Chapter Contents
Introduction 618
Fetal circulation 618
Fetal cardiology 620
Incidence and aetiology of fetal and neonatal heart disease 621
Clinical implications of adaptation to birth 622
Normal adaptation 622
History and examination 625
Dysmorphic features and non-cardiac malformations 625
Pulses 626
Blood pressure 626
Cyanosis 626
Heart failure 626
Heart murmur 626
Airway obstruction 627
Investigations 627
Chest radiography 627
Electrocardiogram 627
Pulse oximetry 630
Hyperoxia test and blood gas analysis 630
Echocardiography 631
Cardiac catheterisation 633
Magnetic resonance and computed tomographic imaging 635
Treatment 635
General principles 635
Ductal manipulation 635
Heart failure 635
Antiarrhythmic drugs 639
Interventional catheterisation 640
Surgery 640
Persistent pulmonary hypertension of the newborn 640
Cardiovascular problems in the preterm infant 640
General considerations 640
Patent ductus arteriosus 641
Chronic lung disease 645
Endocarditis 645
Arrhythmias 645
Structural heart disease in the preterm infant 645
Structural heart disease in the newborn 645
Cyanosis 645
Pulmonary atresia 646
Pulmonary atresia with intact ventricular septum 647
Pulmonary atresia with ventricular septal defect 648
Pulmonary atresia as part of a complex lesion 648
Tetralogy of Fallot 648
Tetralogy of Fallot with absent pulmonary valve 648
Tricuspid atresia 648
Transposition 649
Total anomalous pulmonary venous connection 649
Complex structural cyanotic congenital heart disease 649
Common arterial trunk or truncus arteriosus 650
Ebstein’s anomaly of the tricuspid valve 650
Congenitally corrected transposition of the great arteries 650
Collapse 651
Arrhythmias 651
Duct-dependent pulmonary circulation and transposition 651
Duct-dependent systemic circulation 651
Hypoplastic left-heart syndrome 651
Critical aortic stenosis 653
Aortic arch interruption 653
Coarctation 654
Conclusion 654
Heart failure 654
Arrhythmias 655
Heart muscle disease 655
Myocardial ischaemia 655
Myocarditis 655
Dilated cardiomyopathy 655
Endocardial fibroelastosis 656
Hypertrophic cardiomyopathy 656
Isolated left ventricular non-compaction 656
Left-to-right shunt lesions 658
Arteriovenous malformation 658
Intracardiac left-to-right shunts 659
Conclusion 660
Cardiac conditions likely to present in the asymptomatic newborn 660
Innocent murmurs 660
Pathological asymptomatic heart murmurs 660
Practical approach to neonatal heart murmurs 660
Arrhythmias 661
Recognition of arrhythmias 661
Supraventricular arrhythmias 661
Sinus bradycardia 661
Sinus arrhythmia and sinus arrest 661
Atrial ectopics 661
Sinus tachycardia 662
Supraventricular tachycardia 662
Atrial flutter 662
Atrioventricular re-entry tachycardia 662
Ventricular arrhythmias 663
Premature ventricular complexes 663
Ventricular tachycardia 663
Idioventricular rhythm 663
Ventricular fibrillation 663
Atrioventricular conduction disturbances (heart block) 663
Hypertension 664
Cardiac tumours 665
Introduction
Neonatal cardiovascular disease can be primary or secondary, congenital or acquired, structural or functional. Whatever categories of abnormality are concerned, a similar systematic approach to history, examination and investigation is required to allow correct management. In many situations, an understanding of fetal as well as of neonatal cardiovascular physiology is necessary. In this chapter the approach is pathophysiological, with particular conditions being considered in the most appropriate category.
Fetal circulation
Much of the information about the fetal circulation has been derived from animal studies. Increasingly sophisticated, non-invasive, ultrasound assessment of the human fetal circulation has demonstrated important differences in the human fetus.
Fetal circulatory pathways
The fetal circulation consists of parallel systemic and pulmonary pathways in contrast to the normal postnatal circulation, in which the systemic and pulmonary circulations exist in series ( Fig. 28.1 ). For the most part, prenatal survival is possible with even major structural cardiovascular malformations, provided that either the right or left ventricle is able to pump blood derived from the great veins into the fetal aorta. It is this remarkably adaptive nature of the fetal circulation which enables fetuses with complex malformations such as those with atresia of either of the semilunar valves to survive throughout pregnancy. In the fetus, oxygenated blood returns from the placenta via the umbilical vein into the inferior caval vein, either through the portal system or through the venous duct. The proportion of blood channelled through these different routes changes as pregnancy progresses and will adapt continuously to the metabolic demands of the fetus by flow regulation in the venous duct. The stream of inferior caval blood entering the right atrium is divided as it hits the superior rim of the oval foramen and the majority of the blood enters the left atrium through the foramen. As pregnancy advances, the proportion crossing into the left atrium falls. Superior caval vein blood enters the right atrium and the majority will enter into the right ventricle via the tricuspid valve. Almost all of the right ventricular (RV) output will be directed through the arterial duct into the systemic circulation, bypassing the high-resistance pulmonary circulation. The proportion of pulmonary blood flow also changes with gestation, increasing during the third trimester. Just as in postnatal life, the fetal pulmonary vascular bed is reactive. Fetal pulmonary blood flow can be increased by manipulation of the pulmonary vasculature bed with pulmonary vasodilator agents (such as oxygen) administered to the mother (Rasanen et al. 1998). As pregnancy progresses, the effective cardiac output increases to a maximum of approximately 250 ml/kg/min by term, with the right ventricle contributing 55% and the left ventricle 45% of the fetal cardiac output. Of the combined output, 65% returns to the placenta and 35% to the fetal organs and tissues ( ).

Function of the fetal heart
Compared with the normal adult heart, there are a number of differences both within the fetal heart itself as well as between the physiological environment during fetal and postnatal life which explain many of the observations of fetal cardiac function. In early fetal life, the expression of contractile proteins is different from the postnatal pattern ( ). In addition, the expression of different types of collagen within fetal heart muscle results in reduced compliance of the fetal heart compared with the postnatal heart ( ). With advancing gestation, there is continuing maturation of excitation–contraction coupling as well as increasing autonomic innervation ( ). As a result, the immature fetal heart is much less compliant and less able to generate contractile force than its adult counterpart, for the same degree of stretch. However, the observation that the Starling curve in the fetus is blunted should not be attributed to the differences in the fetal myocardium alone. It is significantly influenced by the external constraints existing around the heart in the fetus, including the fluid-filled lungs and rigid chest wall.
Changes at birth
The most dramatic events that occur with birth are a shift from a circulation in parallel to one in series, as well as a marked increase in cardiac output from both ventricles. At term, the cardiac output from each ventricle approximately equals the combined cardiac output from both ventricles in the immediately preterm fetus. With inspiration, there is a rapid fall in pulmonary vascular resistance (PVR), as lung expansion allows new vessels to open and existing vessels to enlarge. Reduced resistance and decreased pulmonary artery pressures increase pulmonary blood flow. Simultaneously, the lower resistance placental circulation is removed from the systemic circulation as the cord is cut. The increased preload occurring from placental return can now be converted into useful increased cardiac output, once the external constraints of fluid-filled lungs around the heart are replaced by more compliant air-filled lungs. The sudden increase in oxygen tension produced by breathing alters local prostaglandin synthesis, resulting in a constriction of both the arterial and venous ducts. For most neonates, functional closure of the arterial duct will have occurred within 24–72 hours and anatomical closure is complete within 1–2 weeks. The oval foramen and venous duct may remain patent for some time after birth, with the potential to allow shunting. This can mask the signs of underlying structural congenital cardiovascular malformations, such as infracardiac total anomalous pulmonary venous drainage or, occasionally, transposition of the great arteries (TGA). The oval foramen is functionally closed in the majority of cases by the third month of life. Continued patency may contribute to clinical problems in some conditions ( Table 28.1 ).
Foramen ovale | Allows right-to-left shunting in persistent pulmonary hypertension of the newborn and other cardiac/respiratory conditions with increased right heart pressures |
Ductus venosus | No definite problem identified (except in obstructed total anomalous pulmonary venous connection: see text) |
Ductus arteriosus | Associated with major respiratory and other problems in preterm infants |
Important left-to-right shunting only rarely seen in the newborn period in term infants with patent ductus arteriosus |
Fetal cardiology
Diagnosis
Fetal cardiac anatomy, function and rhythm have been determined by transabdominal scanning from 18 weeks’ gestation for the last two decades ( ). An approach using both abdominal and (when necessary) transvaginal scanning in high-risk cases at 10–13 weeks allowed interpretable images in over 80% of cases ( ), with a probable low false-positive rate, although verification was incomplete. Screening fetal cardiac anatomy with a four-chamber view at 18 weeks is a routine part of the general anomaly scan, but this will pick up under 50% of cases of structural heart lesions, even in experienced hands ( ; ). Heart lesions picked up by a four-chamber screening scan are generally the more complex ones with a poorer outlook both prenatally and after delivery. If views of the outflow tracts are obtained in addition to the four-chamber view, the range of abnormalities detectable is increased ( ). In particular, transposition, tetralogy of Fallot and similar lesions should be detected.
Detailed evaluation of fetal cardiac anatomy by ultrasound is much more time-consuming and is usually reserved for women with an increased risk of having a fetus with a cardiac problem ( Table 28.2 ). However, when detailed echocardiography was used on all pregnancies, reported 86% sensitivity and 100% specificity. The conditions missed were mainly atrial septal defects (ASDs) and small ventricular septal defects (VSDs). Other workers have pointed out the difficulty in diagnosing coarctation and total anomalous pulmonary venous connection (TAPVC) in the fetus ( ). Abnormalities of fetal heart rhythm can be determined by M-mode and Doppler studies.
|
There are ethical aspects to fetal diagnosis and practitioners in this field need to be sensitive to parental views. Fetal cardiac scanning should be carried out in the context of expertise in all aspects of fetal medicine, including the provision of support to families. The association with non-cardiac abnormalities is strong and these are often major influences in the natural history in utero and after birth, as well as in decisions about continuation of pregnancy. For example, in one study in which cardiac scans were performed for a variety of indications, 70% of fetuses with a cardiac anomaly had an underlying chromosome abnormality ( ).
Treatment
Accurate diagnosis of structural heart disease in a fetus allows information to be given to families and provides for greater choice. Some parents will opt for termination of pregnancy and about 5% of fetuses with structural cardiac abnormalities will die in utero ( ). Cardiac diagnosis and prognosis must be considered in the context of full fetal assessment, as non-cardiac abnormalities may well be more important in determining the overall prognosis. The combination of a structural cardiac abnormality and an extracardiac abnormality will often influence outcome more adversely than anticipated for either lesion alone.
In pregnancies progressing to viability, a cardiac diagnosis will, in a minority of cases, influence place, time or mode of delivery, but non-cardiac factors also need to be taken into account. The most important point with respect to early postnatal management of heart disease is whether or not the lesion is likely to be duct-dependent, thereby allowing prostaglandin to be used before symptoms develop. Progressive underdevelopment of the ventricle in the fetus with severe arterial valve stenosis is well recognised ( ; ) and prenatal arterial valve balloon valvuloplasty has been performed with limited proven efficacy ( ; ). Some structural lesions do not progress with advancing gestation and, in those that do, only a tiny minority are suitable for any form of prenatal intervention. There is evidence that fetal diagnosis of severe duct-dependent congenital heart disease (CHD) results in babies reaching a cardiac centre in better condition than those not diagnosed until after delivery ( ). Benefit from fetal diagnosis in terms of improved survival and morbidity has been shown for coarctation ( ), and in improved mortality in transposition ( ) and hypoplastic left heart ( ), although, in the last two conditions, the benefit from prenatal diagnosis has been difficult to demonstrate ( ). Reassurance of normality or the chance to prepare for the arrival of an abnormal baby is valued by many parents but has not been formally quantified.
Accurate diagnosis of fetal dysrhythmias is essential to avoid unnecessary intervention and to allow appropriate treatment which can almost always improve the outlook for the fetus with a haemodynamically compromising rhythm disturbance. Diagnosis and management of abnormal fetal rhythms are discussed in the section on neonatal rhythm disturbances ( p. 661 ).
Incidence and aetiology of fetal and neonatal heart disease
Structural CHD occurs in approximately 8 per 1000 live births ( ). In populations uninfluenced by fetal diagnosis, between 30% and 40% of these children will be symptomatic in early infancy and about two-thirds of them will have been diagnosed by the end of the first year of life. There are various studies on the prevalence of CHD: diagnostic methods and criteria vary, but Table 28.3 gives figures for those presenting in the neonatal period. The true prevalence may be higher than this, because some lesions such as a bicuspid aortic valve are usually excluded. Bicuspid aortic valve occurs in 10–20 per 1000 live births and may be associated with significant morbidity in later life ( ). An increased prevalence of CHD is also supported by fetal echocardiography data, which have shown that some severe cardiac lesions will result in death in utero ( ). Furthermore, the effect of prenatal diagnosis on postnatal prevalence and spectrum of disease may be considerable ( ).
LESION/GROUP | MEDIAN | 25 TH –75 TH |
---|---|---|
Ventricular septal defect | 32.0 | 27–42 |
Patent arterial duct | 6.8 | 5–11 |
Atrial septal defect | 7.5 | 6–11 |
Atrioventricular septal defect | 3.8 | 3–5 |
Pulmonary stenosis | 7.0 | 5–9 |
Aortic stenosis | 3.9 | 3–6 |
Coarctation of aorta | 4.8 | 4–6 |
Transposition of great arteries | 4.4 | 4–5 |
Tetralogy of Fallot | 5.2 | 4–8 |
Common arterial trunk | 1.4 | 1–2 |
Hypoplastic left heart | 2.8 | 2–3 |
Hypoplastic right heart | 2.2 | 2–3 |
Double-inlet ventricle | 1.5 | 1–2 |
Double-outlet right ventricle | 1.8 | 1–3 |
Total anomalous pulmonary venous drainage | 1.0 | 1–2 |
Others | 10.0 | 8–15 |
Acquired heart disease, such as endocarditis and myocarditis, may present in the newborn period. Metabolic disorders, which may involve heart muscle, can produce symptoms in the newborn period. There are no reliable data for the incidence of these problems or for the incidence of fetal and neonatal arrhythmias.
Although there have been major advances in the diagnosis and treatment of CHD, understanding of its causes remains limited. Advances in cytogenetics have provided a greater understanding of the role of inherited and environmental factors and their interaction on the development of CHD. Environmental factors such as maternal diabetes, alcohol/drug ingestion and, less commonly, some congenital infections are known to affect cardiac development adversely. The major chromosomal abnormalities such as trisomies 21, 18 and 13 cause syndromes, part of which can include structural cardiac malformations, and these have been well documented ( Table 28.4 ). Before the advent of fluorescence in situ hybridisation (FISH), standard chromosome analysis revealed chromosomal abnormalities in about 10% of newborns with CHD ( ). Improved genetic techniques, including FISH, cytogenetic techniques and DNA mutation analysis, have confirmed that this prevalence is much higher. An explanation of the genetic techniques and their uses is beyond the scope of this chapter but readers are referred to the American Heart Association statement on the genetic basis for congenital heart defects ( ). Therefore, chromosomal and genetic analysis in neonates with various types of CHD is an important part of their evaluation, particularly because, in some syndromes, the extracardiac manifestations are often less evident and the cardiac abnormalities provide the clue to the diagnosis in the newborn period.
CHROMOSOMAL DISORDER | MAIN FEATURES | PERCENTAGE WITH CHD | HEART ANOMALY |
---|---|---|---|
Deletion 4p (Wolf–Hirschhorn syndrome) | Pronounced microcephaly, widely spaced eyes, broad nasal bridge (Greek helmet appearance), downturned mouth, micrognathia, preauricular skin tags, elongated trunk and fingers, severe mental retardation and seizures; one-third die in infancy | 50–65 | ASD, VSD, PDA, LSVC, aortic atresia, dextrocardia, TOF, tricuspid atresia |
Deletion 5p (cri du chat) | Cat-like cry, prenatal and postnatal growth retardation, round face, widely spaced eyes, epicanthal fold, simian crease, severe mental retardation, long survival | 30–60 | VSD, ASD, PDA |
Deletion 7q11.23 (Williams–Beuren syndrome) | Infantile hypercalcaemia, skeletal and renal anomalies, cognitive deficits, ‘social’ personality, elfin facies | 53–85 | Supravalvar AS and PS, PPS |
Trisomy 8 mosaicism | Skeletal/vertebral anomalies, widely spaced eyes, broad nasal bridge, small jaw, high arched palate, cryptorchidism, renal anomalies (50%), long survival | 25 | VSD, PDA, CoA, PS, TAPVR, truncus arteriosus |
Deletion 8p syndrome | Microcephaly, growth retardation, mental retardation, deep-set eyes, malformed eyes, small chin, genital anomalies in males, long survival | 50–75 | AVSD, PS, VSD, TOF |
Trisomy 9 | Severe prenatal and postnatal growth retardation, marked microcephaly, deep-set eyes, low-set ears, severe mental retardation; two-thirds die in infancy | 65–80 | PDA, LSVC, VSD, TOF/PA, DORV |
Deletion 10p | Frontal bossing, short down-slanting palpebral fissures, small low-set ears, micrognathia, cleft palate, short neck, urinary/genital, upper limb anomalies | 50 | BAV, ASD, VSD, PDA, PS, CoA, truncus arteriosus |
Deletion 11q (Jacobsen syndrome) | Growth retardation, developmental delay, mental retardation, thrombocytopenia, platelet dysfunction, widely spaced eyes, strabismus, broad nasal bridge, thin upper lip, prominent forehead | 56 | HLHS, valvar AS, VSD, CoA, Shone’s complex |
Trisomy 13 (Patau syndrome) | Polydactyly, cleft lip and palate, scalp defects, hypotelorism, micro-ophthalmia or anophthalmia, colobomata of irides, holoprosencephaly, microcephaly, deafness, profound mental retardation, rib abnormalities, omphalocele, renal abnormalities, hypospadias, cryptorchidism, uterine abnormalities; 80% die in first year | 80 | ASD, VSD, PDA, HLHS, laterality defects, atrial isomerism |
Trisomy 18 (Edwards syndrome) | IUGR, polyhydramnios, micrognathia, short sternum, hypertonia, rocker-bottom feet, overlapping fingers and toes, TEF, CDH, omphalocele, renal anomalies, biliary atresia, profound mental retardation; 90% die in first year | 90–100 | ASD, VSD, PDA, TOF, DORV, D-TGA, CoA, BAV, BPV, polyvalvular nodular dysplasia |
Deletion 20p12 (Alagille syndrome) | Bile duct paucity, cholestasis, skeletal or ocular anomalies, broad forehead, widely spaced eyes, underdeveloped mandible | 85–94 | Peripheral PA, hypoplasia, TOF, PS (left-sided heart lesions and septal defects less common) |
Trisomy 21 (Down syndrome) | Hypotonia, hyperextensibility, epicanthal fold, simian crease, clinodactyly of fifth finger, brachydactyly, variable mental retardation, premature ageing | 40–50 | AVSD, VSD, ASD (TOF, D-TGA less common) |
Deletion 22q11 (DiGeorge, velocardiofacial and conotruncal anomaly face syndrome) | Hypertelorism, micrognathia, low-set posteriorly rotated ears, ‘fish mouth’, thymic and parathyroid hypoplasia, hypocalcaemia, feeding/speech/learning/behavioural disorders, immunodeficiency, palate/skeletal/renal anomalies | 75 | IAA-B, truncus arteriosus, isolated aortic arch anomalies, TOF, conoventricular VSD |
Monosomy X (Turner syndrome, 45,X) | Lymphoedema of hands and feet, widely spaced hypoplastic nipples, webbed neck, primary amenorrhoea, short stature, normal intelligence | 25–35 | CoA, BAV, valvar AS, HLHS, aortic dissection |
Klinefelter syndrome (47,XXY) | Usually normal-appearing, tall stature, small testes, delayed puberty, emotional and behavioural problems common, variable mental retardation | 50 | MVP, venous thromboembolic disease, PDA, ASD |
Syndromes associated with CHD, such as 22q11 deletion, Noonan’s, Williams, Alagille’s, Holt–Oram and others, have been shown to be associated with subtle chromosomal or genetic abnormalities such as deletions or point mutations ( Tables 28.4 and 28.5 ). In some defects there are no associated syndromes but specific genes have been identified in association with particular defects ( Tables 28.4 and 28.5 ). For individuals with CHD or their families, the identification of a genetic cause for the CHD is beneficial. It helps the clinician to explain the mechanism to the family and broadens the context of the diagnosis to other family members.
CONDITION | DEFECT | CHROMOSOME LOCATION | GENE |
---|---|---|---|
Syndrome | |||
Noonan | PS, ASD, HCM | 12q24 | PTPN11 |
12p1.21 | KRAS | ||
2p21 | SOS1 | ||
8q12 | CHD7 | ||
Holt–Oram | ASD | 12q24 | TBX5 |
Marfan | MVP, Ao root dilatation | 15q21.1 | FBN1 |
Alagille | PS, peripheral PS, PA | 20p12 | JAG1 |
CHARGE association | VSD, AVSD, IAA | 8q12 | CHD7 |
Ellis van Creveld | ASD, VSD, AVSD | 4p16 | EVC, EVC2 |
Costello | HOCM | 11p15.5 | HRAS |
Defect | |||
Fallot | 8q23 | ZFPM2/FOG2 | |
AVSD | 3p21 | CRELD1 | |
TGA | 12q24 | PROSIT240 | |
ASD/VSD | 8p23 | GATA4 | |
Supravalvar AS | 7q11 | ELN | |
Atrial isomerism | Xq26 | ZIC3 | |
2q21 | CFC1 | ||
3p21.3p22 | ACVR28 | ||
1q42.1 | LEFTYA |
Such advances have altered the traditional view of a multifactorial basis for the aetiology of CHD shifting towards a genetic aetiology for an increasing number of lesions. Associated with this is the potential to provide more accurate data about recurrence risk in siblings and offspring of affected parents: this has been shown to be higher when the affected parent is female ( ).
Clinical implications of adaptation to birth
Normal adaptation
Normal adaptation of the fetal circulation at birth may have adverse haemodynamic effects in some congenital cardiac malformations just as failure of normal changes to occur may be disadvantageous under certain circumstances.
Foramen ovale
If exit of blood from the left atrium through the mitral valve is impaired, the presence of a foramen ovale is important in allowing decompression of the left atrium as it is forced open by abnormally high pressure in the left atrium. Failure of this mechanism by virtue of a small foramen ovale results in pulmonary venous hypertension and respiratory distress. This problem is an important part of the pathophysiology of mitral atresia or hypoplasia. A restrictive foramen ovale is also important in obstructive lesions in the right heart, such as tricuspid atresia, pulmonary atresia with intact ventricular septum and critical pulmonary stenosis. In such circumstances, right atrial enlargement and hydops fetalis may occur in utero, but, even if this does not occur, there may be postnatal problems from systemic venous engorgement and poor cardiac output. In the context of TGA, a small foramen ovale results in poor mixing of oxygenated and deoxygenated blood. In many of these conditions, particularly TGA, enlargement of a small foramen ovale by balloon atrial septostomy is an important part of the initial management. In some circumstances, surgical septostomy or septectomy may be indicated, as in mitral atresia. Failure of the foramen ovale to close has the same consequences as the presence of an ASD; indeed, distinguishing between patent foramen ovale (PFO) and ASD in newborn infants can be difficult. The consequences are right-to-left shunting in the presence of structural or functional obstruction to right heart flow and left-to-right shunting otherwise. The balance between favourable and deleterious effects is different for different lesions. In general, left-to-right shunting at atrial level in early infancy is rarely a major disadvantage, whereas right-to-left shunting will worsen systemic arterial desaturation – but this may be less of a disadvantage than very high venous pressures or poor left ventricular filling resulting from poor forward flow through the right heart.
Ductus venosus
Closure of the ductus venosus is of importance in that it removes the possibility of central venous access being obtained via the umbilical vein for monitoring, balloon septostomy or cardiac catheterisation. It will also result in marked deterioration in cases of TAPVC to the portal vein as it will cause severe pulmonary venous obstruction with pulmonary congestion ( Fig. 28.2 ). In this condition, closure of the ductus venosus may not occur until some days after birth. Delay in or failure of closure of the ductus venosus is probably rare and never of significance.


Ductus arteriosus
Closure of the DA will cause marked deterioration in duct-dependent pulmonary and duct-dependent systemic circulations, in the first instance causing worsening cyanosis, and, in the latter, shock and heart failure. Systemic arterial oxygenation in TGA will also deteriorate when the DA closes. These conditions are all discussed in more detail below (see sections on cyanosis and collapse ).
Pulmonary vascular resistance
Pulmonary blood flow increases rapidly at birth as PVR falls. This normal process will have adverse effects if an increase in pulmonary venous return is disadvantageous. As discussed above, this can happen in the presence of pulmonary venous obstruction from any cause or if exit of blood from the left atrium is impeded. Increased pulmonary blood flow secondary to lesions allowing left-to-right shunting will cause respiratory distress and heart failure as PVR falls. This usually is not a problem in the early newborn phase and often is not apparent until 2 or 3 months of age. A common exception to this statement is patent ductus arteriosus (PDA) in the preterm infant (see below). Any shunt lesion may become symptomatic at an earlier postnatal age in preterm infants than in term ones; this is attributed to less well-developed pulmonary vascular musculature in the premature infant ( ). It is also probable that widespread use of more effective mechanical ventilation and the use of surfactant may improve oxygenation, facilitating the decrease in PVR and contributing to the pathophysiological effects of a patent arterial duct in premature newborns.
Delay in the normal fall in PVR may quickly result in a clinical problem (persistent pulmonary hypertension of the newborn, PPHN). It may also play a role in the pathophysiology of chronic lung disease (CLD) and in the development of pulmonary vascular disease (PVD) in structural heart lesions, owing to unrestricted transmission of systemic pressures into the pulmonary circulation, as occurs in a large VSD or a complete atrioventricular septal defect (AVSD).
PPHN may also be seen when there has been underdevelopment of the pulmonary vascular tree in utero (such as in diaphragmatic hernia) or if there has been in utero exposure to prostaglandin synthetase inhibitors as a result of maternal therapy. Hypoxaemia in PPHN is due to right-to-left shunting, which can be either within the lungs or extrapulmonary, in which case it can be at ductal level or intracardiac through the foramen ovale.
History and examination
These are basic in the evaluation of a newborn infant with suspected cardiac disease. History-taking involves details of the current problems as well as obtaining information about pregnancy, the perinatal period, the family and social circumstances. Examination of the newborn is dealt with in Chapter 14 ; a number of additional points with specific reference to the cardiovascular system will be considered here.
Dysmorphic features and non-cardiac malformations
These must be carefully sought and described in detail in relation to all body systems. They are clearly important if a syndrome is to be diagnosed and may influence treatment plans and prognostic information given to families. It may be relevant to investigate other systems on the basis of certain cardiac diagnoses, for example chromosome analysis or immunological assessment. In some circumstances, recognition of an abnormality in an infant will result in a diagnosis being made in a parent, for example 22q11 deletion or Noonan syndrome. When certain non-cardiac structural abnormalities are recognised, a detailed cardiac evaluation is appropriate, as in Down syndrome and many gastrointestinal abnormalities ( ). Of all children with chromosomal abnormalities, at least 30% have a congenital cardiac defect ( ).
Pulses
Impalpable or weak femoral pulses in the presence of good-volume upper limb pulses suggest coarctation of the aorta, in which case other signs may well be present and might include upper limb hypertension, systolic pressure difference between arms and legs, evidence of aortic valve abnormality (ejection click), a bruit between the scapulae and signs of heart failure. If all pulses are weak, then left ventricular outflow obstruction, hypovolaemia or left ventricular dysfunction should be considered. Hypoplastic left heart may be associated with stronger femoral than right brachial pulses before the DA closes. A preterm infant severely compromised by PDA may have very weak femoral pulses. A baby with interrupted aortic arch usually collapses in the first week of life; the diagnosis and the site of interruption can sometimes be deduced by comparing arm and neck pulses as they will be much stronger proximal to the interruption.
Blood pressure
Blood pressure (BP) should be measured in both arms and in one leg in any newborn infant with cardiac symptoms and in an asymptomatic infant if other signs raise the possibility of coarctation. A difference in systolic BP of 20 mmHg or more between arm and leg is strongly suggestive of coarctation, although smaller gradients in term babies can be normal and the absence of such a difference may not rule out the diagnosis of coarctation if the DA is still patent. BP should also be measured in any unwell baby, as well as in those with urinary tract abnormalities, those on steroids, in CLD and in the infants of drug-addicted mothers. It is essential that the baby is settled; results on crying babies are misleading. Monitoring BP is part of the management of severely ill babies and this should be carried out invasively wherever possible. Obtaining a definitive non-invasive BP requires patience and attention to detail. The arterial pulse can be detected by palpation or with a Doppler probe. Both methods will only give a systolic value. Auscultation is very difficult and the flush method is rarely used. Oscillometric monitors may be useful in sequential BP monitoring of immobile babies but even then are subject to error at low pressures in infants with extremely low birthweight. Cuff size is important and for the arm it should cover 75% of the distance from axilla to elbow or have a width that is 40–50% of the arm circumference ( ). The bladder should virtually encircle the arm. Leg BP can be measured using the same cuff around the calf with detection of a dorsalis pedis or posterior tibial pulse. Normal BP values are given in Appendix 4 .
Cyanosis
Peripheral cyanosis is very common in normal newborn babies. Central cyanosis in the presence of structural cardiac disease is caused by three main mechanisms, which may coexist. The commonest is obstruction to pulmonary blood flow with a right-to-left shunt. Cyanosis is also evident when there are discordant ventriculoarterial connections (as in transposition) with adverse streaming of blood within the heart. Thirdly, cyanosis will also occur where there is common mixing of blood, which can occur at atrial, ventricular or great artery level, allowing the mixed systemic and pulmonary venous return to be distributed to both the aorta and the pulmonary arteries.
Central cyanosis in the neonate may have a respiratory cause but can also be mimicked by facial petechiae (traumatic cyanosis) and can be seen in markedly polycythaemic infants. Plethoric infants with a low normal oxygen saturation may have enough deoxygenated haemoglobin to give true central cyanosis. Pigmentation of the lips can also confuse the observer and it is important to look at the tongue to get the best possible assessment of saturation. Anaemia and desaturation make a baby look pale grey rather than really blue, and methaemoglobinaemia gives babies a slate black or grey colour which is often mistaken for cyanosis. Cyanotic, often termed ‘dusky’, episodes are very common and are only occasionally a presenting feature for structural heart disease; persistent cyanosis is far more likely to be cardiac, although it may vary in intensity. Cyanosis whilst crying is rarely pathological if colour and behaviour return to normal rapidly when the infant stops crying. Hypercyanotic episodes, as seen in tetralogy of Fallot and related conditions, are rare in the newborn period.
Heart failure
Fetal echo has shown that prenatal cardiac failure can be caused by myocardial dysfunction, structural abnormalities and arrhythmias. In newborns, early heart failure usually results from myocardial dysfunction, left-heart obstructive lesions, tachyarrhythmias and large arteriovenous malformations (AVMs). These result in tachycardia, tachypnoea with recession, liver enlargement and cardiomegaly. These conditions are a medical emergency. With advancing age, lesions that cause a large left-to-right shunt are the most frequent cause of heart failure (large VSD or big PDA). They manifest as PVR falls and signs appear more quickly when there are combinations of lesions. The signs of heart failure in the older neonate are subtly different, with poor feeding, slow weight gain and tachypnoea being the most common. Common features of neonatal heart failure are listed in Table 28.6 .
|
Heart murmur
Heart murmurs in the newborn are common, occurring in up to 0.6% of newborns ( ). They may be associated with both major and minor cardiac lesions but also can be a normal physiological finding. Many serious cardiac conditions have unimpressive or even no murmurs in the newborn period. Other auscultatory and general cardiovascular signs are therefore very important in assessing the significance of a murmur. The absence of any other features of cardiac disease as well as the presence of certain positive murmur characteristics are required to diagnose innocent heart murmurs. The typical heart murmur in newborn babies comes from the pulmonary artery branches and disappears before 6 months of age in term infants. In practice, cardiac murmurs in the newborn that are associated with additional cardiac symptoms or signs justify prompt echocardiographic assessment unless the diagnosis has been documented prenatally, in which case knowledge of the diagnosis and clinical assessment should determine the timing of investigation. Asymptomatic murmurs will almost always need formal investigation with echocardiography but this can usually be undertaken on an elective basis.
Airway obstruction
This uncommon presentation is usually associated with inspiratory stridor. When associated with a structural cardiac abnormality, the manifestations are usually caused by a vascular ring such as a pulmonary artery sling or a double aortic arch. Major airway obstruction can occur as part of the absent pulmonary valve syndrome in association with tetralogy of Fallot.
Investigations
History and examination often allow cardiac disease to be suspected or ruled out. Investigations range from those readily available in any neonatal nursery (chest X-ray (CXR; Ch. 27 part 2 ), electrocardiogram (ECG), pulse oximetry and hyperoxia test) to those only available at cardiac centres (cardiac catheterisation and magnetic resonance imaging (MRI)). Echocardiography is being increasingly used outside cardiac centres and, with suitable equipment and trained operators, is very valuable in the care of the newborn, particularly to confirm or rule out a cardiac abnormality in those cases where clinical assessment, ECG and CXR have been inconclusive. The value of ECG and CXR in differentiating normal from abnormal murmurs in the newborn has not been systematically examined, and they are less often used now. The role of pulse oximetry as a screening test is also discussed below.
Chest radiography
CXR is less valuable in the newborn period in the evaluation of CHD because the appearance can be normal even in the presence of a severe congenital cardiac malformation. Its value lies in its availability, cost and the additional information it provides. Visceral situs, cardiac position, cardiac size and bony abnormalities of the chest are all well seen on the CXR. Features to look for in this context are listed in Table 28.7 ; examples are shown in Figures 28.3–28.6 and see Ch. 43 . The role of the CXR has diminished with the more widespread use of transthoracic echocardiography.
FEATURE | COMMENT |
---|---|
Quality of film | Adequate inspiration |
Normal penetration | |
Centred on midchest | |
Not rotated | |
Abdominal situs | |
Bronchial situs | Normal/inverted/ambiguous |
Aortic arch side | Left or right |
Heart | Side |
Direction of apex | |
Size | |
Contour | |
Lung vasculature | Plethora |
Oligaemia | |
Pulmonary venous engorgement | |
Diaphragm | Distinct |
Side of apex should be more caudal | |
Lung fields | Any pathology |
Musculoskeletal | Vertebral/rib abnormalities |
Fractures |




Electrocardiogram
The ECG provides useful information in a number of areas ( Table 28.8 ), providing attention is paid to technical aspects of obtaining a good recording, which can be difficult in a neonatal nursery. Systematic reading of the ECG will optimise the information obtained and reference should be made to normal values ( Appendix 3 ) and to a standard text for detailed interpretation of ECGs ( ).
|
Heart rhythm
Abnormal heart rate can be confirmed on the ECG and heart rhythm can usually be ascertained. Sinus rhythm is characterised by normal P waves (frontal plane axis 0–190°) preceding every QRS complex. Sinus rate varies between 70 and 180 beats/min in healthy babies, reaching as much as 220 beats/min in sick babies. In these circumstances, P waves can be hard to see but all leads should be examined and paper run at a faster speed (50 mm/s) if necessary. First-degree heart block (prolonged PR interval) is rarely of importance in its own right in the newborn but may be a marker for structural heart disease such as ASD or Ebstein’s anomaly. A short PR interval is a marker for an increased tendency to supraventricular tachycardia (SVT), although delta waves in the QRS complex may easily be overlooked in neonates. A short PR interval also accompanies some structural heart lesions (Ebstein’s anomaly) and may be seen in glycogen storage disease. Partial atrioventricular (AV) dissociation (second-degree heart block) and complete heart block (CHB) are considered below, as are SVT and ventricular tachycardia (VT).
Information on the atria
Inverted P waves in lead I may be a sign of an incorrectly wired ECG (right arm/left arm reversed). This can be checked by looking at lead V 6 . If I and V 6 look similar, the ECG is wired up correctly and negative P waves in I then suggest one of the following:
- •
not sinus rhythm ( Fig. 28.7 )
Fig. 28.7
Electrocardiogram leads I, II and III, showing inverted P wave in I. Supraventricular tachycardia at 190 beats/min.
- •
heart in abnormal position
- •
heart in normal position but atria in abnormal spatial relationship to each other.
Right atrial enlargement is indicated by tall (>2.5 mV) P waves and left atrial enlargement shown by broad (<3 mm) P waves at standard paper speed (25 mm/s).
Information on the ventricles
Abnormal ventricular positions within the chest, as in dextrocardia, or in relationship to each other, as in congenitally corrected transposition, can be suspected from abnormalities in QRS progression across the chest leads. In dextrocardia, complexes do not evolve between V 1 and V 6 but simply get progressively smaller. Q waves in V 1 mean one of the following:
- •
abnormal intraventricular conduction (as in left bundle branch block or some cases of pre-excitation)
- •
severe RV hypertrophy (RVH)
- •
spatial relationship between right and left ventricles abnormal (as in congenitally corrected transposition).
RVH is suggested by one or more of the following:
- •
right axis deviation
- •
Q in V 1
- •
large RV 1 or SV 6 ( Appendix 3 )
- •
upright T in V 1 after day 3 ( Fig. 28.8 ).
Fig. 28.8
Electrocardiogram chest leads in a 6-day-old infant. Neonatal R→S progression (normal) but upright T-wave V 1 indicating right ventricular hypertrophy.
It is important to note that conditions causing marked RVH in later infancy may cause no ECG abnormality in the immediate newborn period and only an upright T wave in V 1 in the later newborn period.
Left ventricular hypertrophy is suggested by one or more of the following:
- •
adult R/S progression V 1 to V 6 (dominant SV 1 dominant RV 6 )
- •
large SV 1 or RV 6 ( Appendix 3 ).
Biventricular hypertrophy is indicated by a combination of these findings.
Ventricular strain or ischaemia is indicted by ST depression or T-wave inversion in left chest leads (II, aVL, V 5–6 ) and may point to a primary cardiac muscle disorder or be secondary to a severe structural abnormality causing pressure or volume load on the heart. T-wave changes are seen after perinatal stress and resolve in under a week. Pericarditis is rare in the newborn period but ST segment and T-wave changes are seen.
QRS axis
The frontal QRS axis can be calculated as shown in Figure 28.9 . An abnormal QRS axis may be an important diagnostic clue in a number of conditions, including AVSD ( Fig. 28.10 ) and tricuspid atresia (QRS usually −30°); in the former case it makes the ECG a valuable screening investigation in newborn infants with Down syndrome, in whom clinical features of AVSD may be absent or very subtle.


- 1
Identify the lead in which the R and S waves are most nearly of equal size.
- 2
Look at right angles (190° and 290°) to the lead identified in step 1.
- 3
One of the leads identified in step 2 will be predominantly positive, the other predominantly negative.
- 4
The predominantly positive lead identified by step 3 is approximately the QRS axis (depolarisation towards a lead produces a positive deflection).
- 5
The approximate QRS axis obtained from step 4 can be made more accurate by estimating the equiphasic lead (step 2) more accurately by ‘imagining’ it between actual leads.
Pulse oximetry
Pulse oximetry has been advocated as a screening tool for CHD in newborns. Because newborns with complex CHD will often deteriorate within 48 hours of age, oximetry would be performed ideally within 24 hours of birth. However, saturations in newborns vary considerably during the first 24 hours, which can increase the false-positive rate. Most healthy newborns are now discharged before 24 hours of age, which limits the use of this technique. Oximetry should be performed on the foot of a baby breathing air. A value of less than 95% is found in about 5% of apparently well infants ( ). If these infants are further evaluated (by repeating the test initially), few cases of important structural heart disease are overlooked and some other potentially unwell infants are also detected. The American Heart Association and American Academy of Pediatrics have recently reviewed the question of pulse oximetry screening after 24 hours of age in some detail ( ). Ten studies with a population of 123 846 infants were summarised, with a false-positive rate of 0.035% when screening was done after 24 hours. Most studies used a cut-off of 95%. The detection rate for many conditions was good, but the authors conclude that additional studies are needed to determine whether this practice should become a standard of care.
Hyperoxia test and blood gas analysis
The hyperoxia (or nitrogen washout) test was described in the era before echocardiography was available and was designed to help distinguish cardiac from respiratory cyanosis without resorting to cardiac catheterisation. Babies with cyanotic heart disease usually show little rise in arterial oxygen tension in response to increased inspired oxygen. A rise of P a o 2 to a value below 20 kPa after 10 minutes in 85% or more inspired oxygen makes heart disease likely, whereas an increase in P a o 2 above 20 kPa makes respiratory disease likely. There are some exceptions to this pattern in that babies with desaturating cardiac disease with high lung blood flow (as in unobstructed TAPVC and double-inlet ventricle, for example) will pass, whereas those with very severe respiratory disease and with PPHN may fail.
When it is essential to have an accurate measurement of arterial oxygen content, an arterial blood sample must be obtained, and further information of importance in the management of ill infants will also be available from a blood gas analysis. Transcutaneous oxygen tension monitors placed on the right upper chest (that is, preductal) can be used instead of arterial sampling as a screening test if other information is not required. Oxygen pulse saturation monitors will not provide the same assurance of a pass, as an infant will show 100% saturation at a P a o 2 value well below 20 kPa. The role of the hyperoxia test has therefore changed since its inception, but it is still helpful in two circumstances:
- 1
When a well baby seems dusky and there is uncertainty as to whether a cyanotic cardiac condition is present. A failed transcutaneous or pulse oximeter hyperoxia test strongly points to cyanotic heart disease.
- 2
A well baby with signs of heart disease who looks pink may fail the hyperoxia test, thus alerting the physician to the presence of a more complex lesion.
Echocardiography
Transthoracic echocardiography has revolutionised the diagnosis and management of neonatal heart disease. The non-invasive, immediate and portable nature makes this modality ideally suited to the investigation of even the smallest babies. It can define structure and function and has resulted in a dramatic reduction in the need for diagnostic cardiac catheterisation. It is now widely used to guide interventions such as balloon atrial septostomy on the intensive care unit.
The sequential and segmental approach forms the basis of diagnosis of all CHD. A detailed description of this is beyond the scope of this chapter but can be found in . This systematic approach ensures that subtle complex problems are not overlooked even in the most complex malformations. All examinations should begin with indirect evaluation of atrial situs using the great vessels at the level of the diaphragm ( Figs 28.11 and 28.12 ). This is followed by establishing the position of the cardiac apex in the chest from a subcostal view. The same echocardiographic window allows identification of the systemic and pulmonary venous drainage and evaluation of the atrial septum. This is followed by establishing the AV connection and assessment of the AV valves. Next, the position and morphology of the ventricles are established and the ventricular septum is assessed; this is most easily viewed from the cardiac apex. Following this, the ventriculoatrial connections are documented together with the relationship of the great arteries. Assessment of the semilunar valves is followed by documentation of the coronary arteries. From the parasternal views, the branch pulmonary arteries are identified and the aortic arch with the head and neck vessels seen from the suprasternal position. Finally, assessment of function is evaluated using m-mode from a parasternal long-axis view.


All ultrasound modalities have a role in evaluating the cardiovascular system ( Table 28.9 ). Newer ultrasound modalities such as three-dimensional and four-dimensional imaging as well as new Doppler techniques including Doppler tissue imaging and strain imaging are becoming more widely used in the management of CHD but have limited applications in routine neonatal practice at present. More detailed descriptions of these techniques are found in other sources ( ).
MODALITY | USES |
---|---|
Two-dimensional imaging | Defining anatomy |
Chamber size, wall thickness | |
Function (qualitative) | |
Doppler | Assessment of direction and velocity of flow, turbulence and flow patterns |
Colour flow | Rapid identification of site of abnormal flow patterns and flow direction |
Detection of regurgitation and small shunts | |
Pulsed wave | Sampling flow patterns in a localised region |
May be inaccurate with high velocities | |
Calculation of cardiac output | |
Continuous wave | Excellent for high velocities |
Abnormal flow patterns not precisely localised | |
M mode | Dimensions and function |
Standard two-dimensional imaging gives anatomical detail. Doppler identifies or clarifies shunt lesions and regions of turbulent flow and allows quantification of stenosis by measuring blood velocity. This enables calculation of a pressure difference across a valve or between ventricles through a VSD using the modified Bernoulli equation ( P = 4 V 2 , where P = instantaneous peak systolic pressure gradient in mmHg, V = velocity distal to the site of obstruction in m/s).
Standard echocardiographic windows and images of the heart are shown in Figure 28.13 ; examples of clinical scans are given in Figures 28.14–28.16 . Transthoracic echocardiograms may be technically difficult in babies with severe respiratory disease on assisted ventilation. Many of these infants are premature and of low birthweight. As echocardiographic skills become more widespread, telemedicine provides the means whereby neonatal units can collaborate with cardiac centres to analyse recorded echocardiographic studies, helping in the decision-making about whether a baby needs transfer to a tertiary cardiac unit ( ).















Cardiac catheterisation
Cardiac catheterisation and angiography was once the principal means of investigating babies with CHD. It may be diagnostic for anatomical or haemodynamic information or may be therapeutic. Diagnostic catheterisation is now rarely required in the newborn baby with present-day ultrasound capability and other non-invasive imaging modalities. When it is, usually only a small amount of information is required in order to complement that already obtained by echocardiography. Cardiac catheterisation in small children carries a small but definite risk and almost inevitably requires general anaesthesia. This will influence cardiac physiology and the relevance of the information obtained.
Magnetic resonance and computed tomographic imaging
MRI provides excellent anatomical images, but, in the newborn, echocardiography often provides adequate information. The range of uses for cardiac MRI scanning in the newborn is continuing to expand as greater experience is obtained in older children and scanning technology improves. It can provide useful non-invasive anatomical information about pulmonary arterial abnormalities previously only obtainable from angiography. MRI involves no radiation hazard, but does require sedation or general anaesthesia in an environment where detailed monitoring is rather more complicated to achieve. In contrast, computed tomographic (CT) imaging, which requires shorter acquisition times and can frequently be performed with sedation alone, does have a role as a non-invasive imaging technique in neonates. It provides excellent information about aortic arch anatomy and other vascular structures ( Fig. 28.17 ) and the relationship of the vascular structures to the airways but its drawback is the radiation dose required to acquire such images.



Treatment
General principles
In principle, medical management for CHD is largely supportive (e.g. heart failure) whereas significant structural abnormalities usually require intervention. Continued improvement in surgical results for congenital cardiac malformations has been one of the triumphs of modern paediatric cardiology. Surgical mortality for malformations, which were, up to recently, considered untreatable (e.g. hypoplastic left-heart syndrome (HLHS)) is now very acceptable. In addition, improvements in surgical results have meant a major shift in surgical emphasis away from surgical palliation towards primary neonatal correction of structural abnormalities with restoration of normal physiology wherever possible. This has been further facilitated by the advances occurring in neonatal intensive care both pre- and postoperatively.
Attention to the general aspects of medical care to achieve stability and optimise condition prior to transfer and intervention of any kind is a very important aspect of minimising morbidity and mortality for any cardiac condition. There are some specific areas related to the care of neonates with cardiac disease that require further mention.
Ductal manipulation
Establishing and maintaining an open DA is a crucial part of the resuscitation of babies with duct-dependent pulmonary or systemic circulations and usually greatly improves oxygenation in TGA. Prostaglandin E 1 and E 2 will both dilate the DA. Dosage regimens for both drugs are given in Table 28.10 . Acute side-effects of prostaglandin include apnoea, jitteriness, convulsions, diarrhoea, flushing and fever and are dose-dependent. These are usually manageable without loss of therapeutic effect by stopping the infusion for a few minutes and restarting it at a lower dose. Long-term oral or nasogastric use is described ( ) but of limited value. Manipulation of the DA in order to close it is considered on pages 645–647 .
DRUG AND ROUTE | DOSE | COMMENT |
---|---|---|
PGE 1 i.v. | 0.005–0.1 µg/kg/min | For severe cyanosis or shock, start at higher dose range for both drugs and increase at 15–30-minute intervals to maximum if no response. More than threefold increase in dose rarely needed. Side-effects increase with higher doses |
PGE 2 i.v. | 0.005–0.05 µg/kg/min |
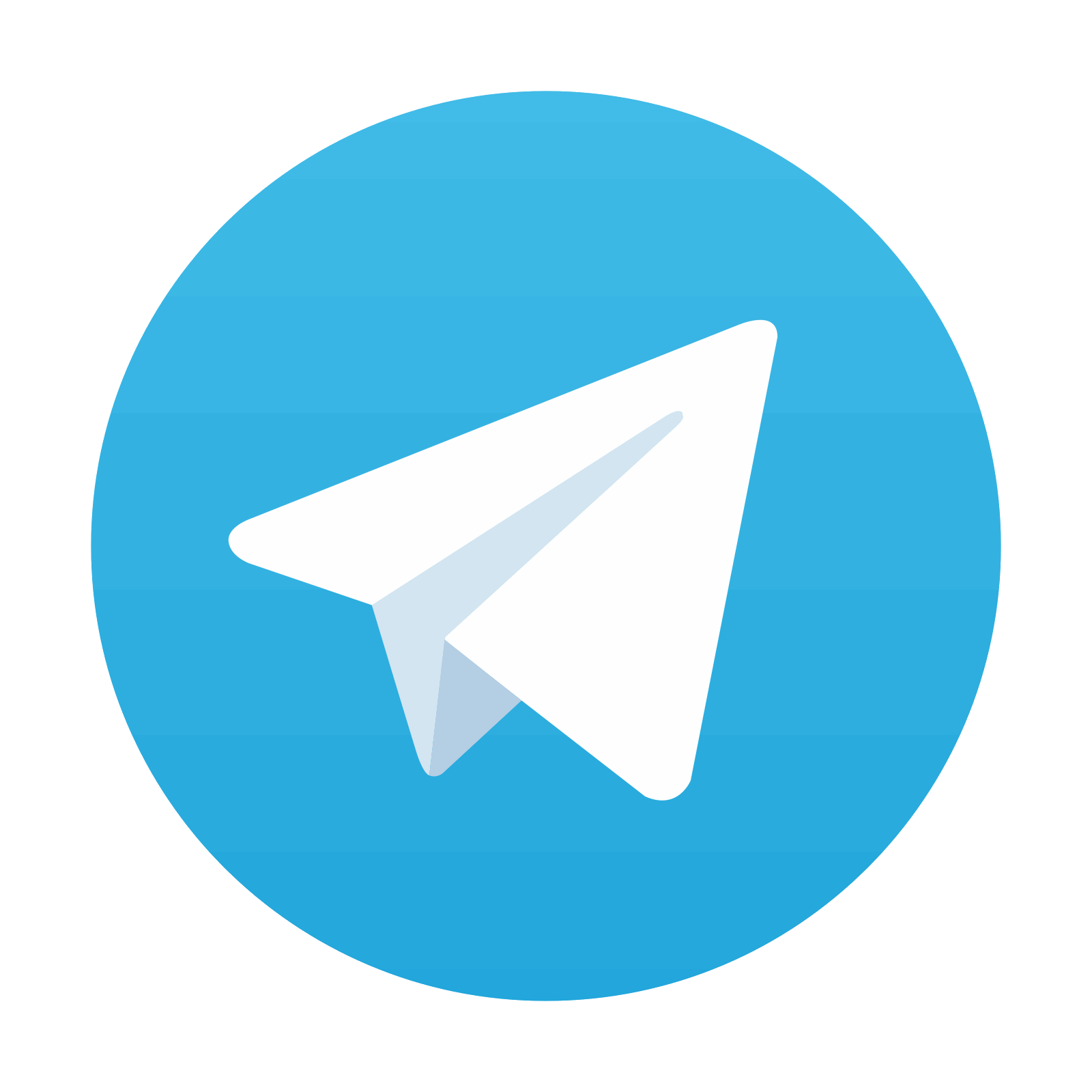
Stay updated, free articles. Join our Telegram channel

Full access? Get Clinical Tree
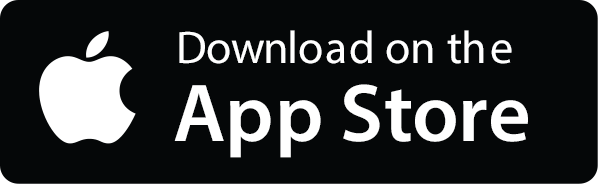
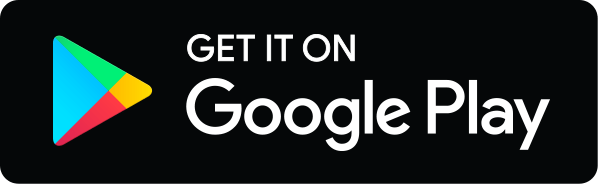
