a capacitated cell, including hyperactivation and the ordered expression of receptors on the sperm surface for the zona pellucida. The selected spermatozoa are also characterized by high levels of DNA integrity, as befits a cell charged with the responsibility of transferring an intact paternal genome onto the next generation [1].
Sperm selection in vivo is an extremely sophisticated process involving changes in the pattern of motility, the creation of isthmic reservoirs, chemotaxis, thermotaxis, rheotaxis, complex interactions with the extracellular matrix and changes in the sperm surface expression of receptors and proteases. In the context of assisted reproductive technology (ART), an important aim is to replicate this complex sperm selection cascade, so that gametes selected in vitro for the purpose of fertilization will reflect those selected in vivo. This selection process is particularly important in the context of intracytoplasmic sperm injection (ICSI), which involves the physical injection of an individual spermatozoon into the ooplasm of the egg. This procedure is particularly forgiving of defects in sperm quality, with the result that even severely damaged spermatozoa possessing high levels of DNA damage can still achieve fertilization, if ICSI is used as the insemination protocol [2]. With IVF, the situation is less critical because the zona pellucida itself acts as a filter that will exclude any spermatozoon that does not possess the qualities of movement needed to achieve penetration of the zona matrix, has not successfully engineered the expression of zona receptors on the sperm surface or has not proven capable of acrosomal exocytosis once zona recognition has occurred [3]. However, even with IVF as the insemination procedure, the spermatozoa have not been subjected to the discrimination that normally occurs at the levels of the cervix and isthmic region of the fallopian tubes and, in mouse infertility models, this is frequently where sperm selection occurs [4].
1.2 Principles of Sperm Isolation: Preventing Leukocytic Attack
Human semen is an extremely complex cellular mixture containing live and dead spermatozoa, leukocytes (largely neutrophils), precursor germ cells, bacteria and cellular detritus originating from the secondary sexual glands. The clinical challenge is to extract the high-quality spermatozoa from this complex mélange, without inducing any iatrogenic damage. In this context, seminal plasma is our friend because it is richly endowed with antioxidants specifically designed to protect the spermatozoa during their short journey from the male reproductive tract into the female. These antioxidants include small molecular mass scavengers such as vitamin E, glutathione, uric acid and taurine as well as highly specialized antioxidant enzymes including catalase, glutathione peroxidase and superoxide dismutase [5]. This complex antioxidant mixture has evolved because the spermatozoa are very vulnerable to oxidative attack during the insemination process – and this attack may come from a variety of sources. In this context it is important to remember that spermatozoa have been matured and stored in the epididymal lumen, which is essentially free of phagocytic leukocytes capable of generating reactive oxygen species (ROS). Then, at the moment of ejaculation, the spermatozoa are suddenly exposed to macrophages and neutrophils that have infiltrated the ejaculate via the secondary sexual glands and urethra. These phagocytes are in an activated free-radical-generating state and would cause considerable damage to the sperm plasma membrane and other structures if it were not for the antioxidants in seminal plasma providing a high level of free radical scavenging protection (Figure 1.1) [6].
Figure 1.1 Human semen samples are invariably contaminated with leukocytes, particularly neutrophils, which are capable of generating reactive oxygen species (ROS) and damaging the spermatozoa. (A) Leukocytes in semen (arrowed) stained with an anti-CD45 antibody. (B) The concentration of leukocytes in human semen correlates very closely with ROS generation by the unfractionated ejaculate, indicating that a significant proportion of the seminal leukocyte population is in an activated state. (C) Using opsonized zymosan to investigate the presence of ROS-generating phagocytes in sperm samples prepared for IVF reveals that electrophoretically separated sperm suspensions (E-separated) possess lower levels of leukocyte contamination than those prepared by discontinuous gradient centrifugation (DGC) (Percoll). Following electrophoresis, most of the leukocytes remain trapped in the inoculation chamber (Residual) [6].
Very soon after insemination, the best quality spermatozoa with the highest levels of progressive motility leave the protection provided by seminal plasma and colonize the cervix. In this location the spermatozoa are safe – for a while. Within a few hours the presence of spermatozoa and semen in the cervix and vagina stimulates a leukocytic infiltration, largely neutrophils, designed to phagocytose any dead or moribund spermatozoa remaining at the site of insemination. The post-insemination phagocytosis of non-viable spermatozoa is generally “silent” in the sense that no ROS or pro-inflammatory cytokines are generated. The silent phagocytosis of senescent spermatozoa is thought to be a response to markers, such as phosphatidylserine (PS), which are expressed on the surface of spermatozoa as they engage in the intrinsic apoptotic cascade. This concept has arisen by analogy to silent phagocytosis in somatic systems, in which the expression of apoptotic markers such as PS on the surface of phagocytosed cells informs the phagocyte that engulfment must not be accompanied by an oxidative burst. Such silent phagocytosis is widespread in biology and can be seen, for example, when senescent neutrophils are being removed by macrophages from sites of tissue repair [7]. The silent nature of this phagocytic process ensures that under normal physiological circumstance, the spermatozoa do not have to contend with additional oxidative stress while they ascend the female reproductive tract. However, if surface expression of PS does not occur because the spermatozoa have died a necrotic death mediated by peroxynitrite [8] or attack by spermicidal detergents such as nonoxynol-9, then they may well be exposed to a post-insemination oxidative attack, only this time they will not be able to rely on the antioxidant properties of seminal plasma to protect them.
The protective role of seminal plasma is an important fundamental concept that has helped design optimized sperm isolation strategies. Whatever sperm preparation approach is used, it is important that seminal leukocytes and spermatozoa are not allowed to come into contact with each other in the absence of seminal plasma. When this is permitted to occur, as when spermatozoa are prepared by repeated cycles of washing and centrifugation or are swum up from a washed pellet, then sperm quality is invariably compromised [9]. This can occur inadvertently, if the sperm preparation procedure has not been successful in removing all of the contaminating leukocytes from the suspension used for IVF. Thus, in one study involving the use of discontinuous gradient centrifugation (DGC) to carefully purify spermatozoa for an IVF program, leukocyte contamination could still be demonstrated in 28.5% of the sperm preparations. Furthermore, the presence of these contaminating cells was associated with elevated levels of spontaneous ROS production, impaired movement, and a significantly reduced capacity for fertilization in vitro [10]. Treatment of such human sperm suspensions with magnetic beads or ferrofluids coated with antibodies against the common leukocyte antigen (CD45) has been found to successfully remove these cellular contaminants and, by so doing, significantly enhance the fertilizing capacity of the remaining spermatozoa (Figure 1.2) [11].
Figure 1.2 When leukocyte contamination is observed in human sperm suspensions, these cells can be removed, resulting in a reduction in oxidative stress and an increase in sperm function. (A) Principle behind the leukocyte separation procedure using magnetic beads coated with antibodies against the common leukocyte antigen (CD45). (B) The treatment is extremely effective in removing residual leukocytes from sperm suspensions. (C) The removal of contaminating leukocytes results in a massive reduction in oxidative stress as determined by the chemiluminescent measurement of ROS generation. (D) Leucocyte removal results in an increase in levels of sperm–oocyte fusion as observed with the hamster oocyte penetration assay [11].
It follows from the above that any strategy for isolating spermatozoa for ART purposes should take its lead from nature and isolate the cells directly from semen rather than a washed sperm suspension. Given that this is the case, there are a limited number of strategies that can be pursued in order to achieve the effective isolation of high-quality spermatozoa from the ejaculate based on sperm motility, density, charge and other surface characteristics. In the sections that follow, we shall consider the effectiveness of these approaches and their potential for application in a clinical setting.
1.3 Intrinsic Motility
Because our species is a vaginal inseminator, we generate spermatozoa that are capable of progressive linear movement capable of penetrating the dense extracellular matrices that characterize the female reproductive tract, beginning with cervical mucus. If we wish to emulate nature in developing optimized methods for isolating high-quality spermatozoa, we could do worse than develop a medium resembling cervical mucus for the spermatozoa to colonize. In this context, cervical mucus itself, whether of human or bovine origin, is too logistically difficult to obtain and too difficult to standardize for routine sperm isolation purposes. Recognizing this, scientists in the early 1990s started to experiment with cervical mucus substitutes for the isolation of spermatozoa and discovered that hyaluronate polymers were extremely effective in this regard [12]. Furthermore, spermatozoa that have been isolated using hyaluronate solutions have been shown to be functionally normal as judged by their motility and ability to undergo both capacitation and the acrosome reaction [13]. Swim-up procedures involving the layering of a hyaluronate solution over human semen, followed by an incubation period of 50–60 minutes at 37ºC in an atmosphere of 5% CO2 in air, have been reported to generate sperm suspensions that are of significantly higher quality than those prepared by swim-up following centrifugation [14]. As a simple inexpensive technique for sperm isolation, the self-migration of spermatozoa directly from semen into medium that has been modified by the addition of sodium hyaluronate in order to increase its viscosity and limit contamination of the sample with seminal plasma constituents, has much to commend it. A variation on this theme has been a double-up technique in which spermatozoa are swum up from a pellet previously prepared by DGC [15]. Such a technique generates sperm suspensions of high purity and quality, although preparation time is prolonged and success ultimately depends on the intrinsic quality of the original semen sample in terms of motility and count.
1.4 Microfluidic Devices
The intrinsic motility of spermatozoa has also been exploited in the generation of microfluidic systems for sperm isolation that employ media based on hyaluronate or occasionally methylcellulose for the initial isolation of the spermatozoa [16]. A variety of such microfluidic systems have been developed that attempt to replicate the mechanisms by which spermatozoa are isolated in vivo. Most of these devices feature carefully engineered microchannels that spermatozoa colonize in the same way as they colonize cervical mucus by virtue of their own motility. Orientation of the spermatozoa in such channels has been achieved by a variety of different mechanisms, depending on such properties as rheotaxis, chemotaxis or thermotaxis, all of which spermatozoa display.
Rheotaxis, for example, refers to the orientation of spermatozoa in a fluid flow. In some respects, spermatozoa are like trout, in that faced with a mild flow in the extracellular environment, they will orientate upstream. Of course, unlike trout, spermatozoa do not possess a lateral line to detect the flow but rather depend on complex hydrodynamic interactions to achieve the upstream orientation [16]. The rate of flow is critical because if it becomes too strong the spermatozoa will simply be swept away. However, a carefully engineered flow of around 15–100 µm/s can facilitate the migration of spermatozoa through the device. Building on these fundamentals, an ingenious microfluidic corralling system has recently been developed for isolating subpopulations of highly motile human spermatozoa [17].
Chemotaxis is another potential mechanism for orientating spermatozoa, particularly for microfluidics systems that aim to not only isolate the spermatozoa but also fertilize the oocyte and culture the embryo. Some candidates for human sperm chemotaxis have already been identified, notably progesterone, and used as the basis for creating a sperm isolation system. In studies with this device, spermatozoa were initially separated on Percoll gradients and then inoculated into a system that mimicked the dimensions of the human female tract [18]. After a prolonged 150-min incubation at 37ºC, spermatozoa attracted by the presence of a progesterone gradient were shown to have significantly better morphology and significantly reduced levels of DNA damage compared with spermatozoa isolated by virtue of their motility alone. Although the incubation time used in this study was protracted and the recovery efficiency low, the general principle of using chemotaxis as an aid to sperm isolation certainly has merit. Clearly, species exhibiting an internal mode of fertilization exhibit nothing like the strong chemotaxis evident in aquatic species where fertilization is external and conspecific gametes have to quickly find each other before becoming diluted to infinity in the water column. Nevertheless, there is strong evidence for the involvement of chemotaxis in human fertilization as suggested by the synthesis and insertion of olfactory receptors in the sperm plasma membrane [19]. Although progesterone is evidently a player in this context, there are other possibly more powerful chemotactic factors elaborated by the oocyte that still await definitive characterization [20]. Further resolution of such factors may greatly assist in the development of sperm isolation systems that reflect the in vivo situation.
Along similar lines, microfluidic devices have also been constructed based on the principle of thermotaxis and exploiting the fact that spermatozoa move along a temperature gradient as they ascend the female reproductive tract. Although the mechanisms that unpin this thermotaxis are currently unresolved, the capacity of these cells to discriminate changes in temperature has been analysed in detail with some astonishing results. According to Bahat and colleagues [21], human spermatozoa can sense and respond to a temperature difference of less than 0.0006°C! Using this principle, a novel microfluidics device has been constructed that appears to be effective in trapping spermatozoa that have migrated into regions of elevated temperature [22].
To date, there are no reports of how such microfluidic devices perform with pathological samples under real-life clinical laboratory conditions. The implementation of such studies will be important not just to determine whether microfluidics systems have any practical potential in a clinical context but also to determine whether such systems could be used as the initial component of a microfluidics system that will not only capture high-quality spermatozoa but also support fertilization and the early stages of embryo development – IVF on a chip. Using donated frozen-thawed human embryos, microfluidic devices have been assessed that appear to provide the same level of support for preimplantation embryonic development as conventional embryo culture methodologies [23]. This is clearly an extremely active area of research at the present time, which envisions development of a single microfluidics device that can achieve isolation of the highest quality spermatozoa, fertilization and the detailed monitoring of preimplantation development with a minimal degree of involvement on behalf of the embryologist overseeing the process. One could even imagine systems where the embryo culture fluid is automatically sampled and scanned for markers that will confirm their euploid status prior to being selected for transfer [24].
The difficulties with such a highly technical automated approach to IVF are cost, time and, ultimately, feasibility. The capacity to troubleshoot any unexpected problems that arise during the sperm isolation–fertilization–development continuum may be curtailed if the process becomes too automated and, at the end of the day, the embryo will still have to be recovered and manually transferred into the uterine cavity – and this comprises one of the most difficult risky phases of the entire ART process. Furthermore, the sperm selection component of such devices depends for its success on the intrinsic motility of the spermatozoa. In an IVF program where many of the semen samples that are being processed for treatment will be of poor quality and frequently suffering from low levels of motility, the ability of spermatozoa to self-select may be impaired. In order to compensate for the poor intrinsic motility of pathological sperm samples, alternative sperm separation strategies have been developed that actively recruit the spermatozoa rather than relying on their capacity to self-select. The two principle properties on which such selections have been based are sperm density and sperm charge.
1.5 Isolation According to Density
One of the features of mammalian spermatozoa is that they have a relatively high density by virtue of their lack of cytoplasmic space. Hence, if these cells are placed in a continuous density gradient and centrifuged, the highest quality cells with the greatest isopycnic densities will be carried to the densest region of the gradient [25]. Such DGC techniques have been progressively simplified with the passage of time and, in minimal two-step mode, have proven highly effective in isolating motile spermatozoa exhibiting high levels of fertilizing potential capable of establishing viable pregnancies following IVF [26].
The positioning of spermatozoa in such discontinuous gradients is a function of their density and thus, inversely, their volume. High-quality spermatozoa are the product of a highly efficient spermatogenic process that effectively removes most of the residual cytoplasm from the spermatozoa just prior to their release from the germinal epithelium. Any residual cytoplasm then snaps back into the midpiece of the cell to create a cytoplasmic remnant that, as far as we are aware, is not further processed by the cell. The retention of excess residual cytoplasm in this manner is in contrast to many other mammalian species where any cytoplasm remaining with the spermatozoon after spermiation is concentrated into a rounded cytoplasmic droplet which then slips down the shaft of the sperm tail to the annulus, where it is discarded or resorbed. Human spermatozoa do not behave in the same way. The retention of excess residual cytoplasm by human spermatozoa is a pathological change that inversely reflects the quality of the spermatogenic process involved in their creation and thus, ultimately, their functionality (Figure 1.3). The more cytoplasm is retained by the spermatozoa the worse their motility, the poorer their capacity for acrosomal exocytosis and sperm–oocyte fusion and the higher the levels of ROS generation [27].

Full access? Get Clinical Tree
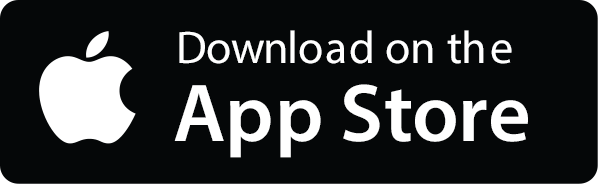
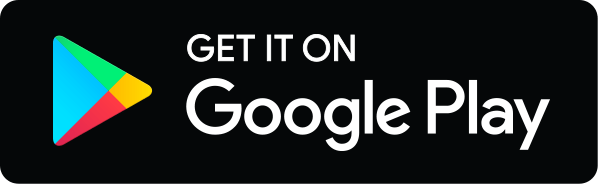