Burns
Over the past several decades, burn-related hospital admissions and deaths have decreased by 50% in large part due to advancements in burn care, introduction of topical antimicrobial agents, and a better understanding of fluid resuscitation and aggressive surgical management.1–3 Despite these advances, nearly 100,000 children age 14 years and under were treated for burns in hospital emergency rooms in 2007. Of these, 20% occurred in children less than 4 years old.4 Thus, burns remain a leading cause of morbidity and mortality in children. Optimal management requires a team of health care providers, therapists, and social workers.
Pathophysiology
In 1953, Jackson described the zones of burn injury that remain important to the understanding and management of burns today (Fig. 13-1).5 The zone of coagulation occurs at the site of maximal damage and is defined by irreversible tissue loss due to protein coagulation. The zone of stasis surrounds this area and has decreased tissue perfusion, but remains salvageable with aggressive resuscitation. Outside the zone of stasis is the zone of hyperemia where tissue perfusion is increased and often survives unless faced with infection or hypotension.
The systemic response to burn injury is mediated by the release of inflammatory mediators such as thromboxane A2, bradyknin, oxidants, and cytokines which can impair flow to the zone of stasis through thrombosis, vasoconstriction, and capillary blockage.6 The administration of antioxidants, bradykinin antagonists, and thromboxane A2 inhibitors can improve blood flow and mitigate injury.7–9 In addition, the administration of β-glucan, through its immunomodulatory effects, antioxidant properties, and ability to reduce the inflammatory response, has been shown to improve re-epithelialization in a rat model of burn injury.10
The systemic effects of burn injury extend beyond these three zones and can potentially lead to multiorgan dysfunction. The cardiovascular system can experience myocardial depression and hypovolemia. Pulmonary vasoconstriction and edema lead to respiratory failure.11 Splanchnic vasoconstriction can result in gut dysmotility and malabsorption by causing epithelial apoptosis and decreased epithelial proliferation.12–14 This results in atrophy of small bowel mucosa, increased intestinal permeability, bacterial translocation, and sepsis.15 Splanchnic vasoconstriction and activation of stress-induced hormones and mediators, such as angiotensin, aldosterone, and vasopressin, leads to a decrease in renal perfusion that can lead to oliguria.16 When unrecognized, this can progress to acute tubular necrosis, renal failure, and, ultimately, death.17,18 Finally, there is a systemic decrease in immune function due to impaired production and function of neutrophils, macrophages and T-lymphocytes, placing the patient at risk for infectious complications.19,20
Initial Management
The majority of pediatric burns are minor, often resulting from scald accidents and affecting less than 10% total body surface area (TBSA), or from thermal injuries isolated to the hands. Such burns are usually limited to partial-thickness injury of the skin and can be managed on an outpatient basis, which is beyond the scope of this chapter. Unfortunately, larger burns require inpatient treatment and special attention. The initial step is completion of the primary and secondary surveys.21 Issues with airway, breathing, and circulation should be addressed immediately. Signs and symptoms including increased respiratory effort, wheezing, stridor, and tachypnea should raise concern for impending loss of the airway. The decision to intubate a patient with a tenuous airway should be made early. Inhalation injury can result in edema that may worsen over the first few hours, so repeated evaluations of the airway are important. Burn injuries can have a negative effect on breathing mechanics through smoke inhalation, a blast injury causing blunt chest trauma, and the restrictive effects of a burn eschar that limits full chest expansion. Escharotomy should be performed for the latter. Patients need support with 100% supplemental oxygen. If inhalation injury is suspected, arterial blood gas analysis and carboxyhemoglobin level are needed. Two large bore IVs should be placed and aggressive fluid resuscitation is important. When extremity burns limit peripheral IV access or if there is difficulty obtaining central venous access, intraosseus access should be utilized as a temporary (<24 hours) alternate route for fluid administration. A urinary catheter should be inserted, and heart rate, blood pressure and urine output should be monitored as tachycardia and low urine output signal a low intravascular volume state.
A decision should be made after initial evaluation, resuscitation, and wound dressing as to whether or not the patient needs to be transferred to a burn center. The American Burn Association and American College of Surgeons have clear recommendation regarding which patients should be referred to these centers (Box 13-1).22 However, a recent study raises concern that resources are often wasted by transferring less severe injuries to burn centers.23
There are several techniques to estimate the TBSA of the burn. The Wallace rule of nines estimates burn area fairly well for adolescents. Each upper extremity and the head represent 9% of the TBSA. The lower extremities and the anterior and posterior trunks are 18% each. The perineum, genitalia, and neck each measures 1%. Due to differences in body proportions for infants and children, the rule of nines has been modified to more accurately determine burn area in these patients. In this modification, the head represents 18% of the TBSA and each leg is 13.5%. Other modifications have been proposed to better estimate TBSA burn in obese patients.24 The Lund and Browder chart may provide more accurate determination of burn area in children as it compensates for variations in body shape and proportions (Fig. 13-2). For a rapid estimation of burn size, the palmar method can be used. The palmar surface is approximately 1% of the TBSA and is best used for estimating small surface area burns. First-degree burns should not be included in burn size calculations using any of these techniques. A recent study found that estimates of burn area are inaccurate in approximately 80% of children with the majority being overestimated.25 The authors concluded that this may be due to the variety of techniques used, or inclusion of first-degree burns in the calculation. This can also lead to unnecessary transfer of patients to burn care centers as discussed previously.
FIGURE 13-2 The Lund–Browder burn diagram is depicted for estimating the body surface area for burns in children.
A key component to the initial management of the severely burned patient includes escharotomy when indicated (Fig. 13-3). Full-thickness circumferential burns on the extremities can produce a constricting eschar which, together with the associated edema, can impede venous outflow and impair arterial flow. If pulses are absent, a bedside escharotomy should be performed with a scalpel or electrocautery along the lateral and medial aspects of the affected extremity. Incisions can be carried onto the hypothenar and thenar eminences and dorsolateral aspects of the digits if the hands or fingers are involved (Fig. 13-4). The resulting ischemia reperfusion injury and edema can cause significant increases in fascial compartment pressures. Although rare, fasciotomy may be necessary to relieve pressure and prevent necrosis of the underlying muscles and neurovascular bundles.
FIGURE 13-3 Escharatomies. The incisions are made on the medial and lateral aspects of the extremity. Hand escharotomies are performed on the medial and lateral digits and on the dorsum of the hand. (From Eichelberger MR [ed]; Pediatric Trauma: Prevention, Acute Care, Rehabilitation. St Louis, Mosby, 1993.)
FIGURE 13-4 A 3-year-old male transferred after being rescued from a house fire. He required extensive escharotomies of the (A,B) chest and (C) distal extremities during the initial resuscitation. (D) Incisions should be carried onto the hypothenar and thenar eminences and dorsolateral aspects of the digits if the hands or fingers are involved.
Fluid Resuscitation
Once adequate intravenous or intraosseous access is obtained, fluid resuscitation is initiated. There are several formulas available to guide this resuscitation (Table 13-1). The Parkland formula is the most widely used, but is not as applicable in young children because children have greater TBSA relative to their body weight than adults. As a result, weight-based formulas can under-resuscitate children with minor burns and can grossly over-resuscitate children with extensive burns.26 TBSA-based formulas, such as the Shriners–Galveston formula, are therefore better at estimating fluid requirements in children less than 20 kg.27 TBSA is assessed from height and weight using standard nomograms or calculated using formulas (Table 13-2). Dextrose-containing solutions, such as 5% dextrose with 0.25 to 0.5 normal saline, are used as the primary solution. Children younger than 2 years of age are susceptible to hypoglycemia due to limited glycogen stores. Therefore, lactated Ringer’s solution with 5% dextrose is given during the first 24 hours in these patients.
TABLE 13-1
Formula | First 24 Hours | Fluid Solution |
Parkland | 4 mL/kg per %TBSA burn | Lactated Ringer’s (LR) |
Brooke | 1.5 mL/kg per %TBSA burn | LR + colloid 0.5 mL/kg per %TBSA burn |
Shriners–Galveston | 5000 mL/m2 burned + 2000 mL/m2 total | LR + 12.5 g albumin |
TABLE 13-2
Formulas for Body Surface Area (BSA)
Dubois Formula | BSA (m2) = height (cm)0.725 × weight (kg)0.425 × 0.007184 |
Jacobson Formula | BSA (m2) = [height (cm) + weight (kg) − 60]/100 |
Regardless of which formula is utilized, there must be close monitoring of the patient’s physiologic response to the fluid administration. Urine output should be maintained at 1 mL/kg/h. Inadequate resuscitation can result in hypoperfusion to the zone of stasis, with subsequent deepening of the burn, as well as hypoperfusion of major organs. During the first six to 12 hours, capillary permeability is increased and fluid moves from the intravascular space to the interstitial tissues with worsening edema. Overly aggressive fluid administration can result in significant tissue edema, tissue hypoxia, and elevated compartment pressures.28
Several authors have shown that the addition of colloid solutions such as albumin can reduce crystalloid requirements and more rapidly establish a balanced fluid intake to output ratio.29,30 However, a 2011 Cochrane database review found that albumin does not lower mortality in adults with major burns.31 Thus, the benefit of the more expensive albumin instead of standard crystalloid solutions is questionable. Other solutions, such as hypertonic saline, have been used in an effort to provide a high osmotic pressure which is thought to keep more volume in the intravascular space. Hypertonic saline also may have anti-inflammatory effects. However, it should be used with caution as it causes hypernatremia, and has not been shown to improve outcomes for hypotensive trauma patients.32
An increased incidence of pneumonia, bloodstream infection, acute respiratory distress syndrome (ARDS), multiple-organ failure, and death may be associated with over-resuscitation during the first 24 hours postburn.33 Interestingly, ‘permissive hypovolemia’ helps avoid these complications and has been shown to decrease multiple-organ dysfunction.34
Inhalation Injury
While inhalation injury is less common in children than adults, the mortality increases to nearly 40% when it occurs.35 The heat of inhaled gas causes upper airway injury. Inhaled toxins can result in upper airway injury in addition to causing tracheobronchial irritation and bronchoconstriction. Damaged epithelium releases vasoactive substances (thromboxanes A2, C3a, and C5a) that lead to hypoxia, increased airway resistance, decreased pulmonary compliance, increased alveolar epithelial permeability, and increased pulmonary vascular resistance.36 Carbon monoxide (CO) is produced during combustion and can contribute to the inhalational injury by displacing oxygen from hemoglobin-binding sites. The oxyhemoglobin dissociation curve is shifted to the left as the ability of hemoglobin to unload oxygen in the tissues is decreased. Secondary injury is due to impaired ciliary clearance of airway debris. Neutrophil infiltration occurs, macrophages are destroyed, and bacteria accumulate leading to pneumonia.
An inhalation injury should be recognized early through a detailed history of the burn and the initial evaluation. On examination, patients may be found to have facial burns, singed hairs in the nose, eyebrows or head, and/or carbonaceous sputum. Patients with inhalation injury who are obtunded are likely to have a carboxyhemoglobin level greater than 10%. Additional signs of significant injury are an altered voice along with hoarseness and stridor. Airway security in these patients is imperative. Fiberoptic bronchoscopy continues to be the preferred technique for documenting inhalation injury. Inflammatory changes in the tracheal mucosa such as edema, hyperemia, mucosal ulceration, and sloughing can be visualized. A ventilation scan with xenon-133 can also identify regions of inhalation injury by assessing respiratory exchange and excretion of xenon-133 from the lungs.37 When combined, bronchoscopy and xenon-133 scanning are over 90% accurate in the diagnosis of inhalation injury. However, the clinical applicability of xenon-133 scans remains unclear due to false positives in children with pulmonary disease.38,39 They may also be potentially dangerous due to the need to transport a critically ill burn patient to a nuclear medicine suite. Chest radiographs are not useful as they are often normal immediately following injury.38,40 Bedside bronchoscopy and a heightened clinical suspicion remain paramount in the diagnosis and treatment of these injuries.
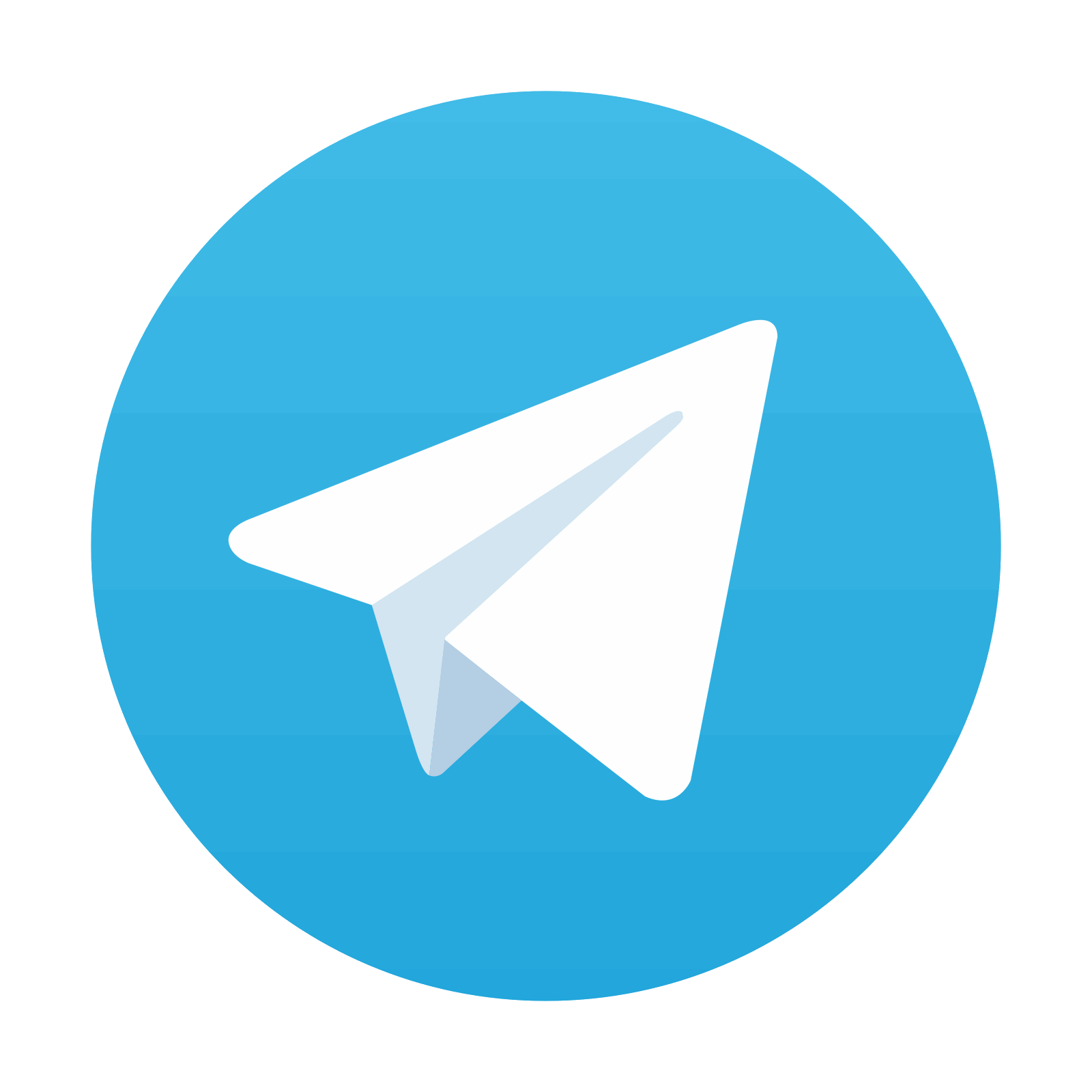
Stay updated, free articles. Join our Telegram channel

Full access? Get Clinical Tree
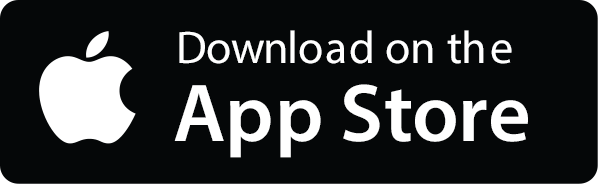
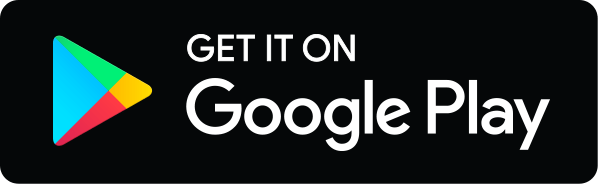