Objective
The objective of this study was to compare histological and biochemical features of the (normal) precervical anterior vaginal wall and the prolapsed anterior vaginal wall of women with pelvic organ prolapse (POP). These data were compared to tissue of the precervical anterior vaginal wall of age-matched controls without POP to identify possible intrinsic and acquired effects.
Study Design
Biopsies were collected from the apex of the anterior vaginal cuff after hysterectomy from a control group of 13 premenopausal women undergoing hysterectomy for benign gynecological diseases, and a case group of 13 premenopausal women undergoing prolapse surgery (cystocele POP-Quantification stage ≥2). In women with POP an additional full-thickness vaginal wall sample was taken from the POP site during anterior colporrhaphy. Histomorphometric and biochemical analysis were performed for different components of the extracellular matrix.
Results
There were no differences between case and control group in the precervical vaginal wall tissue with respect to the different components of the extracellular matrix and the biochemical parameters. However, there was a tendency toward a higher amount of collagen III and elastin, and a significant increase of smooth muscle cells and pyridinoline collagen cross-links in the POP site compared to the non-POP site of the same POP patient.
Conclusion
Our findings suggest that the changes seen in connective tissue in the anterior vaginal wall of women with POP are the effect, rather than the cause, of POP.
Pelvic organ prolapse (POP) is a global health problem, affecting adult women of all ages. It decreases their quality of life considerably. POP is one of the most common reasons for gynecological surgery in women after the fertile period. The failure rate of surgery is relatively high: an estimated 30% of women require reoperation. Despite this, little is known about the underlying pathophysiology of POP.
For decades, it has been speculated that POP occurs due to a structural defect in the vagina and its supportive tissues. Such defects could be a decrease in collagen content and quality, differences in collagen subtypes, changes in the amount and quality of elastin, and the density of smooth muscle cells (SMCs). Due to different biopsy sites, lack of clarity as to which layers of the vaginal wall are actually being analyzed, and very heterogeneous study populations, data are conflicting. Moreover, by comparing tissue that is prolapsed to one that is not prolapsed, it is impossible to distinguish between causes and effects of POP. In other words, it is impossible to distinguish whether the observed changes in the anterior vaginal wall are the result of an intrinsic (genetic) or an acquired (environmental) effect.
The goal of this study was to identify the changes in the nonprolapsed anterior vaginal wall that occur in premenopausal women with POP, compared to age-matched women without POP using histologically defined samples. The second goal was to determine whether the observed changes are caused by the prolapse, by comparing the histological and biochemical features of the anterior vaginal wall at the site of the POP with the same features at the non-POP site within the same patient. This approach helps us to identify possible changes in the connective tissue of the vaginal wall due to the increased pressure and stretch of the prolapsing pelvic organs on the vaginal wall. We hypothesize that the precervical anterior vaginal wall (non-POP site) of women with POP is comparable to the precervical anterior vaginal wall of controls, thus allowing us to consider this precervical tissue in POP patients as a proper control site. We expect to find differences in the histological and biochemical features at the POP site compared to the non-POP site within the same woman with prolapse. By testing the hypothesis that the changes seen in the connective tissue of the anterior vaginal wall are an acquired rather than an intrinsic effect causing POP, we hope to provide useful information that should help to develop new approaches in reconstructive pelvic surgery.
Materials and Methods
Patient population
Upon medical ethical committee approval and acquired informed consent, biopsies were collected from 26 Caucasian premenopausal women at the Department of Obstetrics and Gynecology of the Kennemer Gasthuis, Haarlem, The Netherlands, from March 2009 through March 2011. Eligible women were divided into 2 groups: a control group of 13 women undergoing abdominal or laparoscopic hysterectomy for benign gynecological diseases with no sign of POP during gynecological examination, and a case group of 13 patients undergoing vaginal hysterectomy and reconstructive pelvic surgery of the anterior vaginal compartment because of a cystocele, POP-Quantification (POP-Q) stage ≥2. Signs of POP were recorded during the pelvic examination by the same urogynecologist (M.H.K.) and were described according to the International Continence Society POP-Q. Groups were matched for age, parity, smoking, and use of oral contraceptives. Premenopausal status was defined as having a regular period over the preceding 12 months. Women on oral contraception were asked to temporarily stop for 3 months to see whether a spontaneous regular menstrual cycle would occur. Endometrial biopsies were obtained from all women defining the stage of the menstrual cycle at time of operation. Exclusion criteria included the use of progestin-only hormone regimen, a history of pelvic surgery, pelvic malignancy or connective tissue disease affecting collagen or elastin remodeling, adhesions or scarring at the biopsy site, surgeons judgment that a biopsy may harm the patient, a history or presence of endometriosis, morbid obesity (body mass index >35 kg/m 2 ), diabetes, chronic inflammatory disease, chronic infections, steroid use, and inability to provide informed consent. Standardized demographic and pertinent clinical information was recorded prospectively and stored in a dedicated database.
Tissue acquisition and preparation
The site of tissue collection was standardized because of potential differences in composition of the extracellular matrix (ECM) throughout the vagina. After removal of the uterus in the controls, full-thickness samples of the anterior vaginal wall were obtained from the vaginal cuff at the anterior midline portion of the vaginal wall. In women with POP, an additional full-thickness anterior vaginal wall (midline) sample was taken from the POP site during anterior colporrhaphy (point Ba POP-Q).
To minimize harm in the control subjects, only anterior vaginal wall tissue from the vaginal cuff was retrieved. The minimum size of the biopsy was 0.5 × 1.0 cm 2 . All biopsies were large enough to perform (immuno-)histochemical as well as biochemical analysis. Biopsies were immediately passed off the surgical field and divided into 2 parts. For biochemical analysis, the biopsies were washed in phosphate-buffered saline solution and stored at –80°C until further processing. For analysis by microscopy, the collected tissue was fixed in neutral-buffered formalin for 24 hours, dehydrated, and processed into paraffin blocks. Serial 3-μm sections were cut from the paraffin blocks, mounted on slides, and stored until further processing.
All measurements were performed without knowledge of sample identity.
(Immuno-)histochemistry
Hematoxylin-eosin staining was performed to verify that the collected samples represented the vaginal wall containing the epithelial layer, connective tissue, and muscularis. To detect the amount of elastin in the connective tissue, the Lawson elastic van Gieson stain kit, purchased from Klinipath (Duiven, The Netherlands), was used. As positive controls, tissues from lung, appendix, and liver were used.
Monoclonal antibodies against desmin and CD31 were used to identify SMCs and blood vessels, respectively. Sections were also immunostained for the ECM proteins collagen I, III, and IV ( Table 1 ).
Antibody | Company | Preparation | Titre | Positive control |
---|---|---|---|---|
Mouse antihuman monoclonal antibody desmin | DAKO, Copenhagen, Denmark | Peroxidase block | Ready-to-use antiserum | Appendix |
Mouse antihuman monoclonal antibody CD31 | DAKO, Copenhagen, Denmark | Peroxidase block | Ready-to-use antiserum | Tonsil |
Rabbit antihuman collagen type I | ABNOVA, Heidelberg, Germany | Citrate pH 6.0 | 1:100 | Kidney |
Rabbit antihuman collagen type III | ACRIS, Herford, Germany | Pepsin 0.1% | 1:1000 | Skin |
Mouse antihuman IgG1 collagen type IV | DAKO, Copenhagen, Denmark | Citrate pH 6.0 | 1:150 | Skin |
Rabbit antihuman collagen type V | ACRIS, Herford, Germany | Pepsin 0.1% | 1:1000 | Skin |
Morphometric analysis
To quantify SMCs, elastin, and microvessels we performed a morphometric analysis. Complete slides were scanned with a digital Mirax slide scanner system (3DHistech Ltd, Budapest, Hungary) equipped with a ×20 objective with a numerical aperture of 0.75 and a Sony DFW-X710 Fire Wire 1/3, type progressive scan CCD camera pixel size 4.65 × 4.65 um (3DHistech Ltd) (pixel size 4.65 × 4.65 μm). The actual scan resolution of all pictures at ×20 was 0.23 μm. After scanning, representative areas of both the muscularis layer and the lamina propria layer between 0.55-0.64 mm 2 were randomly annotated by hand using Panoramic Viewer software (3DHistech Ltd). Resulting annotations were exported in the tagged image file format (tiff) image format. A computerized morphometric analysis of the desmin-, elastic van Gieson–, and CD31-stained slides was executed, using ImageJ 1.44p software ( http://rsbweb.nih.gov/ij/ ) with a modified macro from Hadi et al. Analysis was performed for the lamina propria and muscularis layer separately. The amount of SMC was expressed as the total area of desmin-positive cells vs the total tissue area. The amount of elastin was expressed by total area of fibers vs total tissue area. The CD31 staining was used to quantify the amount of microvessels per area as well as the amount of nuclei per area.
Collagen staining was quantified in a blinded fashion using a 4-grade scoring system (absent, light, moderate, or strong) by 2 independent pathologists ( Appendix , Supplementary Figure ). Slides were scored using a standard light microscopy technique with magnification of ×100. An interrater reliability analysis using the Kappa statistic was performed to determine consistency between the 2 pathologists and was excellent (Kappa 0.97; P < .001).
Biochemical analysis
The epithelial layer of the vaginal wall biopsies was removed under a dissecting microscope. The remaining tissues were freeze-dried and weighed. Samples were hydrolyzed with 6 mol/L hydrogen chloride at 110°C for 20 hours. The amount of hydroxyproline and proline was determined by reverse-phase high-performance liquid chromatography (HPLC) of 9-fluorenylmethyl chloroformate-(Fluka, Buchs, Switzerland) derivatized amino acids, as described by Bank et al. Collagen content was calculated assuming 300 residues hydroxyproline per triple helix and a molecular weight of 300,000 g/mol. The hydroxyproline/proline ratio was determined by dividing the hydroxyproline content per mmol/L by proline content per mmol/L, resulting in a dimensionless ratio.
For the determination of the collagen cross-links lysylpyridinoline and hydroxylysylpyridinoline, acid-hydrolyzed samples were diluted to 50% acetic acid and injected on an HPLC system equipped with online sample purification on CC31 cellulose using a Prospekt solid-phase extractor (Separations, Jasco Benelux BV, IJsselstein, The Netherlands). The retained cross-links were eluted from the CC31 material and online chromatographed on a cation exchange column (Whatman Partisil SCX; Fisher Scientific, Waltham, MA). Eluting cross-links were detected by a Jasco fluorometer (model FP-920; Separations). The PYD/DPD HPLC calibrator (Metra, Palo Alto, CA) was used as standard. Values are expressed as total amount of residues per collagen molecule.
Statistics
The primary outcome of the study was to detect a difference in the amount of total collagen (hydroxyproline) or the amount of collagen type III in patients with and without POP. Secondary outcome measurements included a change in the amount of collagen I and IV, the amount of elastin and SMC, the concentration of non-collageneous proteins, and the maturity of the cross-links within the collagen fibrils in patients with and without POP. Based on previous studies 10 women were required in the POP and in the control group to detect a difference of at least 10% in the group-specific amounts of total collagen and collagen type III for a power of 80% and a .05 significance level, using a 1-way analysis of variance. The final sample size was increased with at least 10% due to the use of nonparametric tests.
All statistical calculations were performed using statistical software (SPSS 19.0; IBM Corp, Armonk, NY). Differences between continuous variables were identified using the Mann-Whitney U test and differences between categorical variables were identified using Pearson χ 2 test, Fisher exact test, or likelihood ratio according to the expected cell size and number of degree of freedom. Comparison on paired samples was performed by using Wilcoxon signed ranks test. Results are expressed as mean ± SD for continuous variables and as median and interquartile range for the ordinal variables. All statistical tests were 2-sided and differences were considered statistically significant when P value was < .05.
Results
Demographics
In this study, patient selection and matching were very strict ( Table 2 ). Furthermore, there was no difference in stage of menstrual cycle or in risk factors for POP such as heavy lifting, chronic obstructive pulmonary disease, or mean weight of first child and largest child between the groups. Stress urinary incontinence was more common in the POP group ( P < .004) ( Table 2 ). In the POP group the median stage of cystocele was 3 (range, 2–3). Six women had a cystocele stage II, defined as a mild POP. Seven women had severe POP with a cystocele POP-Q stage 3. The median stage of prolapse in the apical compartment and posterior compartment was 0 (range, 0–1) and 1 (range, 0–3), respectively. Within the control group 1 sample was lost before immunohistochemical analysis was performed, therefore the data of 12 controls were analyzed.
Characteristics | Control (n = 12) | Case (n = 13) | P value |
---|---|---|---|
Age, y a | 44.5 ± 5.0 | 42.9 ± 6.0 | .512 d |
BMI, kg/m 2 a | 24.5 ± 1.7 | 27.2 ± 6.0 | .574 d |
Parity, births b | 2.2 (1–3) | 2.2 (1–4) | .852 d |
Vaginal delivery b | 2.2 (1–3) | 2.2 (1–4) | .820 d |
Assisted vaginal delivery c | 4 (36) | 4 (31) | 1.000 e |
Smoking status c | |||
Never smoked | 3 (25) | 4 (31) | 1.000 e |
Current | 4 (33) | 3 (23) | .673 e |
Previous | 5 (42) | 6 (46) | .821 f |
Oral contraception use c | |||
Until surgery | 4 (33) | 4 (31) | 1.000 e |
Never used | 1 (8) | 1 (8) | 1.000 e |
Stress urinary incontinence c | 1 (8) | 9 (69) | .004 e |
POP-Q stage of prolapse b | |||
Anterior | 0 (0) | 3 (2–3) | |
Apical | 0 (0) | 0 (0–1) | |
Posterior | 0 (0) | 1 (0–3) |
b Data presented as median (interquartile range)
c Data presented as number of patients (%)
(Immuno-)histochemical analysis
Examination of hematoxylin-eosin–stained vaginal biopsies using standard light microscopy techniques confirmed that all layers were present in each biopsy ( Figure 1 ). There was no evidence of inflammation in the subepithelial stroma.

Comparing the lamina propria and muscularis layer of the non-POP site of the POP group with the control group, no statistically significant differences were found with respect to the percentage of SMC, microvessel density, and cellularity ( Table 3 ). Also no statistically significant differences were found in the ECM proteins evaluated: elastin and collagens I, III, and IV. Collagen IV was only detected in the basement membrane in all samples. Precervical tissue of women with POP and precervical tissue of controls seem to maintain a comparable amount and distribution of the different components of the ECM.
Specimen muscularis layer | A | B | C | A-B | B-C |
---|---|---|---|---|---|
Control | Case non-POP site | Case POP site | P value c | P value d | |
Desmin staining a | |||||
Mean percent smooth muscle cells | 20.99 (7.34) | 19.47 (7.73) | 26.85 (6.13) | .553 | .016 e |
CD31 staining a | |||||
N nuclei | 922 (233) | 955 (554) | 925 (374) | .908 | .583 |
N microvessels | 51 (14) | 54 (40) | 47 (12) | .402 | .844 |
Elastic van Gieson staining a | |||||
Mean percent elastin | 2.17 (1.12) | 2.44 (1.51) | 3.04 (2.97) | .458 | .859 |
Collagen I b | |||||
Connective tissue | 4 (4–4) | 4 (4–4) | 4 (4–4) | 1.000 | 1.000 |
Muscularis | 2 (1.75–2) | 2 (2–2) | 2 (2–2) | .486 | .157 |
Collagen III b | |||||
Connective tissue | 2 (2–2) | 2 (1–2) | 2 (2–2) | .254 | .180 |
Muscularis | 1 (1–1) | 1 (1–1) | 1 (1–1) | .204 | .546 |
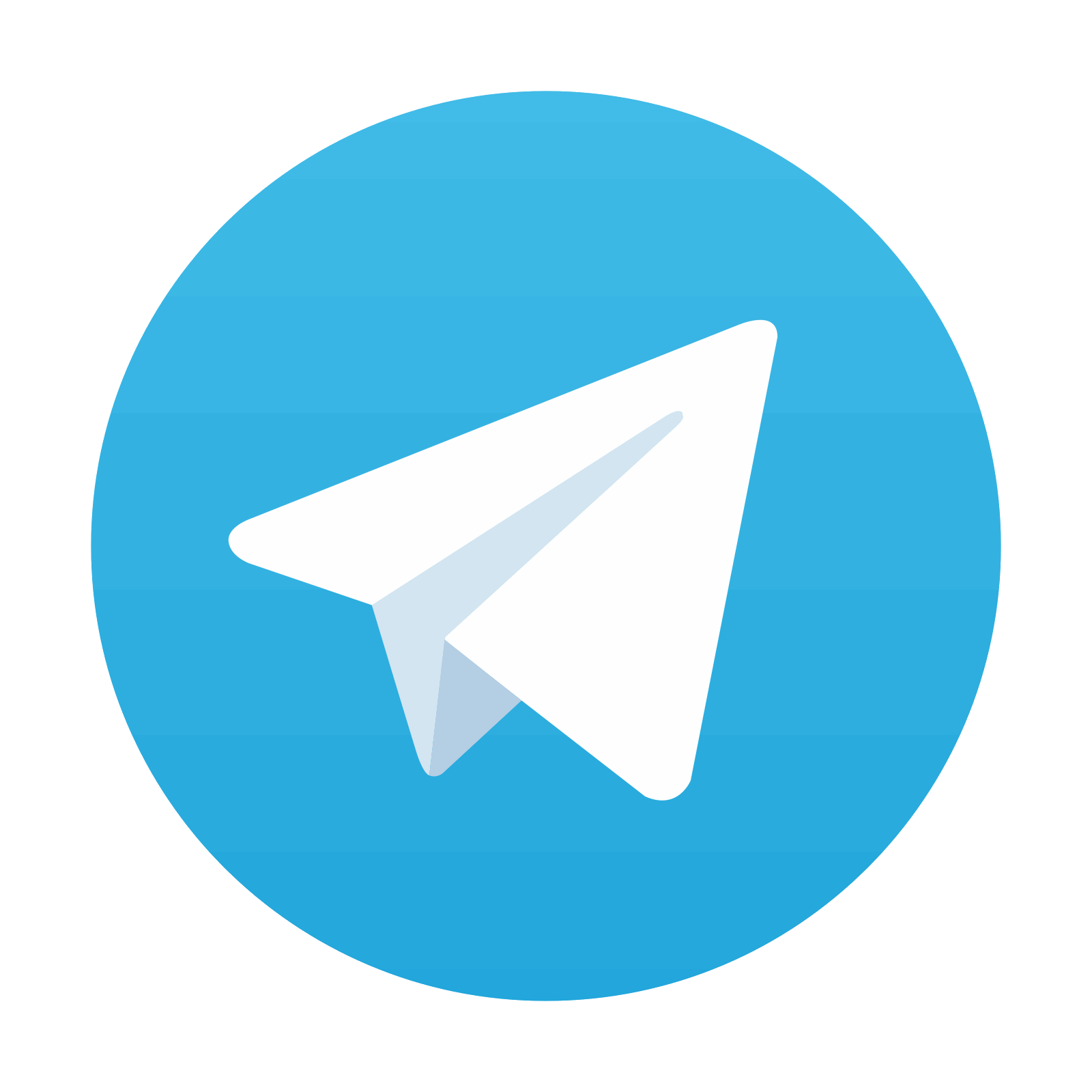
Stay updated, free articles. Join our Telegram channel

Full access? Get Clinical Tree
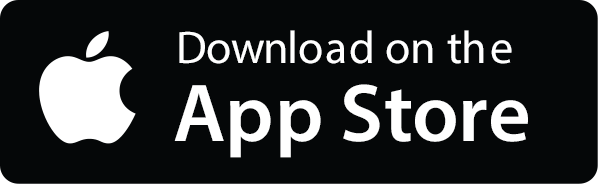
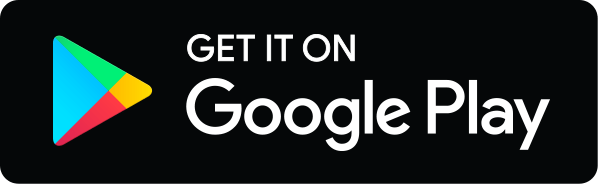
