Cancer is the second-leading cause of death in the United States. The lifetime probability of a women being diagnosed with an invasive cancer is 38%.1 Up to 20% of all cancers might be caused by excessive weight (overweight or obesity).2 The burden of obesity on society continues to rise and warrants closer attention by clinicians for both cancer prevention and improved outcomes after diagnosis. The general focus of this chapter is the association of obesity with nongynecologic cancer in women. The mechanisms by which body weight influences the development of cancer are discussed. Particular emphasis is placed on the impact of increased body weight on prognosis, survival, and risk of recurrence. In addition, prevention and management strategies are discussed to aid clinicians in counseling patients regarding obesity-related cancers.
Surveillance, Epidemiology, and End Results (SEER) data estimates as of 2007 suggest that there will be 50,000 new cases of cancer yearly in women (7%) that are attributable to obesity.2 More than one-third of US adults are obese (approximately 78.6 million), another third are overweight,3 and the prevalence is increasing. The percentage of cancer cases attributable to obesity varies widely for different cancer types but is as high as 40% for some cancers, particularly adenocarcinomas of the endometrium and esophagus. A projection of the future health and economic burden of obesity in 2030 estimated that continuation of existing trends in obesity will lead to about 500,000 additional cases of cancer in the United States by 2030 (Table 12–1).4
Cancer Type | New Cases | Percentage Prevented | Number of Cases Prevented |
---|---|---|---|
Breast, female | 232,670 | 33 | 76,781 |
Prostate (advanced) | 16,310 | 11 | 1,794 |
Gallbladder | 10,650 | 21 | 2,237 |
Endometrial | 52,630 | 59 | 31,052 |
Esophageal | 18,170 | 63 | 11,447 |
Colorectal | 136,830 | 50 | 68,415 |
Ovarian | 21,980 | 5 | 1,099 |
Pancreatic | 46,420 | 19 | 8,820 |
Mouth, pharyngeal, and laryngeal | 38,960 | 63 | 24,545 |
Kidney | 63,920 | 24 | 15,341 |
Liver | 33,190 | 15 | 4,979 |
Stomach | 22,220 | 47 | 10,443 |
Lung | 224,210 | 36 | 80,716 |
Total preventable | 337,667 |
A systematic review and meta-analysis were performed in 2008 to assess the strength of associations between body mass index (BMI; defined as weight in kilograms divided by height in square meters) and different sites of cancer and to investigate differences in these associations between sex and ethnic groups.5 In women, strong associations were noted between a 5-kg/m2 increase in BMI and endometrial (relative risk [RR] 1.59, confidence interval [CI] 1.5–1.68), gallbladder (RR 1.59, CI 1.02–2.47), and renal (RR 1.34, CI 1.25–1.43) cancers and esophageal adenocarcinoma (RR 1.51, CI 1.31–1.74). A weaker positive association (RR < 1.20) was observed between increased BMI and postmenopausal (PMP) breast, pancreatic, thyroid, and colon cancers in women and for leukemia, multiple myeloma, and non-Hodgkin lymphoma in both sexes.
Although the relationship between obesity and cancer in women is well established, the biologic mechanisms underlying this relationship are just beginning to be elucidated. “Obesity-related cancer” is now an official designation used by the American Cancer Society and the National Cancer Institute. The list of obesity-related cancers is long and growing, with lung cancers a prominent exception to the obesity association. It is now estimated that 20% of cancer deaths are attributable to obesity-related oncogenesis. In the case of breast cancer, it was thought for many years that excess estrogen produced by adipose tissue was the major contributor to this increased risk. This was a gross oversimplification of the etiology of breast cancer, and now that we understand more about adipose tissue as an endocrine organ in and of itself, it is becoming apparent that estrogen excess may be an indirect, rather than direct, oncogenic mechanism. The metabolic abnormalities associated with excess adipose tissue and the location of that adipose tissue, and not diet or adiposity itself in most cases, are direct contributors to oncogenesis in many tissues. These metabolic abnormalities occur at both the local and systemic levels, and the variable effect of each may affect tissue-specific susceptibility to carcinogenesis.
There are several pathways by which obesity contributes to excess cancer risk; these are interrelated and synergistic, including localized inflammatory changes and cytokine release, hyperinsulinemia, high leptin levels, and epigenetic changes. These are discussed in further detail in the following material.
One of the primary processes that occurs in adipose tissue of obese individuals is enlargement of adipocytes. When adipocytes reach a critical size, their blood supply is diminished, leading to localized tissue hypoxia. This tissue hypoxia and resultant tissue necrosis set off a cascade of reactions, including release of free fatty acids (FFAs) due to lipolysis, macrophage infiltration, and release of cytokines and adipokines, most importantly tumor necrosis factor alpha (TNF-α) and interleukin 6 (IL–6). Histologically, this inflammatory response can be identified as crown-like structures, or CLS-B, which are inflammatory foci that consist of a necrotic adipocyte and its surrounding macrophages. The release of inflammatory factors by these adipocytes contributes to both cancer initiation and promotion by increasing cell migration and cell invasion. The progression of these changes is shown schematically in Figure 12-1.6
FIGURE 12-1.
Relationship between obesity, metabolic function, and inflammatory changes at the level of the adipocyte. SFRPF5: secreted frizzle-related protein 5; RBP4: retinol binding protein 4; ANGPTL2: angiopoietin-like protein 2; CCL2: chemokine ligand 2; CXCL5: CXC chemokine ligand 5; NAMPT: nicotinamide phosphoribosyltransferase; IL-6: Interleukin 6. (From Ouchi N, Parker JL, Lugus JJ, Walsh K. Adipokines in inflammation and metabolic disease. Nature Rev Immunol. 2011;11:85–97.)

Obesity leads to insulin resistance and the resultant increase in serum insulin causes elevations in insulinlike growth factor 1 (IGF-1) and leptin levels and, in the case of mild insulin resistance, increased adiponectin. Hyperinsulinemia causes activation of several pathways, such as via IRS, ERK, and MYC, which increase cellular mitogenicity and cell growth and are pro-angiogenic and antiapoptotic. As insulin resistance worsens, the process shifts even further to a pro-inflammatory state.
Leptin is an important modulator of immune function through cytokine production, angiogenesis, and mitogenesis. TNF–α, which is produced by infiltrating macrophages in response to chronic inflammation in adipose tissue, leads to increases in leptin secretion, as do insulin, estrogen, and glucocorticoids. Excess TNF-α stimulates the nuclear factor kappa beta (NF-κβ) signaling pathway, which promotes cell migration and cell invasion, both pro-metastatic functions. The secretion of adiponectin, which serves to balance the effects of leptin, is decreased in the presence of these inflammatory factors. Adiponectin blocks induction of vascular endothelial growth factor (VEGF) through its suppression of TNF-α. This effect inhibits migration of vascular endothelial cells and promotes apoptosis.
As insulin resistance worsens, the ratio of adiponectin to leptin changes in favor of pro-inflammatory metabolism and the loss of the protective benefits of adiponectin. In the case of breast cancer, adiponectin levels correlate with improved survival. With unfavorable levels of adiponectin, a positive-feedback loop is created in adipose tissue that favors progression of the cycle of inflammation, cytokine release, and leptin release, leading to a pro-tumorigenic environment.7
Through its activation of kinases, such as Jak (Janus kinase)/STAT (signal transducer and activator of transcription), mitogen-activated protein kinase (MAPK), PI3/AKT, and others, leptin influences cell proliferation and survival. In addition, leptin has been shown to stimulate all steps in the angiogenesis cascade.8 Once a tumor is initiated, this feedback loop also promotes tumor progression by amplifying these factors in the tumor microenvironment (enhanced production of VEGF, NF-κβ, and others that promote angiogenesis, mitogenesis, and vascular permeability). A diagrammatic summary of these factors is shown in Figure 12-2.9
FIGURE 12-2.
Interaction of insulin and leptin on cellular growth factors. LKB1: serine threonine kinase; AMPK: activated protein kinase; TOR: threonine kinase; NO: nitric oxide; eNOS: endothelial nitric oxide synthase; PTEN: phosphatase and tensin homolog; STRAD/MO25: Ste20 related adaptor protein; mTOR: mammalian target of rapamycin; RAPTOR: regulatory associated protein of mTOR; RICTOR: rapamycin-insensitive mammalian target of rapamycin; RHEB: Ras homolog enriched in brain; Ras: rat sarcoma; PIP3: phosphatidylinositol 3,4,5 triphosphate; GTP: guanosine 5-triphosphate; ATP: adenosine triphosphate; AMP: adenosine monophosphate; TSC1: tuberous sclerosus protein 1; TSC2: tuberous sclerosus protein 2; GLUT1: human glucose transporter; eIF4E: eukaryotic translation initiation factor 4E; eIF4B: eukaryotic translation initiation factor 4B; 4E-BP1: 4E binding protein 1; AKT: protein kinase B; PI3: phosphoinositide 3-kinase; LDH: lactate dehydrogenase cdks: cyclin-dependent kinases; PDK1: phosphoinositide-dependent kinase 1; PI3: phosphoinositide 3 kinase; AKT: protein kinase B. (Redrawn from Sundaram S, Johnson AJ, Makowski L. Obesity, metabolism and the microenvironment: links to cancer. J Carcinogen. 2013;12:19.)

Other factors that may affect the risk of certain cancers include alterations in the microbiome, which may be particularly important in colorectal cancer, and epigenetic changes that promote the development of cancer. We expect that these changes and their effects will be elucidated in the next few years.
The risk of a obese PMP woman developing breast cancer is elevated by 20%–30% over her normal-weight peers. Current estimates suggest that up to 17% of breast cancer cases are preventable by maintenance of a normal weight. This risk seems to be related specifically to women with high central and upper body obesity, characterized by an elevated waist-to-hip ratio (WHR). Women in the lowest quartile for WHR have a decreased risk of developing breast cancer. Weight gain in adult life increases breast cancer risk, while sustainable weight loss after menopause can lead to decreased risk. These associations were particularly strong for weight gain of greater than 25 kg (RR 1.45).10
Breast is a fat-rich organ, and large islands of adipose tissue surround the stroma of the epithelial ducts and lobules. This proximity of biologically active adipose tissue with breast epithelium promotes crosstalk between these tissues that affects the initiation and progression of breast neoplasia. There are also data that confirm an elevated risk of breast cancer in PMP women with insulin resistance and type 2 diabetes mellitus (T2DM), independent of BMI. Emerging evidence suggests that hyperinsulinemia is a major contributor to this risk, and many, if not most, women have high insulin levels 10–15 years prior to the diagnosis of T2DM. Insulin is mitogenic and antiapoptotic, and the risk of breast cancer rises proportionally with insulin levels, independently of estradiol levels.
The pathways presented likely occur in all tissues that are at higher risk for obesity-related cancer. In the breast, these pathways also contribute to elevated bioavailable estradiol, which further contributes to increased cancer risk, especially in PMP women (Figure 12-3).9 Adipokines, leptin, and insulin induce aromatase expression in the mesenchymal cells surrounding the adipocytes, thereby further increasing the local estrogen concentration in the breast adipose tissue by upregulating the conversion of androstenedione to estrone and testosterone to estradiol. These changes are conducive to the development of cancer that is estrogen receptor (ER) positive. An association of aromatase levels and local breast inflammation, as measured by the number of CLS-B, has been shown.11 Estrogen promotes further cell proliferation, leading to increased expression of inflammatory mediators, thus establishing a positive-feedback loop in the breast microenvironment. This has implications for treatment and resistance to treatment in some women.
Macrophage infiltration in the microenvironment of ductal carcinoma in situ is associated with breast cancers that are high grade, ER negative (ER–) and progesterone receptor (PR) negative (PR–). The association of breast cancer and obesity in premenopausal women is less clear. Some studies supported an increased risk in obese premenopausal women, while others refuted it. A study from the National Surgical Adjuvant Breast and Bowel Project (NASBP) Cancer Prevention Trial showed that, in women over 35 years of age with a baseline elevated risk (Gail score > 1.66), the effect of overweight and obesity was additive compared to women with normal BMI.12
Animal models supported this association. In one study, premenopausal rats with diet-induced obesity had a 26% excess rate of mammary tumors compared to dietary-resistant rats with a higher proportion of ER– tumors, a higher tumor burden, and a reduction in cancer latency.13 There was also an adverse impact of weight gain on circulating levels of several of the factors discussed previously, including leptin, adiponectin-leptin ratio, insulin, and IGF-1.
Premenopausal women are at higher risk to develop triple-negative breast cancers [ER, PR, and human epidermal growth factor receptor 2 (HER2/neu)]. Paradoxically, obesity does seem to be a risk factor for triple-negative breast cancer in premenopausal women. The initial insult may be similar to that in obese PMP women with inflammatory changes and cytokine release. However, the epithelial cells in premenopausal women may undergo transition to mesenchymal cells caused in part by the leptin-induced increase in Wnt expression. Gene expression in these mesenchymal cells favors cell proliferation and invasion and may give rise to the triple-negative phenotype seen in premenopausal women. The details of these pathways and mechanisms remain to be elucidated.
Along with being a risk factor for the development of breast cancer, obesity is a prognostic factor in stage at diagnosis and outcome. And, for most cancers in which obesity is a risk factor, the mortality risk mirrors that for incidence. In the Million Women Study, women with BMI greater than 30 had a 50% higher mortality than women with a normal BMI.14 A similar association was found in the California Breast Cancer Survivorship Consortium.15 In addition, breast tumors in obese women are more likely to be of higher histologic grade and more advanced stage than in women with normal BMI.
Leptin levels are significantly higher in PMP women with more advanced ER+ tumors, and leptin (along with BMI) is an independent predictor of stage in these women.16 Leptin has been shown to activate ER transcription independent of estradiol, suggesting that leptin is involved in stimulation of growth of ER+ breast tumors through both autocrine and paracrine actions, the latter manifested as induction of aromatase in stromal cells of the breast tissue, as well as by influencing the secretion of other modulators of cell growth regulation. High leptin levels may contribute to tumor response to treatment by competing with antiestrogens at the ER and may cause antiestrogen resistance in some patients. Overexpression of leptin in breast cancer tissue may be a major factor in poor treatment response in some women because it promotes a positive-feedback loop for expression of factors essential for continued tumor promotion.
Leptin does not correlate with breast cancer stage in premenopausal women.
Maintenance of a healthy weight should be a primary disease prevention strategy as weight is one of the few modifiable cancer risk factors. Weight loss as a breast cancer prevention strategy in the United States should be promoted on a public health level; up to 17% of breast cancer cases could be prevented by weight loss, as confirmed by studies that have demonstrated a protective effect of weight loss in PMP women.10 Unfortunately, data suggest that the incidence of obesity in the United States and worldwide continues to climb. Many women are not aware of the association between obesity and breast cancer, even women who have high awareness of other health consequences of obesity, such as hypertension and diabetes. This knowledge deficit was particularly high among black and Hispanic women, with close to 50% of these women in 1 study unaware of this association.17
There are multiple medical strategies under study for breast cancer treatment and possible prevention, which take advantage of anti-inflammatory and antiestrogenic properties. One strategy is the use of nonsteroidal anti-inflammatory medications (NSAIDs), such as cyclooxygenase 2 (COX-2) inhibitors. Epidemiological studies indicated that anti-inflammatory drugs reduce the risk of both ER+ and ER– breast cancer. CLS-Bs have been shown to produce excess amounts of COX-2-derived prostaglandin E2, which is known to stimulate aromatase expression and estradiol production in preadipocytes. In vitro studies have shown that obesity-associated serum factors induce aromatase expression by way of induction of macrophage COX-2 expression.18 This also led to increased ER-α activity in breast cancer cell lines. One retrospective study showed a better survival rate and longer disease-free survival in predominantly overweight and obese breast cancer patients who were taking COX-2 inhibitors than those who were not.
Another avenue that may be exploited in the fight to prevent breast cancer is the use of oral hypoglycemic agents such as metformin. Epidemiologic studies have shown an association between metformin use and decreased incidence of breast and other cancers. Metformin has been shown to induce downregulation of ErbB2 (HER2/neu equivalent in humans) expression in a mouse model.19 HER2/neu is a membrane glycoprotein that is overexpressed in about 20%–30% of breast cancers and that has been correlated with poorer prognosis. Overexpression of HER2/neu may be associated with breakdown in the normal cell control mechanisms that would promote cell proliferation and a more aggressive tumor phenotype. Metformin may also decrease local and circulating insulin levels.
A third class of drugs, statins (used to treat hyperlipidemia), has shown potential as chemopreventive agents and has been shown to inhibit cell proliferation and induce apoptosis in in vitro models.20 However, epidemiologic and prospective studies have revealed disappointing results. In the Women’s Health Initiative (WHI), only small, nonsignificant reductions in breast cancer incidence were seen in users of a hydrophilic statin, and a small, statistically significant reduction was seen in users of a hydrophobic.21
Antiestrogens, such as the selective ER modulators (SERMs) tamoxifen and raloxifene, decrease the incidence of ER+ breast cancer in both premenopausal and PMP women by approximately 40%. The US Preventive Services Task Force (USPSTF) recommends increased use of these SERMs for primary prevention of ER+ breast cancer in women at high risk (5-year Gail model score > 1.67).22 Exemestane, an aromatase inhibitor, has been shown to decrease the incidence of ER+ breast cancer in high-risk PMP women by approximately 70%23 and is recommended as a primary prevention strategy by the American Society of Clinical Oncology.24
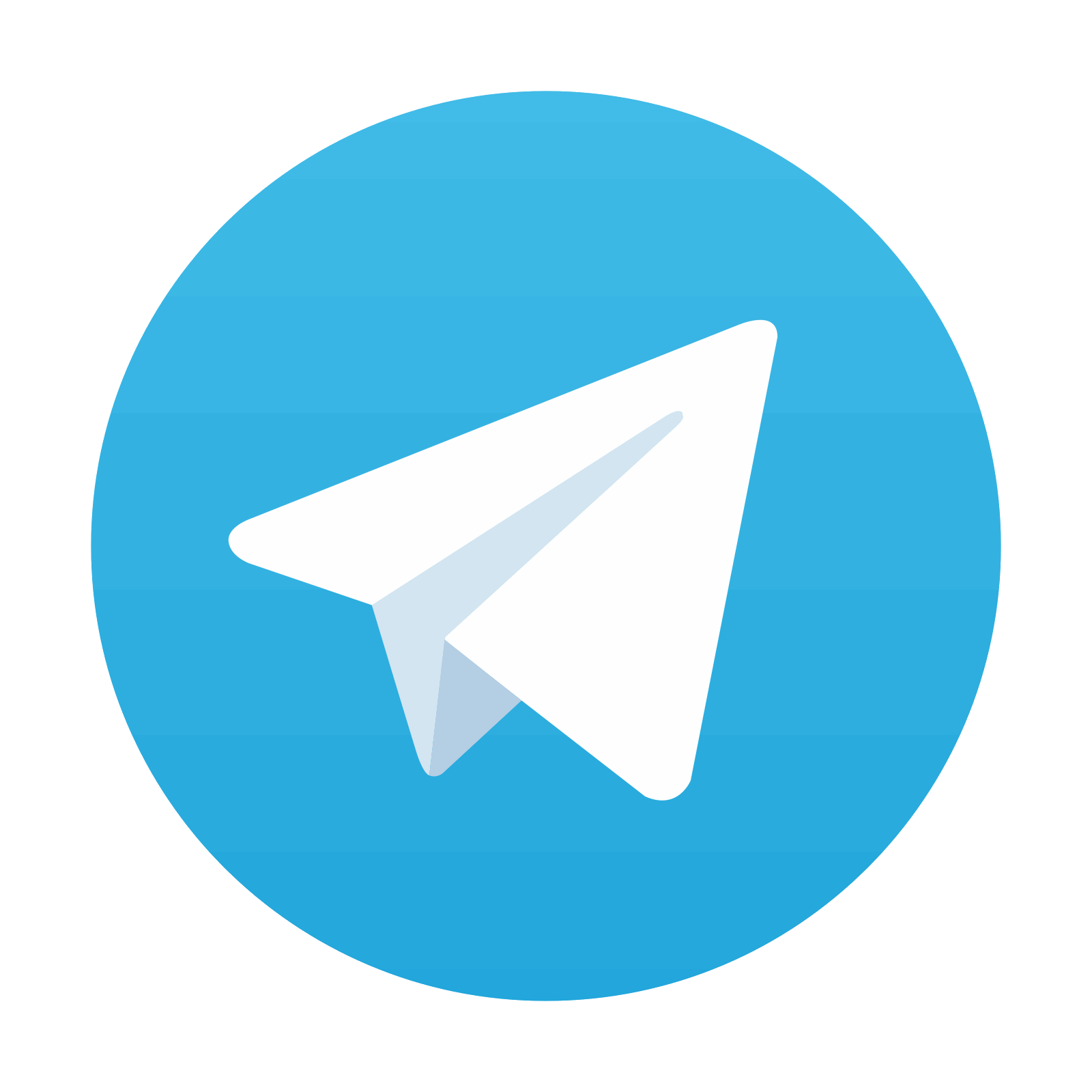
Stay updated, free articles. Join our Telegram channel

Full access? Get Clinical Tree
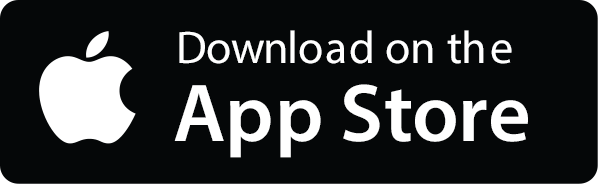
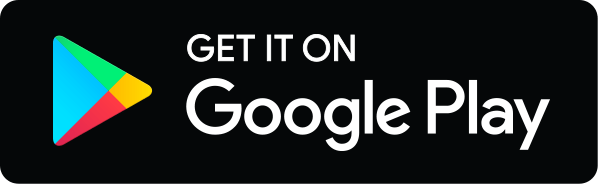