The cardiovascular system is the first major system to function in the embryo. The primordial heart and vascular system appear in the middle of the third week ( Fig. 13.1 ). This precocious cardiac development occurs because the rapidly growing embryo can no longer satisfy its nutritional and oxygen requirements by diffusion alone. Consequently, there is a need for an efficient method of acquiring oxygen and nutrients from the maternal blood and disposing of carbon dioxide and waste products.

Multipotential cardiac progenitor cells from several sources contribute to the formation of the heart. These include two distinct mesodermal populations of cardiac precursor cells, a primary (first) heart field and a second heart field. Neural crest cells also contribute to the heart. Mesodermal cells from the primitive streak migrate to form bilateral paired strands of the primary heart field . Cardiac progenitor cells from the pharyngeal mesoderm are constituted as the second heart field , which is located medial to the first heart field.
Successive stages in the development of blood and blood vessels (angiogenesis) are described in Chapter 4 , Fig. 4.11 . Primordial blood vessels cannot be distinguished structurally as arteries or veins; however, they are named according to their future fates and relationship to the heart.
Early Development of Heart and Blood Vessels
13
By day 18, the bilateral mesoderm has somatopleure and splanchnopleure components; the latter gives rise to almost all of the heart components. These early endocardial progenitor cells separate from the mesoderm to create paired heart tubes. As lateral embryonic folding occurs, the endocardial heart tubes approach each other and fuse to form a single heart tube (see Figs. 13.7 C and 13.9 C ). Shortening of the endoderm plays an important mechanical role in the formation of the tubular heart. Fusion of the heart tubes begins at the cranial end of the developing heart and extends caudally. The embryonic heart begins to beat at 22 to 23 days ( Fig. 13.2 ). Blood flow begins during the fourth week, and heartbeats can be visualized by Doppler ultrasonography ( Fig. 13.3 ).


A multitude of genes and transcription factors are involved in the development of the mammalian heart, which includes lineage determination, specification of the cardiac chambers, valvuloseptal development, and formation of the conducting system.
Gene expression analysis and lineage tracing experiments suggest that progenitor cells from the pharyngeal mesoderm, located anterior to the early heart tube (anterior heart field) , gives rise to ventricular myocardium and the myocardial wall of the outflow tract. Expression of the Id gene, inhibitor of DNA Binding 1, HLH Protein, is important for the specification of cardiogenic progenitors of the first heart field that will form the early heart tubes . Moreover, another wave of progenitor cells from the pharyngeal mesoderm (second heart field) also contributes to the rapid growth and elongation of the heart tube. The myocardium of the left ventricle and the anterior pole of the heart tube are derived mostly from the second field. Expression of Hes-1 in pharyngeal endoderm and mesoderm (second heart field) plays an essential role in the development of the outflow tract.
The basic helix−loop−helix genes, dHAND and eHAND , are expressed in the paired primordial endocardial tubes and in later stages of cardiac morphogenesis. The MEF2C and Pitx-2 genes, which are expressed in cardiogenic precursor cells emerging from the primitive streak before the formation of the heart tubes (Wnt 3a–mediated), also appear to be essential regulators in early cardiac development.
Development of Veins Associated With Embryonic Heart
Three paired veins drain into the primordial heart of a 4-week embryo (see Fig. 13.2 ):
- •
Vitelline veins return poorly oxygenated blood from the umbilical vesicle.
- •
Umbilical veins carry well-oxygenated blood from the chorionic sac.
- •
Common cardinal veins return poorly oxygenated blood from the body of the embryo to the heart.
The vitelline veins follow the omphaloenteric duct into the embryo. This duct is the narrow tube connecting the umbilical vesicle with the midgut (see Chapter 11 , Fig. 11.1 ). After passing through the septum transversum, which provides a pathway for blood vessels, the vitelline veins enter the venous end of the heart, the sinus venosus ( Fig. 13.4 A , and see Fig. 13.2 ). The left vitelline vein regresses, and the right vitelline vein forms most of the hepatic portal system (see Fig. 13.5 B and C ), as well as a portion of the inferior vena cava (IVC) . As the liver primordium grows into the septum transversum, the hepatic cords anastomose around preexisting endothelium-lined spaces. These spaces, the primordia of the hepatic sinusoids , later become linked to the vitelline veins.


The umbilical veins run on each side of the liver and carry well-oxygenated blood from the placenta to the sinus venosus (see Fig. 13.2 ). As the liver develops, the umbilical veins lose their connection with the heart and empty into the liver. The right umbilical vein disappears during the seventh week, leaving the left umbilical vein as the only vessel carrying well-oxygenated blood from the placenta to the embryo.
Transformation of the umbilical veins may be summarized as follows ( Fig. 13.5 ):
- •
The right umbilical vein and the cranial part of the left umbilical vein between the liver and the sinus venosus degenerate.
- •
The persistent caudal part of the left umbilical vein becomes the umbilical vein , which carries all the blood from the placenta to the embryo.
- •
A large venous shunt, the ductus venosus , develops within the liver (see Fig. 13.5 B ) and connects the umbilical vein with the IVC. The ductus venosus forms a bypass through the liver, enabling most of the blood from the placenta to pass directly to the heart without passing through the developing capillary networks of the liver.
The cardinal veins constitute the main venous drainage system of the embryo (see Figs. 13.2 and 13.4 A ). The anterior and posterior cardinal veins , the earliest veins to develop, drain cranial and caudal parts of the embryo, respectively. They join the common cardinal veins , which enter the sinus venosus (see Fig. 13.2 ). During the eighth week, the anterior cardinal veins are connected by an anastomosis (see Fig. 13.5 A and B ), which shunts blood from the left to the right anterior cardinal vein. This anastomotic shunt becomes the left brachiocephalic vein when the caudal part of the left anterior cardinal vein degenerates (see Figs. 13.4 D and 13.5 C ). The superior vena cava (SVC) forms from the right anterior cardinal vein and the right common cardinal vein.
The posterior cardinal veins develop primarily as the vessels of the mesonephroi (interim kidneys) and largely disappear with these transitory kidneys (see Chapter 12 , Fig. 12.5 F ). The only adult derivatives of these veins are the root of the azygos vein and common iliac veins (see Fig. 13.4 D ). The subcardinal and supracardinal veins gradually develop and replace and supplement the posterior cardinal veins (see Fig. 13.4 A to D ).
The subcardinal veins appear first (see Fig. 13.4 A ). They are connected with each other through the subcardinal anastomosis and with the posterior cardinal veins through the mesonephric sinusoids. The subcardinal veins form the stem of the left renal vein, the suprarenal veins, the gonadal veins (testicular and ovarian), and a segment of the IVC (see Fig. 13.4 D ). The supracardinal veins become disrupted in the region of the kidneys (see Fig. 13.4 C ). Cranial to this region, they are united by an anastomosis that is represented in the adult by the azygos and hemiazygos veins (see Figs. 13.4 D and 13.5 C ). Caudal to the kidneys, the left supracardinal vein degenerates; however, the right supracardinal vein becomes the inferior part of the IVC (see Fig. 13.4 D ).
Development of Inferior Vena Cava
The IVC forms during a series of changes in the primordial veins of the trunk of the body, which occur when blood, returning from the caudal part of the embryo, is shifted from the left to the right side of the body. The IVC is composed of four main segments ( Fig. 13.4 C ):
- •
A hepatic segment derived from the hepatic vein (proximal part of the right vitelline vein) and hepatic sinusoids
- •
A prerenal segment derived from the right subcardinal vein
- •
A renal segment derived from the subcardinal–supracardinal anastomosis
- •
A postrenal segment derived from the right supracardinal vein
Because of the many transformations that occur during the formation of the SVC and IVC, variations in their adult forms may occur. The most common anomaly of the IVC is for its abdominal course to be interrupted; as a result, blood drains from the lower limbs, abdomen, and pelvis to the heart through the azygos system of veins.
Double Superior Venae Cavae
Persistence of the left anterior cardinal vein results in a persistent left SVC ; hence, there are two superior venae cavae ( Fig. 13.6 ). The anastomosis that usually forms the left brachiocephalic vein is small or absent. The abnormal left SVC, derived from the left anterior cardinal and common cardinal veins, opens into the right atrium through the coronary sinus.
Left Superior Vena Cava
The left anterior cardinal vein and common cardinal vein may form a left SVC, and the right anterior cardinal vein and common cardinal vein, which usually form the SVC, degenerate. As a result, blood from the right side is carried by the brachiocephalic vein to the unusual left SVC, which empties into the coronary sinus.
Absence of Hepatic Segment of Inferior Vena Cava
Occasionally, the hepatic segment of the IVC fails to form. As a result, blood from inferior parts of the body drains into the right atrium through the azygos and hemiazygos veins. The hepatic veins open separately into the right atrium.
Double Inferior Venae Cavae
In unusual cases, the IVC inferior to the renal veins is represented by two vessels; usually, the left one is much smaller. This condition probably results from failure of an anastomosis to develop between the veins of the trunk (see Fig. 13.4 B ). As a result, the inferior part of the left supracardinal vein persists as a second IVC.
Pharyngeal Arch Arteries and Other Branches of Dorsal Aortae
As the pharyngeal arches form during the fourth and fifth weeks, they are supplied by arteries, the pharyngeal arch arteries that arise from the aortic sac and terminate in the dorsal aortae (see Fig. 13.2 ). Neural crest cells contribute to the formation of the outflow tract of the heart and to the pharyngeal arch arteries. Initially, the paired dorsal aortae run through the entire length of the embryo. Later, the caudal portions of the aortae fuse to form a single lower thoracic/abdominal aorta. Of the remaining paired dorsal aortae, the right one regresses, and the left one becomes the primordial aorta.

Intersegmental Arteries
Thirty or so branches of the dorsal aorta, the intersegmental arteries, pass between and carry blood to the somites and their derivatives (see Fig. 13.2 ). These arteries in the neck join to form a longitudinal artery on each side, the vertebral artery . Most of the original connections of the arteries to the dorsal aorta disappear.
In the thorax, the intersegmental arteries persist as intercostal arteries . Most of the intersegmental arteries in the abdomen become lumbar arteries ; however, the fifth pair of lumbar intersegmental arteries remains as the common iliac arteries . In the sacral region, the intersegmental arteries form the lateral sacral arteries .
Fate of Vitelline and Umbilical Arteries
The unpaired ventral branches of the dorsal aorta supply the umbilical vesicle, allantois, and chorion (see Fig. 13.2 ). The vitelline arteries pass to the umbilical vesicle and later to the primordial gut, which forms from the incorporated part of the umbilical vesicle. Only three vitelline artery derivatives remain: the celiac arterial trunk to the foregut, the superior mesenteric artery to the midgut, and the inferior mesenteric artery to the hindgut.
The paired umbilical arteries pass through the connecting stalk (primordial umbilical cord) and become continuous with vessels in the chorion , the embryonic part of the placenta (see Chapter 7 , Fig. 7.5 ). The umbilical arteries carry poorly oxygenated blood to the placenta (see Fig. 13.2 ). The proximal parts of these arteries become internal iliac arteries and superior vesical arteries . The distal parts of the umbilical arteries become modified and form the medial umbilical ligaments .
Later Development of Heart
The external layer of the embryonic heart tube, the primordial myocardium , is formed from splanchnic mesoderm surrounding the pericardial cavity (cardiac precursors of the anterior, or second, heart field; Figs. 13.7 A and B and 13.8 B ). At this stage, the developing heart is composed of a thin endothelial tube, separated from a thick myocardium by a gelatinous matrix of connective tissue, cardiac jelly (see Fig. 13.8 C and D ).

The endothelial tube becomes the internal endothelial lining of the heart, or endocardium , and the primordial myocardium becomes the muscular wall of the heart, or myocardium . The visceral pericardium, or epicardium, is derived from mesothelial cells that arise from the external surface of the sinus venosus and spread over the myocardium (see Fig. 13.7 D and F ).
As folding of the head region occurs, the heart and pericardial cavity come to lie ventral to the foregut and caudal to the oropharyngeal membrane ( Fig. 13.9 A to C ). Concurrently, the tubular heart elongates and develops alternate dilations and constrictions (see Fig. 13.7 C to E ): the bulbus cordis (composed of the truncus arteriosus , conus arteriosus , and conus cordis ), ventricle, atrium, and sinus venosus . The growth of the heart tube results from the addition of cells, cardiomyocytes, differentiating from mesoderm at the dorsal wall of the pericardium. Progenitor cells added to the rostral and caudal poles of the heart tube form a proliferative pool of mesodermal cells located in the dorsal wall of the pericardial cavity and the pharyngeal arches.

The truncus arteriosus is continuous cranially with the aortic sac from which the pharyngeal arch arteries arise ( Fig. 13.10 A ). Progenitor cells from the second heart field and cranial neural crest cells contribute to the formation of the arterial and venous ends of the developing heart. The sinus venosus receives the umbilical, vitelline, and common cardinal veins from the chorion, umbilical vesicle, and embryo, respectively ( Fig. 13.10 B ). The arterial and venous ends of the heart are fixed by the pharyngeal arches and septum transversum, respectively. The tubular heart undergoes a dextral (right-handed) looping at approximately 23 to 28 days, forming a U -shaped D-loop (bulboventricular loop) that results in a heart with its apex pointing to the left (see Figs. 13.7 D and E and 13.8 E ). Before the formation of the heart tube, the homeobox transcription factor (Pitx2c) is expressed in the left heart-forming field and plays an important role in the left−right patterning of the heart tube during formation of the cardiac loop. As the primordial heart bends, the atrium and sinus venosus come to lie dorsal to the truncus arteriosus, bulbus cordis, and ventricle (see Fig. 13.10 B and C ). By this stage, the sinus venosus has developed lateral expansions, the right and left sinus horns (see Fig. 13.5 A ). The signaling molecule(s) and cellular mechanisms responsible for cardiac looping are complex and involve pathways including the BMP, Notch, Wnt, and SHH pathways; all are required in heart tube remodeling.

As the primordial heart elongates and bends, it gradually invaginates into the pericardial cavity (see Figs. 13.7 B to D and 13.8 C and D ). The primordial heart is initially suspended from the dorsal wall by a mesentery (double layer of peritoneum ), the dorsal mesocardium. The central part of the mesentery soon degenerates, forming a communication, the transverse pericardial sinus , between the right and left sides of the pericardial cavity (see Fig. 13.8 E and F ). The primordial heart is now attached only at its cranial and caudal ends.
Circulation Through Primordial Heart
The initial contractions of the heart are of myogenic origin (in or starting from muscle). The muscle layers of the atrium and ventricle outflow tract are continuous, and contractions occur in peristalsis-like waves that begin in the sinus venosus. At first, circulation through the primordial heart is an ebb-and-flow type; however, by the end of the fourth week, coordinated contractions of the heart result in unidirectional flow. Blood enters the sinus venosus (see Fig. 13.10 A and B ) from the:
- •
Embryo through the common cardinal veins
- •
Developing placenta through the umbilical veins
- •
Umbilical vesicle through the vitelline veins
Blood from the sinus venosus enters the primordial atrium ; flow from it is controlled by sinuatrial (SA) valves ( Fig. 13.11 A to D ). The blood then passes through the atrioventricular (AV) canal into the primordial ventricle. When the ventricle contracts, blood is pumped through the bulbus cordis and truncus arteriosus into the aortic sac, from which it is distributed to the pharyngeal arch arteries in the pharyngeal arches (see Fig. 13.10 C ). The blood then passes into the dorsal aortae for distribution to the embryo, umbilical vesicle, and placenta (see Fig. 13.2 ).

Partitioning of Primordial Heart
13
Partitioning of the AV canal, primordial atrium, ventricle, and outflow tract begins during the middle of the fourth week. Partitioning is essentially completed by the end of the eighth week. Although described separately, these processes occur concurrently.
Partitioning of Atrioventricular Canal
Toward the end of the fourth week, AV endocardial cushions form on the dorsal and ventral walls of the AV canal (see Fig. 13.11 A and B ). The AV endocardial cushions develop from a specialized extracellular matrix (cardiac jelly) , as well as neural crest cells (see Fig. 13.8 C and D ). As these masses of tissue are invaded by mesenchymal cells during the fifth week, the AV endocardial cushions approach each other and fuse, dividing the AV canal into right and left canals (see Fig. 13.11 C and D ). These canals partially separate the primordial atrium from the primordial ventricle, and the endocardial cushions function as AV valves . The septal valves are derived from the fused superior and inferior endocardial cushions. The mural leaflets (thin, flattened layers of wall) are mesenchymal in origin.
After inductive signals emanate from the myocardium of the AV canal, a segment of the inner endocardial cells undergoes epithelial–mesenchymal transformation , and the resulting cells then invade the extracellular matrix. The transformed AV cushions contribute to the formation of the valves and membranous septa of the heart.
Transforming growth factor-β (TGF-β 1 and TGF-β 2 ), bone morphogenetic proteins (BMP-2A and BMP-4), the zinc finger protein Slug, and an activin receptor–like kinase (ChALK2) have been reported to be involved in the epithelial–mesenchymal transformation and formation of the endocardial cushions.
Partitioning of Primordial Atrium
Beginning at the end of the fourth week, the primordial atrium is divided into right and left atria by the formation of, and subsequent modification and fusion of, two septa: the septum primum and septum secundum ( Figs. 13.12 and 13.13 ).



The septum primum , a thin crescent-shaped membrane, grows toward the fusing endocardial cushions from the roof of the primordial atrium , partially dividing the common atrium into right and left halves. As the curtain-like muscular septum primum grows, a large opening, or foramen primum , remains between its crescentic free edge and the endocardial cushions (see Figs. 13.12 C and 13.13 A to C ). This foramen serves as a shunt, enabling oxygenated blood to pass from the right to the left atrium. The foramen becomes progressively smaller and disappears as the mesenchymal cap of the septum primum fuses with the fused AV endocardial cushions to form a primordial AV septum (see Fig. 13.13 D and D 1 ). Molecular studies have revealed that a distinct population of extracardiac progenitor cells from the second heart field migrates through the dorsal mesocardium to complete the lateral septum; Shh signaling plays a critical role in this process.
Before the foramen primum disappears, perforations produced by apoptosis appear in the central part of the septum primum. These perforations coalesce to form another opening in the septum primum, the foramen secundum . As the free edge of the septum primum fuses with the left side of the fused endocardial cushions, obliterating the foramen primum (see Figs. 13.12 D and 13.13 D ). The foramen secundum ensures continued shunting of oxygenated blood from the right to the left atrium.
The septum secundum , a thick crescentic muscular fold, grows from the muscular ventrocranial wall of the right atrium, immediately adjacent to the septum primum (see Fig. 13.13 D 1 ). As this thick septum grows during the fifth and sixth weeks, it gradually overlaps the foramen secundum in the septum primum (see Fig. 13.13 E ). The septum secundum forms an incomplete partition between the atria; consequently, a foramen ovale forms. The cranial part of the septum primum, initially attached to the roof of the left atrium, gradually disappears (see Fig. 13.13 G 1 and H 1 ). The remaining part of the septum, attached to the fused endocardial cushions, forms the flap-like valve of the foramen ovale.
Before birth , the foramen ovale allows most of the oxygenated blood entering the right atrium from the IVC to pass into the left atrium ( Fig. 13.14 A , and see also Fig. 13.13 H ). It also prevents the passage of blood in the opposite direction because the septum primum closes against the relatively rigid septum secundum (see Fig. 13.14 B ).

After birth , the foramen ovale functionally closes because the pressure in the left atrium is higher than that in the right atrium. At approximately 3 months, the valve of the foramen ovale fuses with the septum secundum, forming the oval fossa (fossa ovalis; see Fig. 13.14 B ). As a result, the interatrial septum becomes a complete partition between the atria.
Changes in Sinus Venosus
Initially, the sinus venosus opens into the center of the dorsal wall of the primordial atrium , and its right and left horns are approximately the same size (see Fig. 13.5 A ). Progressive enlargement of the right horn results from two left-to-right shunts of blood :
- •
The first shunt results from transformation of the vitelline and umbilical veins.
- •
The second shunt occurs when the anterior cardinal veins are connected by an anastomosis (see Fig. 13.5 B and C ). This communication shunts blood from the left to the right anterior cardinal vein; this shunt becomes the left brachiocephalic vein . The right anterior cardinal vein and right common cardinal vein become the SVC ( Fig. 13.15 C ).
By the end of the fourth week, the right horn of the sinus venosus is noticeably larger than the left horn ( Fig. 13.15 A ). As this occurs, the SA orifice moves to the right and opens in the part of the primordial atrium that will become the adult right atrium (see Figs. 13.11 and 13.15 C ). As the right horn of the sinus enlarges, it receives all the blood from the head and neck through the SVC and from the placenta and caudal regions of the body through the IVC. Initially, the sinus venosus is a separate chamber of the heart and opens into the dorsal wall of the right atrium (see Fig. 13.10 A and B ). The left horn becomes the coronary sinus , and the right horn is incorporated into the wall of the right atrium (see Fig. 13.15 B and C ).

Because it is derived from the sinus venosus, the smooth part of the wall of the right atrium is called the sinus venarum of the right atrium (see Fig. 13.15 B and C ). The remainder of the anterior internal surface of the atrial wall and the conical muscular pouch, the right auricle , has a rough trabeculated appearance. These two parts are derived from the primordial atrium. The smooth part and the rough part are demarcated internally in the right atrium by a vertical ridge, the crista terminalis , and externally by a shallow groove, the sulcus terminalis (see Fig. 13.15 B ). The crista terminalis represents the cranial part of the right SA valve (see Fig. 13.15 C ). The caudal part of the SA valve forms the valves of the IVC and coronary sinus. The left SA valve fuses with the septum secundum and is incorporated with it into the interatrial septum.
Primordial Pulmonary Vein and Formation of Left Atrium
Most of the wall of the left atrium is smooth because it is formed by incorporation of the primordial pulmonary vein ( Fig. 13.16 A ). This vein develops as an outgrowth of the dorsal atrial wall, just to the left of the septum primum. As the atrium expands, the primordial pulmonary vein and its main branches are incorporated into the wall of the left atrium. As a result, four pulmonary veins are formed ( Fig. 13.16 C and D ).

Molecular studies have confirmed that atrial myoblasts migrate into the walls of the pulmonary veins. The functional significance of this pulmonary cardiac muscle (pulmonary myocardium) is uncertain. The small left auricle is derived from the primordial atrium; its internal surface has a rough trabeculated appearance.
In the disorder involving total anomalous pulmonary venous connections, none of the pulmonary veins connect with the left atrium. Most commonly, the veins coalesce into a confluence of one of the systemic veins posterior to the left atrium and then drain into this chamber of the heart. Less commonly the veins may descend below the diaphragm and empty into the hepatic venous system, where they commonly become obstructed and produce increased pressure, leading to significant congestive heart failure. In the disorder involving partial anomalous pulmonary venous connections, one or more pulmonary veins have similar anomalous connections, but the others have normal connections.
Partitioning of Primordial Ventricle
Division of the ventricle is indicated by a median ridge, the muscular interventricular septum , in the floor of the ventricle near its apex (see Fig. 13.12 B ). Myocytes (muscle cells) from both the left and right primordial ventricles contribute to the formation of the muscular part of the interventricular septum . The septum has a concave free edge ( Fig. 13.17 A ). Initially, it attains most of its height from dilation of the ventricles on each side of the muscular interventricular septum ( Fig. 13.17 B ). Later, there is active proliferation of myoblasts in the septum, which increases the size of the septum.

Until the seventh week, there is a crescent-shaped interventricular foramen between the free edge of the interventricular septum and the fused endocardial cushions. The foramen permits communication between the right and left ventricles ( Fig. 13.18 B , and see also Fig. 13.17 ). The foramen usually closes by the end of the seventh week as the bulbar ridges fuse with the endocardial cushion ( Fig. 13.18 C to E ).

Closure of the interventricular foramen and formation of the membranous part of the interventricular septum result from the fusion of tissues from three sources: the right bulbar ridge, the left bulbar ridge, and the endocardial cushion. The membranous part of the interventricular septum is derived from an extension of tissue from the right side of the endocardial cushion to the muscular part of the septum as well as neural crest cells. This tissue merges with the aorticopulmonary septum and the thick muscular part of the interventricular septum ( Fig. 13.19 C , and see also Fig. 13.18 E ). After closure of the interventricular foramen and formation of the membranous part of the interventricular septum, the pulmonary trunk is in communication with the right ventricle, and the aorta communicates with the left ventricle (see Fig. 13.18 E ).

Cavitation of the ventricular walls forms a spongy mass of muscular bundles, trabeculae carneae . Some of these bundles become papillary muscles and tendinous cords (chordae tendineae) . The cords run from the papillary muscles to the AV valves (see Fig. 13.19 C and D ) .
Cardiac screening using high-resolution real-time ultrasonography is usually first performed between 18 and 22 weeks of gestation ( Fig. 13.20 ), when the heart is large enough to examine. Based on international convention, a four-chamber view of the heart is obtained (see Fig. 13.20 ) and the great vessels are also examined for anomalies.

Partitioning of Bulbus Cordis and Truncus Arteriosus
During the fifth week, active proliferation of mesenchymal cells in the walls of the bulbus cordis results in the formation of bulbar ridges ( Fig. 13.21 B and C , and see also Fig. 13.18 C and D ). Similar ridges that are continuous with the bulbar ridges form in the truncus arteriosus . The bulbar and truncal ridges are derived largely from neural crest mesenchyme (see Fig. 13.21 B and C ).

Neural crest cells migrate through the primordial pharynx and pharyngeal arches to reach the ridges. As this occurs, the bulbar and truncal ridges undergo a 180-degree spiraling. The spiral orientation of the ridges, caused in part by streaming of blood from the ventricles, results in the formation of a spiral aorticopulmonary septum when the ridges fuse (see Fig. 13.21 D to G ). This septum divides the bulbus cordis and truncus arteriosus into two arterial channels, the ascending aorta and pulmonary trunk. Because of the spiraling of the aorticopulmonary septum, the pulmonary trunk twists around the ascending aorta (see Fig. 13.21 H ).
The bulbus cordis is incorporated into the walls of the definitive ventricles (see Fig. 13.18 A and B ):
- •
In the right ventricle, the bulbus cordis is represented by the conus arteriosus (infundibulum), which is the origin of the pulmonary trunk.
- •
In the left ventricle, the bulbus cordis forms the walls of the aortic vestibule , the part of the ventricular cavity just inferior to the aortic valve.
Development of Cardiac Valves
When partitioning of the truncus arteriosus is nearly completed (see Fig. 13.21 A to C ), the semilunar valves begin to develop from three swellings of subendocardial tissue around the orifices of the aorta and pulmonary trunk. Cardiac precursor neural crest cells also contribute to this tissue. These swellings are hollowed out and reshaped to form three thin-walled cusps ( Fig. 13.22 , and see also Fig. 13.19 C and D ). The AV valves (tricuspid and mitral valves) develop similarly from localized proliferations of tissue around the AV canals.

Full access? Get Clinical Tree
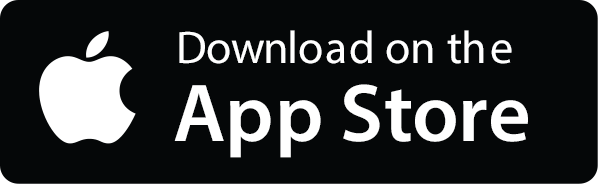
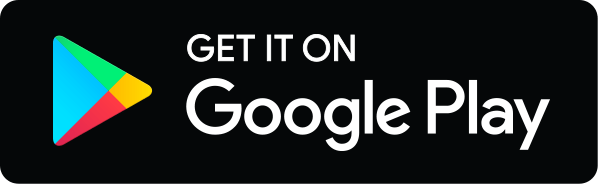