Cardiovascular Embryology
Louis I. Bezold
The development of the cardiovascular system—first organ system to reach functional maturity—is essentially complete by 8 weeks’ gestational age in humans. The critical period of heart development occurs between 20 and 50 days’ gestation (Fig. 51.1). For some time, anatomic and morphologic studies have formed the major basis of cardiovascular embryology, with recent expansion of our understanding of developmental mechanisms being gained through advances in molecular biology and experimental embryology.
ORIGINS OF CARDIAC CELL LINES
The mature heart is a composite of several embryonic cell types (Fig. 51.2). Most of the heart, including the endocardium, atrial
and ventricular myocardium, and Purkinje cells (specialized conduction systema), are derived from the cardiac mesoderm of the embryo. Cardiac neural crest cell migration contributes to the smooth muscle of the aortic wall, autonomic innervation of the heart, and outlet septation. The proepicardium contributes the epicardium and participates significantly in the development of the coronary arteries.
and ventricular myocardium, and Purkinje cells (specialized conduction systema), are derived from the cardiac mesoderm of the embryo. Cardiac neural crest cell migration contributes to the smooth muscle of the aortic wall, autonomic innervation of the heart, and outlet septation. The proepicardium contributes the epicardium and participates significantly in the development of the coronary arteries.
BASIC EMBRYOGENESIS
After fertilization, the ovum travels down the fallopian tube while undergoing several cellular divisions that result in a multicelled structure called the morula. By the time the morula reaches the uterine cavity on day 4 after fertilization, a cavity develops within it, at which point it is known as a blastocyst. Implantation into the uterine stroma occurs on approximately day 7. By this time, the blastocyst has further differentiated into an inner cell mass, or embryoblast, which ultimately forms the embryo proper, and an outer cell mass, which forms the embryonic membranes and contributes to formation of the placenta and umbilical cord.
The bilaminar germ disk forms during the second week of gestation, with differentiation of the embryoblast into two cell layers: the endoderm (hypoblast) and the ectoderm (epiblast). Late in week 2 of development, a third cell layer, the intraembryonic mesoderm, becomes apparent. The mesoderm is derived from ectodermal cells, which migrate toward the primitive streak in the midline of the embryo and invaginate between the ectoderm and the endoderm, a process called gastrulation (Fig. 51.3, top panel). The timing and entry point of these cells through the primitive streak confer some restriction on cell fate, with cells entering early and caudally ultimately ending up in the venous end of the heart and cells entering later and more cranially ending up in the outflow portion of the heart. The mesodermal cells migrate laterally and cephalically under the influence of the underlying endoderm, meeting anterior to the prochordal plate (buccopharyngeal membrane) to form the cardiogenic plate by day 15 of gestation. Mesodermal cells from this region are determined genetically to become myocardial cells by this point.
FORMATION OF THE STRAIGHT HEART TUBE
The intraembryonic mesoderm further differentiates into three portions: the paraaxial, the intermediate, and the lateral plate mesoderm, the last of which contributes to cardiac development. The lateral plate splits to form a cavity known as the intraembryonic coelom, which ultimately forms the pleural, pericardial, and peritoneal cavities. The more medial layer of the lateral plate mesoderm, adjacent to the endoderm, is called the splanchnic or visceral mesoderm (Fig. 51.3, middle panel). This layer contains the precardiac mesoderm from which the endocardial and myocardial cells will arise. Multiple transcription factors, anterior endoderm induction, and the process of epithelial-mesenchymal transformation are involved in the differentiation of the precardiac mesoderm. The outer layer of the mesoderm, the somatic or parietal mesoderm, will form the body linings, including the pericardium and the pleura.
In conjunction with cardiogenic plate formation and mesodermal differentiation, the embryo undergoes two major conformational changes. The relatively rapid expansion of the cephalic end of the neural tube results in anterior folding of the cardiogenic area, and accelerated growth of the somites along the longitudinal axis of the body results in the lateral margins of the embryo folding ventrally (Fig. 51.4). The net result of these maneuvers is the formation of an endodermal tube (embryonic foregut and hindgut) and apposition of the lateral mesoderm in the ventral midline, anterior to the foregut. Initially, the precardiac mesoderm forms two solid strands, each of which eventually develops lumens (paired endocardial tubes), and they finally fuse in the midline to form a single straight heart tube (Fig. 51.3, bottom panel). Programmed cell death (apoptosis) appears to play a role in this fusion process. The heart tube at this stage has two layers, classically known as an inner endocardium and an outer epimyocardium; these terms originated from early concepts of the mature cardiac tissues to which these embryonic layers were thought to give rise. More recent observations have shown that the term epimyocardium
is inaccurate. This layer actually contains only myocardial cells; the epicardium originates later in development from pericardial villi (proepicardial organ) at the venous end of the heart. Between the two cell layers is a relatively hypocellular material known as cardiac jelly, which also is somewhat of a misnomer because the molecular makeup of this substance is quite complex. Cardiac jelly is thought to contribute significantly to the critical processes of cardiac looping and septation of the atrioventricular canal and conotruncal regions of the heart.
is inaccurate. This layer actually contains only myocardial cells; the epicardium originates later in development from pericardial villi (proepicardial organ) at the venous end of the heart. Between the two cell layers is a relatively hypocellular material known as cardiac jelly, which also is somewhat of a misnomer because the molecular makeup of this substance is quite complex. Cardiac jelly is thought to contribute significantly to the critical processes of cardiac looping and septation of the atrioventricular canal and conotruncal regions of the heart.
Concurrent with formation of the heart tube, blood islands in the visceral mesoderm form endothelium-lined vessels and blood-forming elements. The primitive heart tube receives the developing systemic veins caudally and connects to the first arterial arches cephalically.
Figure 51.5 shows the primitive straight heart tube. The variability in the interpretation of the segments and the terminology describing them reflects the disagreement that exists in classifying the parts of the primitive heart tube. Table 51.1 outlines the traditional nomenclature of the commonly described embryonic segments, the connecting regions of the primitive heart tube, and the mature heart derivatives to which the segments are thought to give rise. Cellular marking studies suggest that significant portions of both the cephalic and caudal ends of the mature heart are formed during and after cardiac looping, by cells not present in the original heart tube. The molecular processes and precursor cells involved are not well understood but include the migration of extracardiac mesenchymal and neural crest cells.
CARDIAC LOOPING
Cardiac looping, the process by which the straight heart tube normally bends to the right (dextral or D-loop) and slightly ventrally at approximately 23 days’ gestation, is a crucial step in cardiac morphogenesis. Looping is the earliest sign of developing left–right asymmetry in vertebrates. Abnormalities of looping are associated with complex congenital heart disease, including abnormalities of ventricular relationships (i.e., ventricular inversion) and heterotaxy syndromes. Complete failure of looping results in embryonic loss. After looping occurs, the external appearance of the heart is similar to the mature organ; however, the heart remains a single tube internally. Looping results in the common atrium being located somewhat posterior and cephalad to the ventricles, and the outlet segment comes to lie in the midline, in a slight depression of the roof of the primitive atria. Looping is critical to the subsequent internal partitioning of the heart tube.
TABLE 51.1. EMBRYONIC SEGMENTS AND CONNECTING REGIONS OF THE PRIMITIVE HEART TUBE | ||||||||||||||||||
---|---|---|---|---|---|---|---|---|---|---|---|---|---|---|---|---|---|---|
|
The mechanisms that determine left- and right-sidedness and the forces responsible for cardiac looping are not clearly determined, but they are under intensive investigation on the molecular and genetic levels. Currently, the most plausible theory is that cardiac jelly generates a uniform force (caused by changes in the status of hydration within the jelly) that is converted to a directional force by the specific myofibrillar arrangement within the surrounding myocardial cells. Molecular biologic investigations are ongoing using iv/iv and inv/inv mouse models of situs inversus, and molecular biology continues to advance research into the potential roles of signaling molecules and extracellular matrix molecules with asymmetric expression of mRNA at various times in early development.
Internal Partitioning of the Heart
The embryonic heart is a tube within a single closed vascular circuit that must be converted to a four-chambered structure with a second, separate circuit (lungs) for gas exchange. Cardiac septation takes place between days 26 and 37 of gestation. Four concurrent events take place: atrial septation, formation of endocardial cushions and atrioventricular canal septation, ventricular chamber formation and septation, and truncus septation. Multiple cellular mechanisms, including coalescence of hypocellular masses (atrioventricular canal and conotruncal septum), rapid expansion of adjacent chambers (primary interventricular septum), participation by extracardiac mesenchyme (sinus venosus, atria, and truncus arteriosus), and neural crest cell migration (aortopulmonary septation), are involved. Although different mechanisms are involved in septation at different levels, the resulting system of septae can be thought of as a continuous system of ridges extending from the sinus venosus to the truncus arteriosus (Fig. 51.6).
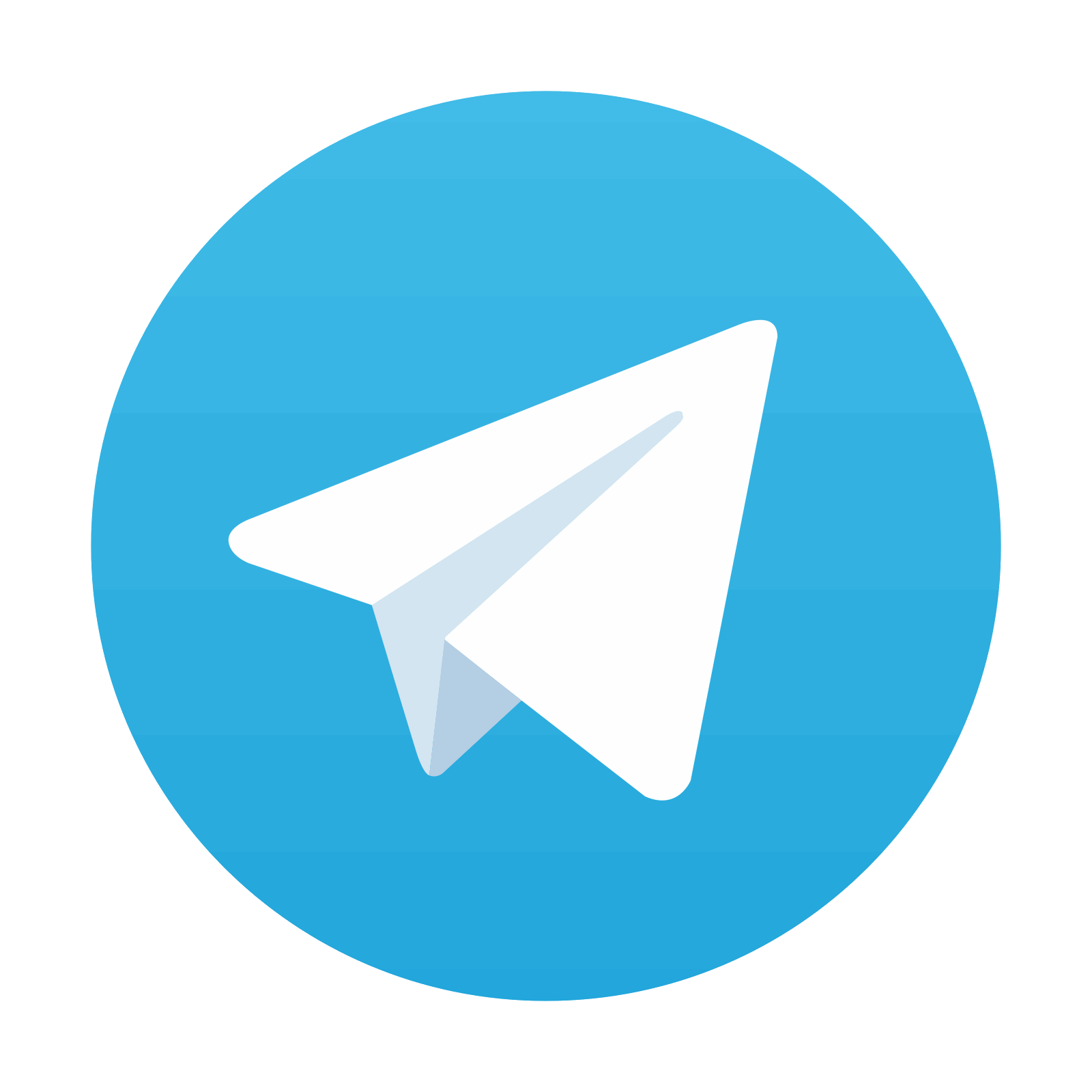
Stay updated, free articles. Join our Telegram channel

Full access? Get Clinical Tree
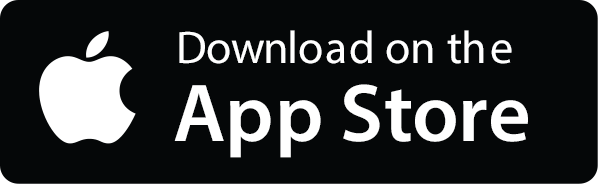
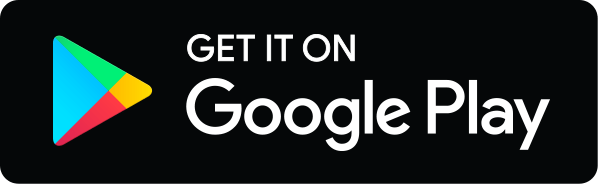