Objective
Women who have had preeclampsia demonstrate higher prevalence of metabolic syndrome (MetS), impaired vascular function, and increased sympathetic activity and are at increased risk of cardiovascular disease. The aim of this study was to assess the effects of 12 weeks of exercise training (70-80% maximum volume of oxygen utilization) in women who had had preeclampsia on physical fitness, components of MetS, vasculature, and autonomic functions compared with healthy control subjects.
Study Design
Our prospective case-control study included 24 normotensive women who had had preeclampsia and 20 control subjects who were matched for age and postpartum interval (all 6-12 months after delivery). Before and after training, we measured all components of MetS (ie, BP, lipids, glucose/insulin, and albuminuria), carotid intima media thickness (IMT) and brachial and superficial femoral artery endothelial function that used flow-mediated dilation (FMD). Autonomic activity was quantified with power spectral analysis (low-frequency/high-frequency power [LF/HF] ratio).
Results
At baseline, women who had had preeclampsia demonstrated higher values of most components of MetS. Compared with the control subjects, women who had had preeclampsia had increased IMT (580 ± 92 μm vs 477 ± 65 μm, respectively), impaired endothelial function (FMD brachial artery, 5.3% ± 2.2% vs 10.8% ± 3.5%, respectively; FMD superficial femoral artery, 4.9% ± 2.1% vs 8.7% ± 3.2%, respectively) and increased LF/HF power ratio (2.2 ± 1.0 vs 1.3 ± 0.4, respectively; all P < .05). In both groups, exercise training decreased values of most components of MetS and IMT, improved FMD, and concurrently reduced LF/HF. Despite these improvements, vascular and autonomic variables did not normalize by 12 weeks of training in women who had had preeclampsia.
Conclusion
This study demonstrates that exercise training in women who had had preeclampsia and control subjects improves components of MetS, endothelial function, vascular wall thickness, and autonomic control. Nonetheless, trained women who had had preeclampsia only reached a cardiovascular status that is comparable with sedentary healthy control subjects.
Women who have experienced preeclampsia during pregnancy are prone to cardiovascular disease later in life. The cardiovascular stress test of pregnancy therefore may unmask a previously unrecognized cardiovascular risk profile in young women. Most likely the development of preeclampsia during pregnancy reflects their preexisting, often latent, cardiovascular risk condition. Alternatively, the increased risk can be attributed to factors that originate from the complicated pregnancy itself. In either case, women who have had preeclampsia may benefit from selective screening for cardiovascular risk markers, assuming that targeted treatment alters these risk factors, and effectively alter the risk for pregnancy-related vascular complications and future cardiovascular disease.
Women who have had preeclampsia demonstrate higher prevalence of traditional cardiovascular risk factors (eg, hypertension, dyslipidemia, and reduced insulin sensitivity), endothelial dysfunction, increased arterial wall thickness, and augmented sympathetic nervous system activity, compared with healthy control subjects, which likely relates to the increased cardiovascular risks in these women. These dysfunctions potentially are amenable for improvement by exercise training.
Aerobic exercise training is a well-established potent physiologic stimulus that reduces primary and secondary cardiovascular events. The beneficial effects of exercise on cardiovascular risk are mediated not only through improvement of traditional cardiovascular risk factors but also through up-regulation of nitric oxide (NO)–derived endothelium-mediated vasodilation, reduction of arterial wall thickness, and reduction of sympathetic tone. Although suggested in many articles, no previous study has examined the effects of exercise training in women who have had preeclampsia that could provide strong rationale for advising exercise training in women with increased cardiovascular risk.
This study therefore addressed the question to what extent an exercise training program (12 weeks of cycling at 70-80% maximum volume of oxygen utilization [VO 2 max], 2-3 times per week) in women who had had preeclampsia affects physical fitness, components of the metabolic syndrome, endothelial function, arterial wall thickness, and autonomic function and how these changes compare with control subjects. The primary outcome of this study was endothelial function, which was measured by flow-mediated dilation (FMD). Endothelial dysfunction is not only a key feature in preeclampsia, but also it has proved to be associated with cardiovascular disease burden and outcome. We hypothesize that exercise training improves vascular function and structure as well as autonomic control, we expect that these vascular and autonomic changes to be related closely. The question is, however, whether women who have had preeclampsia will respond adequately to the exercise stimulus, because these women maladapted to the cardiovascular stimulus of previous pregnancy. Based on the circulatory maladaptation to previous pregnancy, we hypothesized that women who have had preeclampsia are less able to improve vascular and autonomic characteristics with exercise training compared with healthy control subjects. We therefore performed an experimental intervention study in 24 women who had had preeclampsia and 20 control subjects.
Materials and Methods
Subjects
We recruited 25 normotensive women who had had preeclampsia and 22 control subjects. Primiparous women who had had preeclampsia were recruited from the Radboud University Nijmegen Medical Centre, and control subjects were recruited from the community by advertisement at day care centers. Preeclampsia in previous pregnancy was defined by the combination of gestational hypertension (≥140/90 mm Hg, measured 2 and ≥6 hours apart) and proteinuria (consistently ≥300 mg/24 hours) at >20 weeks of gestation in previously normotensive women. In our hospital, women who have had preeclampsia are invited for cardiovascular follow-up evaluation 6-12 months after pregnancy that was complicated by preeclampsia. At this follow-up visit, women who were eligible for our study were given written information about this study, and if interested, these women contacted our laboratory for further information and/or inclusion. Control subjects contacted our laboratory after reading the advertisement. If interested, they received the same written information as women who had had preeclampsia. Control subjects were healthy primiparous women whose pregnancy charts were checked to ensure a normal pregnancy course that resulted in a term delivery. Participants were not compensated in this study.
All participants were white women who were healthy and normotensive at the time of measurements. None of the women had diabetes mellitus, autoimmune disease, or overt cardiovascular disease. None of the women smoked or used medication or supplements that might affect the cardiovascular system. None of the women who were included were pregnant, breastfeeding, or using hormonal contraceptives. Excluded from analysis were women who became pregnant during the course of the study and women who were unable to cope with physical exercise training. The study was approved by the Medical Ethics Committee of the Radboud University Nijmegen Medical Centre. All participants gave written informed consent before entering the study. The study adhered to the principles of the Declaration of Helsinki and was registered at clinicaltrials.gov (id: NCT00900458 ).
Experimental design
Measurements and training were performed in the nonpregnant state, 6-12 months after pregnancy. Subjects were tested before and after 12 weeks of exercise training. All measurements, except VO 2 max, were performed during the same visit. VO 2 max was tested 1-5 days from the other visit. In sequential order, we measured body characteristics (weight, height, waist circumference), metabolic components (lipids, glucose, and insulin concentrations), blood pressure (BP), heart rate (HR), autonomic function by spectral analysis of spontaneous fluctuations in HR and BP and vascular ultrasound measurements, intima media thickness (IMT), flow-mediated dilation (FMD) and vasodilation response to glyceryl trinitrate (GTN).
Experimental procedures
Tests and measurements were performed between days 3 and 11 of the menstrual cycle to minimize possible endocrine influences of the sex hormones on the cardiovascular and autonomic nervous system. All measurements, except VO 2 max were performed after an overnight fast. Participants were instructed to abstain from strenuous physical activity in the 24 hours before testing. Participants collected urine in the 24 hours preceding the measurements. The 24-hour urine sample was assayed for albumin, protein, and creatinine to define microalbuminuria corrected for creatinine output (g/mol creatinine) and total protein level (g/24 hours; Aeroset; Abbott Laboratories, Green Oaks, IL).
Height, body mass (888 scale; Seca, Hamburg, Germany), and waist circumference were measured. Venous blood samples were taken from the antecubital vein and analyzed for metabolic parameters: glucose, insulin, total cholesterol, high- and low-density lipoproteins, and triglycerides (Aeroset; Abbott Laboratories). The homeostasis model assessment index was calculated as fasting insulin to estimate insulin resistance: fasting insulin (mU/L) × fasting glucose (mmol/L)/22.5.
Tests were performed under standardized conditions in a temperature-controlled room (22 ± 0.5°C). Measurements were performed at the same time in the morning to prevent diurnal variation in the vascular and autonomic responses. After 30 minutes rest in the supine position, BP and HR were measured oscillometrically (Dinamap, Vital Signs Monitor 1846; Critikon Inc, Tampa, FL), at the right upper arm, with the cuff size recommended for the arm circumference, at 3-minute intervals for 30 minutes. We recorded systolic (SAP), diastolic (DAP), and mean (MAP) arterial pressures and HR (beats/min) and used the median values of 9 consecutive measurements for analysis. This resting HR was used for the calculation of the training intensity.
Autonomic function
Autonomic function was measured with subjects comfortably lying in supine position in a quiet, partially darkened room. Autonomic activity and baroreflex sensitivity (BRS) were quantified by spectral analysis, from a 5-minute recording of spontaneous fluctuations in HR and BP. HR and arterial BP were measured continuously using a finger arterial BP–monitoring device attached to the third digit of the right hand at a sampling rate of 100Hz (Finometer; Finapres Medical Systems BV, Amsterdam, The Netherlands). Post hoc, these recordings were subdivided into data segments of 100s that overlapped for 50% and resampled at 5.12 Hz. Each segment was then analyzed with fast Fourier transformation to search for rhythmic fluctuations in SAP and pulse interval with a frequency range between 0 and 2.56 Hz. The amplitude of each fluctuation determines the power at each frequency. The ratio of absolute low-frequency (LF) and high-frequency (HF) powers of the pulse interval was used to represent the autonomic balance between the sympathetic and vagal system. The LF component is regarded a marker of mainly sympathetic modulation; HF components mainly reflect vagal activity. Therefore, a higher LF/HF ratio can be interpreted as a sign of increased sympathetic dominance. Baroreflex sensitivity (milliseconds/millimeters of Mercury) was defined as the LF transfer gain from SAP to pulse interval, which provides information about the changes in the HR (output) in response to fluctuation in SAP (input).
Vascular measurements
All measurements were performed by the same sonographer (R.R.S.) according to recent guidelines for assessment of the FMD who used a 10-MHz multifrequency linear array probe attached to a high resolution ultrasound machine (T3000; Terason Corporation, Burlington, MA). Ultrasound parameters were set to optimize longitudinal B-mode images of the lumen/arterial wall interface. Continuous Doppler velocity was assessed simultaneously with the use of the lowest possible insonation angle (always <60 degrees). For assessment of the FMD responses, a rapid inflation/deflation pneumatic cuff (Hokanson, Bellevue, WA) was positioned distal to the imaged artery and inflated for 5 minutes (220 mm Hg) to provide the stimulus for reactive hyperemia. FMD represents a predominantly NO-mediated, endothelium-dependent vasodilation.
Carotid artery intima media thickness
The intima media thickness (IMT) of the left common carotid artery (CA) was measured 2 cm proximal to the bulbus. Perpendicular incidence of the B-mode imaging ultrasound beam in relation to the orientation of the vessel provided clearly demarcated intima medial boundaries, which were optimized with the use of contrast control subjects. Wall thickness and diameter were measured continuously for 1 minute. The measurement was repeated once from a different perpendicular plane (±90 degree, compared with previous measurement). From the 2 measurements, mean diameter and wall thickness were calculated and used for analysis.
Brachial artery FMD
Brachial artery (BA) FMD was measured with subjects positioned supine with the right arm extended and immobilized with foam, supported at an angle of approximately 80 degrees from the torso. The pneumatic cuff was placed distal to the olecranon. The BA was scanned 2-5 cm above the antecubital fossa. Before inflation, resting baseline diameter and blood flow velocity were recorded continuously for at least 1 minute. After cuff deflation, diameter and blood flow velocity were recorded continuously for 3 minutes.
Superficial femoral artery FMD
Superficial femoral artery (SFA) FMD was measured with subjects positioned supine with the lower leg slightly elevated (approximately 10 degrees). The pneumatic cuff was placed 15 cm below the inguinal ligament. The SFA was scanned in the proximal one-third of the thigh, at least 5 cm distal from the bifurcation and above the cuff position. Before inflation, resting baseline diameter and blood flow velocity were recorded continuously for at least 1 minute. After cuff deflation, diameter and blood flow velocity were recorded continuously for 5 minutes.
Vasodilation after GTN
GTN responses were measured after a resting period of 30 minutes to allow arterial diameter to return to baseline, another 1-minute baseline recording of the SFA was made. Endothelium-independent dilation was examined with the use of a single sublingual dose of GTN (400 μg; G-Pohl-Boskamp GmbH and Co KG, Hohenlockstedt, Germany), a NO donor, followed by ≥10 minutes of recording of the arterial diameter. The maximal dilation after administration of GTN represents the endothelium-independent vasodilation.
Conduit artery diameter, wall thickness, and blood flow analysis
Posttest analysis of diameter, velocity, and wall thickness of the 3 conduit arteries (CA, BA, and SFA) was performed with edge-detection and wall-tracking Digital Imaging and Communications in Medicine–based software (National Electrical Manufacturers Association, Rosslyn, VA) to minimize investigator bias. The post-hoc analysis of the diameter, velocity, and wall thickness was performed blinded (to subject and moment of assessment). FMD was calculated as the percentage of rise in peak diameter from the preceding baseline diameter. The postdeflation shear rate data, which was derived from simultaneously acquired velocity and diameter measures at 30 Hz, was used to calculate the area under the shear rate curve for data up to the point of maximal postdeflation diameter (FMD) for each measurement. Reproducibility of the BA FMD using this semiautomated software processes a coefficient of variance of 6.7% ; the SFA FMD has a coefficient of variance of 15%.
Physical fitness
Physical fitness was measured before and after a 12-week training program. Fitness was defined as the peak oxygen uptake (VO 2 max: milliliter minute –1 kilogram –1 ) during a maximal cycling test on a cycle ergometer (Excalibur Sport; Lode BV, Groningen, The Netherlands). Tests were performed in the afternoon after a light lunch. The initial workload set at 10 W for 1 minute followed by 10-W increments every minute until complete exhaustion. Breath-by-breath oxygen uptake was measured with spiroergometric equipment (Quark CPET, Cosmed, Milan, Italy). HR and rhythm were continuously recorded by 3-lead echocardiography. Maximal workload (workmax) was defined as the last completed work load before exhaustion. Test performance was considered to be adequate when (1) the increase in VO 2 (milliliters) during workmax was <150 mL compared with the previous workload, indicating plateau formation in oxygen uptake; (2) HR at workmax was <10 beats/min from estimated maximal HR (220-age); (3) the respiratory exchange ratio (CO 2 /O 2 ) was consistently >1.1 during workmax, and (4) capillary lactate level was >8 mmol/L 90 seconds after exhaustion. If the test failed to achieve these 4 qualifications, the test was repeated 2-3 days later. In 3 cases (2 women who had had preeclampsia, 1 control subject), the test had to be repeated; all tests were eventually considered adequate.
Exercise training
Exercise training consisted of 12 weeks of HR-controlled cycle training (cycle ergometer; Corival; Lode BV) at 70-80% of VO 2 max for 2-3 times per week (according to the most recent American College of Sports Medicine guideline). Participants trained twice a week during the first 6 weeks and 3 times a week during the last 6 weeks. Each training session was supervised and was executed in the gymnasium of our laboratory. Participants were instructed not to exercise in addition to the exercise protocol that they were given. During each training session, HR was monitored continuously and recorded (RS800CX; Polar Electro Inc, New York, NY). Each training session started with a 10-minute warm-up at 50% of the HR reserve (HRR) above the resting HR. HRR was calculated in the following manner: HRR = HR max – HR rest, in which HR max is the maximal HR measured during the fitness test at study entry and HR rest is the HR determined at rest. Training consisted of 40 minutes of cycling at 70-80% of the individual HR reserve above the resting HR. Within their target HR zone, participants were free to choose the number of revolutions per minute. The training was completed by cooling down for 5 minutes at warm-up workload.
Statistics
Statistical analyses were performed with SPSS software (version 17.0; SPSS Inc, Chicago, IL). All data are reported as mean ± SD, unless stated otherwise. Normality of data was tested with Kolmogorov-Smirnov tests. Statistical significance was assumed at a probability value of < .05. Two-way repeated-measures analysis of variance was used to examine differences between women who had had preeclampsia and control subjects (groups) to assess the effects of training (intervention) and to determine whether the training effects differed between patients and control subjects (groups × intervention interaction). Post-hoc Bonferroni analysis was used to correct for multiple comparisons. Pearson correlation coefficient was used to examine the relation between exercise-induced change in autonomic function (LF/HF ratio) and endothelial function (FMD) in both groups. Based on an anticipated difference in %FMD (which has a coefficient of variation of 6.7-10.5% ) between women who had had preeclampsia and control subjects of 4%, a power of the study of 90%, and an alpha level of .05, we needed 17 subjects per individual group. We based our group-size calculation for the intervention part of our study on an anticipated change in %FMD (primary outcome measure) of 2%, a power of 90%, and an alpha level of .05. Based on these assumptions, we required 18 subjects per group. To anticipate possible drop outs, we decided to include at least 20 subjects per group.
Results
Included in the analysis were 24 women who had had preeclampsia and 20 control subjects, after exclusion of 1 woman who had had preeclampsia (who became pregnant) and 2 control subjects (1 woman who became pregnant and 1 woman who did not finish the training). Mean age (32 ± 4 vs 32 ± 4 years, respectively; P = .62) and postpartum interval (7 ± 2 vs 7 ± 1 months, respectively; P = .88) were comparable between groups. Women who had had preeclampsia delivered at an earlier gestational age compared with control subjects (32 [range, 29–37] vs 40 [range, 38–41] weeks of gestation, respectively; P < .01) and delivered children with a lower birthweight compared with control subjects (1571 ± 675 vs 3532 ± 311 g, respectively).
Baseline values
Before training, physical fitness was similar in both groups (VO 2 max: control subjects, 28.2 ± 3.7 mL min –1 kg –1 ; patients, 27.0 ± 4.0 mL min –1 kg –1 , respectively; P = .32), as were body mass index, resting HR, and fasting glucose and cholesterol concentrations ( Table 1 ). In women who had had preeclampsia, group average values of SAP, DAP, MAP, fasting insulin and triglyceride concentrations, and albuminuria were higher than in control subjects. Vascular diameters (CA, BA, and SFA) were not different between groups. In women who had had preeclampsia, CA-IMT was larger and BA- and SFA-FMD responses were smaller than in control subjects ( Table 2 ). Mean shear rate area under the curve, time-to-peak of FMD, and vasodilation in response to GTN were not different between groups. In women who had had preeclampsia, the average LF/HF ratio was higher and BRS was lower than in control subjects ( Table 3 ).
Variables | Healthy control subjects (mean ± SD) | Women who had had preeclampsia (mean ± SD) | 2-way analysis of variance ( P value) | ||||
---|---|---|---|---|---|---|---|
Before training | After training | Before training | After training | Group a | Intervention b | Group X intervention c | |
Body mass index, kg/m 2 | 26.8 ± 3.4 | 25.1 ± 6.8 | 25.9 ± 4.9 | 24.4 ± 7.0 | .63 | .04 | .88 |
Waist circumference, cm | 93 ± 10 | 91 ± 10 | 92 ± 12 | 89 ± 11 | .84 | < .01 | .25 |
Arterial pressure | |||||||
Systolic, mm Hg | 109 ± 6 | 104 ± 6 | 119 ± 10 d | 112 ± 8 | < .01 | < .01 | .39 |
Diastolic, mm Hg | 66 ± 6 | 63 ± 6 | 74 ± 7 d | 69 ± 7 | < .01 | < .01 | .19 |
Mean, mm Hg | 78 ± 5 | 74 ± 5 | 86 ± 8 d | 80 ± 7 | < .01 | < .01 | .26 |
Resting heart rate, beats/min | 63 ± 9.8 | 57 ± 7.6 | 77 ± 8.7 d | 59 ± 8.9 | .15 | < .01 | .03 |
Biochemical parameters | |||||||
Fasting glucose, mmol/L | 4.7 ± 0.5 | 4.6 ± 0.5 | 4.8 ± 0.5 | 4.7 ± 0.5 | .51 | .01 | .32 |
Fasting insulin, mmol/L | 7.7 ± 6.9 | 5.9 ± 4.3 | 13.3 ± 9.3 d | 8.4 ± 5.0 | .04 | < .01 | .04 |
Homeostatic model assessment of insulin resistance index | 1.7 ± 1.8 | 1.3 ± 1.1 | 3.0 ± 2.3 d | 1.8 ± 1.1 | .04 | < .01 | .04 |
Total cholesterol, mmol/L | 4.5 ± 0.9 | 4.1 ± 0.8 | 4.6 ± 1.0 | 4.5 ± 1.1 | .45 | < .01 | .14 |
High density lipoprotein cholesterol, mmol/L | 1.2 ± 0.3 | 1.3 ± 0.3 | 1.1 ± 0.2 | 1.2 ± 0.2 | .19 | < .01 | .88 |
Low density lipoprotein cholesterol, mmol/L | 2.8 ± 0.8 | 2.5 ± 0.7 | 2.8 ± 0.8 | 2.7 ± 0.8 | .58 | < .01 | .58 |
Triglycerides, mmol/L | 0.8 ± 0.4 | 0.8 ± 0.3 | 1.4 ± 0.9 d | 1.1 ± 0.5 d | .02 | .18 | .19 |
Albuminuria, mg/mmoL creatinine | 0.4 ± 0.3 | 0.4 ± 0.3 | 2.4 ± 4.4 d | 1.7 ± 3.0 d | .04 | .07 | .08 |
Physical fitness | |||||||
VO 2 max, mL min –1 kg –1 | 28.2 ± 3.7 | 31.9 ± 3.5 | 27.0 ± 4.0 | 30.4 ± 5 | .32 | < .01 | .52 |
Maximal heart rate, beats/min | 186 ± 9 | 185 ± 7 | 189 ± 7 | 189 ± 6 | .08 | .57 | .32 |
Maximal ventilation, L/min | 96 ± 20 | 108 ± 10 | 88 ± 19 | 99 ± 18 | .07 | < .01 | .74 |
Maximal work load, W | 195 ± 27 | 210 ± 22 | 188 ± 25 | 204 ± 27 | .27 | < .01 | .53 |
a Patients vs control subjects
b Main effect exercise program
c Interaction between groups and exercise training
d P < .05 compared with control subjects before training ( t -test).
Variables | Healthy control subjects (mean ± SD) | Women who had had preeclampsia (mean ± SD) | 2-way analysis of variance ( P value) | ||||
---|---|---|---|---|---|---|---|
Before training | After training | Before training | After training | Group a | Intervention b | Group X intervention c | |
Carotid artery | |||||||
Resting diameter, mm | 5.6 ± 0.5 | 5.6 ± 0.5 | 5.7 ± 0.6 | 5.6 ± 0.4 | .98 | .99 | .74 |
Intima media thickness, μm | 477 ± 65 | 423 ± 65 | 580 ± 92 d | 530 ± 138 | < .01 | < .01 | .86 |
Wall-to-lumen ratio | 0.086 ± 0.012 | 0.076 ± 0.012 | 0.104 ± 0.02 d | 0.095 ± 0.025 | < .01 | < .01 | .86 |
Brachial artery | |||||||
Diameter at baseline, mm | 2.8 ± 0.3 | 3.0 ± 0.3 | 3.0 ± 0.3 | 3.1 ± 0.3 | .15 | <.01 | .10 |
Flow-mediated dilation, % | 11.8 ± 3.5 | 13.3 ± 3.6 | 5.3 ± 2.2 d | 8.1 ± 2.7 d | < .01 | < .01 | .58 |
Shear rate area under the curve, ×10 3 sec –1 | 27.9 ± 10.4 | 27.1 ± 12.7 | 26.3 ± 11.2 | 24.3 ± 13.1 | .44 | .41 | .93 |
Time to peak diameter, sec | 48 ± 15 | 51 ± 13 | 50 ± 14 | 59 ± 20 | .26 | .08 | .43 |
Superficial femoral artery | |||||||
Diameter at baseline, mm | 5.6 ± 0.7 | 5.8 ± 0.6 | 5.7 ± 0.7 | 5.7 ± 0.7 | .90 | .81 | .84 |
Flow-mediated dilation, % | 8.7 ± 3.2 | 10.5 ± 2.8 | 4.9 ± 2.1 d | 6.1 ± 2.2 d | < .01 | < .01 | .98 |
Shear rate area under the curve, ×10 3 sec –1 | 14.5 ± 9.1 | 14.7 ± 5.5 | 15.8 ± 10.5 | 14.4 ± 9.1 | .82 | .53 | .69 |
Time to peak diameter, sec | 83 ± 36 | 74 ± 27 | 85 ± 36 | 78 ± 38 | .62 | .08 | .88 |
Glyceryl trinitrate, % | 11.9 ± 4.0 | 13.0 ± 3 | 10.9 ± 3.3 | 12.1 ± 2.1 | .24 | .04 | .75 |
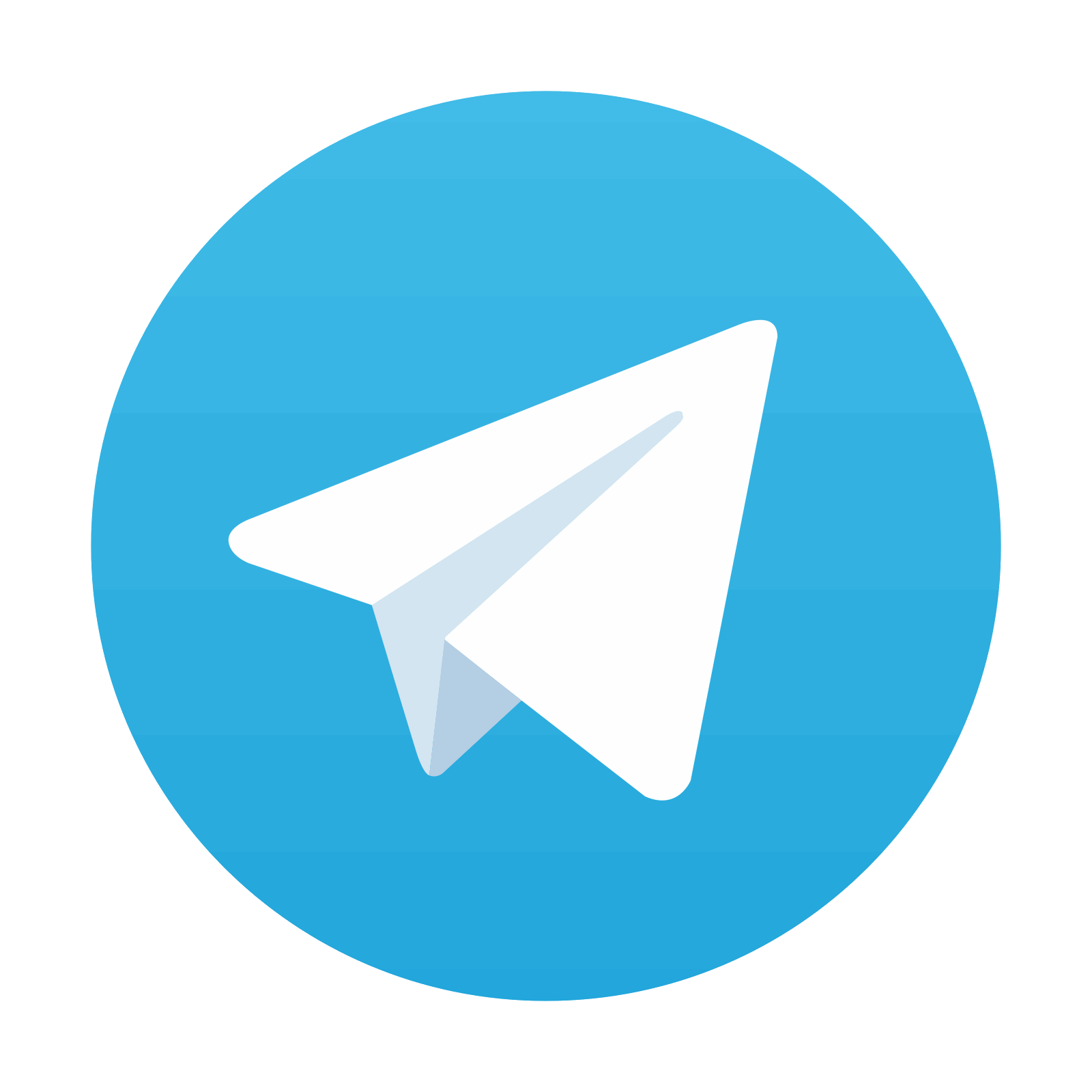
Stay updated, free articles. Join our Telegram channel

Full access? Get Clinical Tree
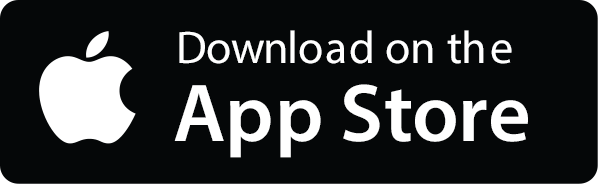
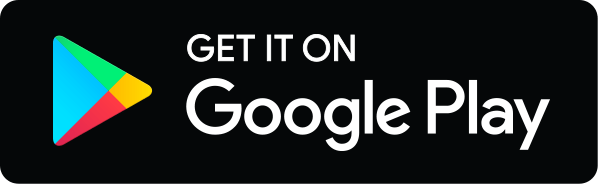