Bronchopulmonary Dysplasia
Jonathan M. Davis
Warren N. Rosenfeld
Bronchopulmonary dysplasia (BPD) is a chronic lung disease that develops in newborn infants treated with oxygen and positive-pressure mechanical ventilation for a primary lung disorder. The introduction of new treatment modalities (e.g., surfactant replacement therapy, high-frequency ventilation, extracorporeal membrane oxygenation, inhaled nitric oxide [INO]) has significantly improved the outcome for many critically ill premature and term infants. As a result, more infants are surviving the newborn period and developing BPD. Approximately 10,000 new cases of BPD occur each year in the United States and there is significant associated morbidity and mortality. There has been significant debate about the exact definition of BPD, further complicated because the specific nature of the disease has changed with the widespread use of surfactant and other therapeutic interventions. Although the current form of BPD appears to be much less severe than in the past, BPD is still an extremely important complication of neonatal intensive care (NICU) and the most common form of chronic lung disease in infants.
The modern history of BPD began with Northway’s observations in 1967 (1). This study documented the clinical course, radiographic findings, and histopathologic lung changes in a group of infants who had received oxygen and ventilatory support for treatment of respiratory distress syndrome (RDS) and established the term BPD. Although Northway originally postulated that oxygen toxicity caused BPD, the exact mechanisms causing the lung injury are complex and are currently the subject of intense investigation. Treatment with positive-pressure ventilation appears to be a critical factor in the development of BPD, although factors such as oxygen toxicity, prematurity, genetic predisposition, infection and inflammation may also play important roles. Therapies for infants with BPD are directed toward improving the pathophysiologic abnormalities after they occur and include oxygen, mechanical ventilation, fluid restriction, and a variety of medications. Many different therapies can be used in these infants, often concurrently. The optimal treatment and prevention strategies have not been definitively established.
This chapter reviews the definition and incidence of BPD (also known as chronic lung disease in newborns), its pathogenesis, pathophysiologic changes, treatment strategies, and long-term outcome. Newly developed approaches for the prevention of BPD in high-risk infants are presented.
DEFINITION AND INCIDENCE
In the original description of BPD, Northway defined chronic lung changes in a group of premature neonates who survived mechanical ventilation for treatment of RDS (1). Northway’s definition of BPD included radiologic, pathologic, and clinical criteria. BPD was divided into four developmental stages. The acute stages, I and II were indistinguishable from RDS and were seen in the first 10 days of life. Stages III and IV marked the transition to the chronic stages of this disease, with stage IV forming the basis for the definition of BPD. This stage described abnormalities that persisted beyond 1 month of age in patients who continued to require respiratory support (i.e., ventilation or oxygen supplementation). Chest radiographs demonstrated cyst formation and hyperexpansion alternating with areas of atelectasis.
As the care of neonates has become more sophisticated and smaller, sicker infants survived, the clinical and radiographic findings that define BPD have changed. Northway’s original criteria depended heavily on a progression of radiographic changes, and clinical criteria were considered secondary. Further refinement was offered by Bancalari, whose criteria included ventilation for at least the first 3 days of life and respiratory symptoms (e.g., tachypnea, auscultatory rales, retractions) at 28 days of life, a need for supplemental oxygen to maintain a partial pressure (PaO2) greater than 50 mm Hg and an abnormal chest radiograph at 28 days of life (2).
TABLE 30-1 DEFINITION OF BRONCHOPULMONARY DYSPLASIA: DIAGNOSTIC CRITERIAa | ||||||||||||||||||||||||
---|---|---|---|---|---|---|---|---|---|---|---|---|---|---|---|---|---|---|---|---|---|---|---|---|
|
Many infants who eventually develop chronic lung disease do not require prolonged mechanical ventilation, nor have significant radiographic abnormalities. Several studies have used less stringent criteria to define BPD such as simply the requirement for oxygen supplementation at 28 days of life (3,4). Shennan and associates questioned the large number of normal neonates who would be included by this criterion and suggested that the need for supplemental oxygen at 36 weeks postconceptual gestational age may be a more accurate predictor of ultimate pulmonary outcome (5). Other investigators have found that the most reliable method to definitively establish the diagnosis of BPD is the presence of chronic respiratory symptoms (e.g., asthma, repeated pulmonary infections) requiring treatment with bronchodilators or corticosteroids in the first year or two of life (6,7). Recently, a consensus conference convened by the National Institutes of Health suggested a new definition of BPD that incorporates many elements of previous definitions and attempts to categorize the severity of BPD (Table 30-1) (7). Ultimately, the definition of BPD will need to be validated with clinically important long-term endpoints in a prospective fashion.
The incidence of BPD depends on the definition used and the patient population studied. Several surfactant replacement trials have reported the incidence of BPD, defined as oxygen dependency at 28 days with appropriate radiographic findings, to be in the range of 11% to 63% and varying significantly by center (8,9,10,11). It appeared from these studies that the incidence of BPD decreased only slightly after surfactant therapy. However, the prevalence, or total number of infants with BPD, increased because of improved survival. Fenton and associates subsequently reported that exogenous surfactant had significantly improved the survival of many low-birth-weight infants in their geographically defined population and was actually associated with a marked increase in the incidence of BPD (12). BPD will continue to be an important problem for the neonatologist and pediatric pulmonologist in the future. Further study of the mechanisms involved in the lung injury process and the development of possible prevention strategies are urgently needed.
PATHOGENESIS
No single factor has been identified as the cause of BPD. Its origin is multifactorial and may depend on the nature of the injury, mechanisms of response, or the infant’s inability to respond appropriately to the injury process (Fig. 30-1). Although Northway attributed the occurrence of BPD primarily to prolonged hyperoxia in infants with RDS, numerous other causes have been proposed.
Barotrauma/Volutrauma
Although the initial phases of lung injury in BPD are the result of the primary disease process (e.g., RDS), superimposed positive-pressure mechanical ventilation appears to
add to the lung injury and provoke a complex inflammatory cascade that ultimately leads to chronic lung disease. Barotrauma is the term generally used to describe the lung injury that occurs secondary to positive-pressure mechanical ventilation, although volutrauma from excessive tidal volume ventilation may be a more appropriate term to describe this lung injury process.
add to the lung injury and provoke a complex inflammatory cascade that ultimately leads to chronic lung disease. Barotrauma is the term generally used to describe the lung injury that occurs secondary to positive-pressure mechanical ventilation, although volutrauma from excessive tidal volume ventilation may be a more appropriate term to describe this lung injury process.
![]() Figure 30-1 An overview of the pathogenesis of BPD showing prenatal and postnatal factors. Adapted from Jobe AH. The new BPD: an arrest of lung development. Pediatr Res 1999;46:641, with permission. |
The role of barotrauma in BPD depends on several factors, including the structure of the tracheobronchial tree and the physiologic effects of surfactant deficiency. With surfactant deficiency, surface tension forces are elevated, aeration is unequal, and most terminal alveoli are largely collapsed. The pressure needed to distend these poorly compliant saccules is high and is transmitted to the terminal bronchioles and alveolar ducts. In the premature infant, these airways are highly compliant and subject to rupture. Gas then dissects into the interstitium and pleural space, resulting in the development of pulmonary interstitial emphysema (PIE) and pneumothorax. These complications are strongly associated with the development of BPD, suggesting that ventilator-induced lung injury is important in the pathogenesis (13,14).
Nilsson and colleagues have shown that even brief periods of positive-pressure ventilation cause bronchiolar epithelial damage in the lung, with the severity of the injury correlating with the amount of peak pressure used (15). Even with normal tidal volumes, ventilation of the immature or injured newborn lung results in nonuniform inflation and relative overdistension of ventilated segments, especially in the presence of low functional residual capacity (FRC) as a result of surfactant deficiency. Stretching of capillary endothelium and distal lung epithelium increases permeability to serum proteins that may further inhibit surfactant function, creating a vicious cycle that promotes lung injury.
The adverse effects of tidal volume breaths in lungs with low FRC can be ameliorated with better lung recruitment by application of higher positive end-expiratory pressure (PEEP). Experimental studies have shown that overdistension of the lung (not increased pressure) is responsible for lung injury in the surfactant-deficient lung (14). For example, strapping the chest wall to restrict overexpansion increases lung pressure but limits lung stretch, and ameliorates lung injury in animal models (16). Tremblay and associates have observed that mechanical ventilation with high tidal volumes and low PEEP markedly increases edema and cytokine production in the lung of rats (17). In this same model, lung injury increased cytokine release from the lungs into the systemic circulation, suggesting a potential mechanism linking multi-organ dysfunction and sepsis syndrome with lung injury. Improved survival of adults with acute respiratory distress syndrome (ARDS) has been found when employing a ventilator strategy that used low tidal volumes with high PEEP to recruit atelectatic lung regions and improve FRC (18).
Strategies to prevent lung injury have included changes in methods of ventilation. Even brief exposure to large tidal volume breaths during resuscitation shortly after birth can initiate early lung injury, which decreases the subsequent response to surfactant therapy (19). With the increased use of nasal continuous positive airway pressure (NCPAP), many preterm infants may be able to be managed without the need for endotracheal intubation and mechanical ventilation, which may lessen the risk for lung injury. Synchronized intermittent mechanical ventilation (SIMV) and high-frequency devices have also been extensively studied. Bernstein and associates reported that infants (<1,000 g) treated with SIMV developed less BPD than those receiving conventional ventilation (20). Several multicenter trials have demonstrated that high-frequency oscillatory ventilation (HFOV) or high frequency jet ventilation (HFJV) using high volume, alveolar recruitment strategies in conjunction with surfactant replacement therapy reduced complications of mechanical ventilation (PIE, pneumothorax) and lowered the incidence of BPD compared to conventional ventilation (21,22). However, these findings have not been consistently found in other trials. Regardless of the type of ventilation strategy used, it is important to avoid even brief periods of overdistension, because high tidal volumes and hypocarbia are associated with a greater risk for developing both BPD and neurologic injury (23,24). A retrospective study of 1,105 newborns born with birth weights about 2,000 g between 1984 to 1987 demonstrated a strong relationship between prolonged hypocapnia (PaCO2 <35 torr) and the development of cerebral palsy (CP) (24).
The use of surfactant replacement therapy has reduced some complications of mechanical ventilation. Surfactant permits more equal distribution of pressures and ventilation to all alveoli, prevents overdistension of distal air spaces and bronchioles, and stabilizes lung volume. A major benefit of surfactant therapy has been the ability to reduce airway pressures and the incidence of air leak, while maintaining a greater uniformity of lung recruitment. However, BPD continues to be an important problem despite the widespread use of exogenous surfactant and strategies to minimize lung injury from mechanical ventilation (e.g., permissive hypercapnia). Van Marter and associates recently studied 452 premature infants weighing 500 to 1,500 g at birth who were born at specific neonatal centers in either Boston or New York. They found that the incidence of BPD was significantly higher in Boston (22%) compared to New York (4%), even after adjusting for baseline risk factors, such as severity of prematurity (25). Using multivariate analyses to examine differences in specific respiratory care practices during the first week of life, most of the increased risk of BPD was associated with the early initiation of mechanical ventilation. Interestingly, the use of both exogenous surfactant and indomethacin increased the risk for BPD. This suggests that attempts to minimize the use of mechanical ventilation using nasal continuous positive airway pressure (NCPAP) (with or without exogenous surfactant) may actually lower the incidence and severity of BPD in high-risk infants. Ongoing multicenter trials of early NCPAP in premature infants are currently underway. BPD continues to be a serious problem, suggesting that
barotrauma is only one of many factors involved in the pathogenesis of BPD.
barotrauma is only one of many factors involved in the pathogenesis of BPD.
Oxygen and Antioxidants
Under normal conditions, a delicate balance exists between the production of oxygen free radicals (also called reactive oxygen species) and the antioxidant defenses that protect cells in vivo. Free radicals are molecules with extra electrons in their outer ring and they are toxic to living tissues (Table 30-2). Oxygen has a unique molecular structure and is abundant within cells. It readily accepts free electrons generated by oxidative metabolism within the cell, producing free radicals. Increased free-radical production can occur under conditions of hyperoxia, reperfusion, or inflammation. Alternatively, free radicals can increase because of an inability to quench production because of inadequate antioxidant defenses. Damage caused by oxygen free radicals includes lipid peroxidation, mitochondrial injury, protein nitration and unraveling of nucleic acids.
The premature neonate may be more susceptible to free-radical damage because adequate concentrations of antioxidants may be absent at birth. Frank and associates documented the development of the antioxidant enzymes superoxide dismutase (SOD), catalase, and glutathione peroxidase in the lungs of rabbits during late gestation (Fig. 30-2) (26). The 150% increase in these enzymes during the last 15% of gestation parallels the maturation pattern of pulmonary surfactant. These developmental changes in the fetal lung allow proper ventilation by reducing surface tension and provide for the transition from the relative hypoxia of intrauterine development to the oxygen-rich extrauterine environment. Premature birth can occur before the normal upregulation of these antioxidant systems and other free radical scavengers (e.g. vitamin E, ascorbic acid, glutathione, and ceruloplasmin) and may result in an imbalance between oxidants and antioxidants and an increased risk for the development of BPD. Experimental animal models have shown that exposure to chronic hyperoxia can induce inflammation and lung injury that has many features seen in infants developing BPD. Both epithelial and endothelial cells are extremely susceptible to oxidant injury, leading to increased permeability edema and cell dysfunction. Hyperoxia also impairs mucociliary function, promotes inflammation and inactivates antiproteases, further complicating clinical outcome.
TABLE 30-2 FREE RADICALS | ||||||||||||||||||||||||
---|---|---|---|---|---|---|---|---|---|---|---|---|---|---|---|---|---|---|---|---|---|---|---|---|
|
Clinical studies suggest that free radicals are involved in the pathogenesis of BPD. Plasma concentrations of allantoin, an oxidation byproduct of uric acid, has been shown to be significantly elevated in the first 48 hours of life in infants developing BPD compared to controls (27). Expired pentane and ethane have also been measured as indirect evidence of free radical-induced lipid peroxidation in the first week of life and found to be significantly elevated in neonates subsequently developing BPD (28). Varsila and colleagues analyzed proteins in tracheal aspirates in the first week of life and found evidence of protein oxidation (carbonyl formation) in infants developing BPD (29). Banks and associates found a fourfold increase in plasma 3-nitrotyrosine concentration in infants developing BPD compared to controls, indicating increased evidence of reactive nitrogen species (endogenous nitric oxide [NO] reacting with superoxide) modifying serum proteins (30). Of note, the multicenter STOP-retinopathy of prematurity (ROP) trial examined whether exposing premature infants to higher inspired oxygen concentrations would prevent the development of severe ROP. Although the effects of the increased oxygen were minimal on the eyes, exposed infants had a marked increase (55%) in the incidence of BPD and pulmonary infections (31).
Further evidence for the role of oxygen free radicals in lung injury comes from animal studies, which have shown that antioxidant supplementation with SOD and catalase reduces cell damage, increases survival, and prevents lung
injury from prolonged hyperoxia and mechanical ventilation (32,33). Genetically engineered mice overexpressing SOD survive longer, whereas mice with disrupted SOD genes die more quickly in a hyperoxic environment compared to normal diploid controls (34,35). These studies all demonstrate that oxygen free radicals are intimately involved in the development of acute lung and chronic lung disease in newborn infants.
injury from prolonged hyperoxia and mechanical ventilation (32,33). Genetically engineered mice overexpressing SOD survive longer, whereas mice with disrupted SOD genes die more quickly in a hyperoxic environment compared to normal diploid controls (34,35). These studies all demonstrate that oxygen free radicals are intimately involved in the development of acute lung and chronic lung disease in newborn infants.
Inflammation
Marked inflammation in the lung appears to begin a cascade of destruction and abnormal repair that develops into BPD. The initial stimuli activating the inflammatory process in the lung may be oxygen free radicals, pulmonary barotrauma, infectious agents, or other stimuli that result in the attraction and activation of leukocytes. Inflammation appears to play an important role in the pathogenesis of BPD and allows many factors to be unified into a single cause of this disease. Inflammatory mediators and cellular responses have been found to be prominent in animal models of lung injury and in infants who develop BPD.
A sustained increase in the number of neutrophils that generate excessive oxygen free radicals in tracheal fluid samples distinguishes infants who develop BPD from those who recover spontaneously (36,37). Additionally, the presence of activated macrophages, high concentrations of lipid products, inactivated α-1-antitrypsin activity, and other markers of active inflammation are strongly linked with the development of BPD (38). Release of early response cytokines, such as tumor necrosis factor-α (TNF-α), interleukin-1β (IL-1β), interleukin-8 (IL-8), and transforming growth factor (TGF)-β by macrophages and the presence of soluble adhesion molecules (e.g., selectins) may impact other cells to release chemoattractants and recruit neutrophils which further amplify the inflammatory response (38,39,40,41,42). Elevated concentrations of pro-inflammatory cytokines (IL-6, IL-8) in conjunction with reduced antiinflammatory products, (IL-10) usually appear in tracheal aspirates within a few hours of life in infants subsequently developing BPD (40,41,42). All these agents recruit and activate leukocytes resulting in significant pulmonary damage, including breakdown of capillary endothelial integrity and leakage of macromolecules (e.g., albumin) into alveolar spaces. Albumin leakage and pulmonary edema are known to inhibit surfactant function and have been postulated to be important factors in the development of BPD (43).
The release of elastase and collagenase from activated neutrophils directly destroys the elastin and collagen framework of the lung. The breakdown products of collagen (hydroxyproline) and elastin (desmosine) have been recovered in the urine of infants who develop BPD (44). The major defense against the action of elastase activity is α1-proteinase inhibitor, which may be inactivated by oxygen radicals. Increased elastase activity accompanied by compromised antiproteinase function may enhance lung injury. This combination has been demonstrated in tracheal aspirates and serum of neonates who develop BPD (45,46,47).
As the acute cycle of injury continues with further production and accumulation of inflammatory mediators, significant injury to the lung can occur during a particularly critical period of rapid growth; six divisions from 24 to 40 weeks of gestation. It appears likely that this abnormal inflammatory process is primarily responsible for the acute and the chronic changes that occur in the lungs of infants with BPD. Watterberg and associates have demonstrated that extremely premature infants have evidence of adrenal insufficiency at birth with the lowest serum cortisol concentrations during the first week of life correlating with increased lung inflammation and adverse respiratory outcome (48). Pilot studies examining early treatment with low-dose hydrocortisone in extremely low birth weight (LBW) infants increased the likelihood of survival without BPD, a benefit that was particularly apparent in infants born to mothers with chorioamnionitis (49). The mechanisms of action may include suppression of lung inflammation with less inhibition of lung growth observed with higher doses of steroids or even beneficial effects of low dose hydrocortisone on lung development. These studies all clearly support the notion that this abnormal inflammatory process is primarily responsible for the acute and the chronic changes that occur in the lungs of infants with BPD.
Infection
Subclinical intrauterine infection and the ensuing inflammatory response have been clearly implicated in the etiology of preterm labor and premature rupture of membranes (50). Growing evidence indicates that prenatal infection and inflammation are major risk factors for the subsequent development of BPD. Although several investigators have found a lower incidence of RDS in preterm infants born to mothers with chorioamnionitis and funisitis (possibly as a result of an adaptive response to in utero stress), they also observed a significantly higher incidence of BPD in these same infants (51). This suggests that although intrauterine infection may accelerate lung maturation, the ensuing inflammatory response may also “prime the lung,” causing lung injury, progressive inflammation and subsequent inhibition of lung growth. Subsequent exposure to hyperoxia and mechanical ventilation may further exacerbate the injury process in an already infected and/or inflamed lung.
Ureaplasma urealyticum has been recovered from cervical cultures of pregnant women and implicated as a possible cause of chorioamnionitis, prematurity, and BPD (52). Cassell and colleagues cultured tracheal aspirates and blood and found that BPD developed in 82% of infants (<1,000 g) colonized with Ureaplasma, compared with 41% of those with negative cultures (52). In contrast, other studies have found that although Ureaplasma was frequently detected (by culture or polymerase chain reaction) in many LBW infants, its presence was not associated
with the development of BPD (53,54). It is possible that infection simply acts as a stimulus for the inflammatory response, with recruitment of leukocytes and the production of cytokines and oxygen free radicals, ultimately leading to the development of BPD. Normal defense mechanisms against infection can be compromised in the lungs of these chronically ventilated, premature infants. This makes them more susceptible to colonization and subsequent infection with a variety of infectious agents (e.g., virus, bacteria, fungi) that may affect the severity of BPD (55). Several large clinical studies have found a strong correlation between the presence of BPD and the development of late-onset sepsis, usually with organisms such as staphylococcus epidermidis (56,57). These infections are associated with increased morbidity, mortality, and length of hospital stay and appear to contribute to the severity of BPD.
with the development of BPD (53,54). It is possible that infection simply acts as a stimulus for the inflammatory response, with recruitment of leukocytes and the production of cytokines and oxygen free radicals, ultimately leading to the development of BPD. Normal defense mechanisms against infection can be compromised in the lungs of these chronically ventilated, premature infants. This makes them more susceptible to colonization and subsequent infection with a variety of infectious agents (e.g., virus, bacteria, fungi) that may affect the severity of BPD (55). Several large clinical studies have found a strong correlation between the presence of BPD and the development of late-onset sepsis, usually with organisms such as staphylococcus epidermidis (56,57). These infections are associated with increased morbidity, mortality, and length of hospital stay and appear to contribute to the severity of BPD.
Nutrition
The nutritional status of the critically ill premature infant may also be important in the development of BPD. Adequate calories and essential nutrients for growth may be lacking during a period of stress and growth; vital components for immunologic and antioxidant defenses may be inadequate; and the nutritional supplements provided may actually contribute to ongoing damage.
Premature infants have increased nutritional requirements because of increased metabolic needs and rapid growth requirements. If these increased energy needs are not met, the infant will develop a catabolic state, which most likely contributes to the pathogenesis of BPD. Inadequate nutrition could interfere with normal growth and maturation of the lung and may potentiate the deleterious effects of oxygen and barotrauma. Newborn rats with inadequate caloric intake have decreased lung weights, protein levels, and DNA content (58). These abnormalities were even greater in pups that were nutritionally deprived at birth and exposed to hyperoxia.
Antioxidant enzymes may play a vital role in the protection of the lung and the prevention of BPD. Many of these enzymes have trace elements (e.g., copper, zinc, selenium) that are an integral part of their structure. Deficiencies in these elements may compromise the premature infant’s defenses and predispose the lung to further injury. The repair of elastin and collagen is limited in animals that are undernourished, and copper and zinc may be necessary for this repair (59). Although supplementation with these elements may provide protection to the lung and prevent hyperoxic lung injury, clinical trials using limited dosing strategies have not been able to demonstrate a beneficial effect in preventing BPD (60). Vitamin deficiency has been postulated to be important in the development of BPD. Although current nursery feeding and hyperalimentation regimens appear to provide adequate amounts of vitamin E for preterm infants, a relative decrease in the concentrations of other vitamins may be important in the pathogenesis of BPD. For example, Vyas and colleagues reported significant decreases in levels of ascorbate (which can function as an antioxidant) in tracheal aspirate fluid from infants developing BPD compared to control infants, suggesting that supplementation with vitamin C might be beneficial (61). However, early supplementation with high dose “antioxidant” vitamins has been studied in preterm baboons and was not efficacious in preventing lung damage from prolonged hyperoxia (62).
Concentrations of vitamin A (retinol) may also be deficient in very LBW infants (63,64,65). This vitamin appears to be important in maintaining cell integrity and in tissue repair. Its deficiency has been associated with changes in the ciliated epithelium of the tracheobronchial tree (66). Hustead and associates demonstrated lower serum retinol levels in cord blood and at day 21 of life in infants who developed BPD (65). Similarly, Shenai and colleagues demonstrated lower plasma retinol concentrations in the first month of life in infants who subsequently developed BPD (67). Despite adequate supplementation, some infants remain vitamin A deficient, presumably from increased absorption of parenteral vitamin A into the tubing of the intravenous administration set or from higher nutritional requirements (68). A multicenter trial of vitamin A supplementation in premature infants at risk for developing BPD recently demonstrated that large doses of intramuscular vitamin A given three times per week was associated with a small (7%), but significant reduction in the incidence of BPD, suggesting that vitamin A deficiency is an important contributor to lung injury (69).
Large volumes of intravenous fluids are often administered to premature infants to provide adequate fluid requirements (from increased insensible water losses) and sufficient calories. Excessive fluid administration can be associated with the development of a patent ductus arteriosus and pulmonary edema, which can lead to an increase in oxygen and ventilator requirements and the subsequent risk of BPD (55,70). Early closure of the ductus, using indomethacin or surgical ligation, has been associated with improvements in pulmonary function, but these approaches have not affected the incidence of BPD (71).
Genetics
Numerous investigators have observed that neonates were more likely to develop BPD if there was a strong family history of atopy and asthma. Nickerson and Taussig found a positive family history of asthma in 77% of infants with RDS who subsequently developed BPD, compared with only 33% who did not (72). Bertrand evaluated the relationship of prematurity, RDS, and need for mechanical ventilation to a family history of airway hyperactivity (73). The severity of lung disease was directly related to the degree of prematurity and the duration of oxygen exposure. However, siblings and mothers of infants with the most significant lung disease had evidence of airway reactivity, suggesting that all three factors are involved in
determining long-term outcome. When histocompatibility loci (HLA) were examined, Clark and associates found that only infants with HLA-A2 developed BPD, again suggesting that other underlying factors that are poorly understood may be important in the pathogenesis of BPD (74). More recently, Hagan reported that a family history of asthma is associated with an increase in the overall severity of BPD in premature infants but does not appear to be a causative factor (75).
determining long-term outcome. When histocompatibility loci (HLA) were examined, Clark and associates found that only infants with HLA-A2 developed BPD, again suggesting that other underlying factors that are poorly understood may be important in the pathogenesis of BPD (74). More recently, Hagan reported that a family history of asthma is associated with an increase in the overall severity of BPD in premature infants but does not appear to be a causative factor (75).
PATHOPHYSIOLOGIC CHANGES
Infants with BPD demonstrate abnormal findings on clinical examination, chest radiograph, pulmonary function testing, echocardiogram, and morphologic examination of the lung. The severity of BPD is directly proportional to the degree of the pathophysiologic insult and can be assessed through all of these techniques. Determining the severity of BPD is complex and has been the subject of several workshops sponsored by the National Institutes of Health and many publications. Several scoring systems have been developed to address this important issue.
Clinical Assessment
A clinical scoring system to help evaluate the severity of BPD was developed by Toce and colleagues (76). Infants with BPD are tachypneic and may have intercostal and subcostal retractions. Accessory muscles may be used to assist with respiration. Infants can be hypoxic and hypercarbic and may grow poorly despite adequate caloric intake. The Toce system attempts to standardize clinical assessment, and a severity score can be assigned to each infant at 28 days of postnatal age and at 36 weeks postconceptual age. The clinical assessment should be adjusted if infants are receiving multiple medications for their BPD (e.g., diuretics, methylxanthines), NCPAP, or positive-pressure mechanical ventilation.
Radiographic Abnormalities
Northway and associates first described radiographic abnormalities characteristic of BPD in 1967 (1). A staging system was employed that documented the progression of the disease process through four distinct stages. The first stage was similar to uncomplicated RDS and occurred in the first few days of life. The BPD often progressed to pulmonary parenchymal opacities (stage II), a bubbly appearance (stage III), and then to an inhomogeneous appearance with marked hyperinflation, bleb formation, irregular fibrous streaks, and cardiomegaly (stage IV).
The radiographic progression of BPD is now seldom categorized by these four stages, so Edwards and colleagues redefined the radiologic changes (77). Their system is based on the four most prominent radiographic findings in BPD, including lung expansion, emphysema (including bleb formation), interstitial densities, and cardiovascular (CV) abnormalities (Table 30-3). More severe changes are associated with higher scores (maximum of 10). The occurrence of hyperinflation or interstitial abnormalities on chest radiograph appears to correlate with the development of airway obstruction later in life (78). Because the severity of BPD has continued to change so significantly over the past decade, Weinstein developed a new scoring system incorporating some of the more subtle radiographic signs that are often seen today in infants with BPD (Fig. 30-3) (79). The utility of these scoring systems remains to be demonstrated.
Computed tomography (CT) and magnetic resonance imaging (MRI) of the lung may provide more details of the structural disease in BPD, and can reveal significant abnormalities that are not readily apparent on chest radiographs (Fig. 30-4) (80). These findings can be important in determining ultimate pulmonary morbidity. CT scans often show regional heterogeneity, with regions of hyperinflation or “emphysema” and sparse arterial density alternating with relatively normal appearing regions. MRI of ventilated premature newborns may demonstrate striking regional variations in lung disease, with marked gravity-dependent
atelectasis and edema. More studies of the role of CT and MRI in BPD are needed to correlate structural and functional changes.
atelectasis and edema. More studies of the role of CT and MRI in BPD are needed to correlate structural and functional changes.
TABLE 30-3 ROENTGENOGRAPHIC SCORING SYSTEM FOR SEVERITY OF BRONCHOPULMONARY DYSPLASIAb | |||||||||||||||||||||||||||||||||||
---|---|---|---|---|---|---|---|---|---|---|---|---|---|---|---|---|---|---|---|---|---|---|---|---|---|---|---|---|---|---|---|---|---|---|---|
|
Cardiovascular Changes
In addition to adverse effects on airways and alveoli, acute lung injury also impairs growth, structure and function of the developing pulmonary circulation after premature birth (81). Endothelial cells have been shown to be particularly susceptible to oxidant injury and the media of small pulmonary arteries may also undergo smooth muscle cell proliferation (82). Structural changes in the lung vasculature contribute to high pulmonary vascular resistance (PVR) as a result of narrowing of the vessel diameter and decreased angiogenesis. In addition to these structural changes, the pulmonary circulation is further characterized by abnormal vasoreactivity, which also increases PVR (83). Overall, injury to the pulmonary circulation can lead to the development of pulmonary hypertension and cor pulmonale, which contributes significantly to the morbidity and mortality of severe BPD (84). Echocardiographic assessment is an extremely valuable tool in confirming these diagnoses.
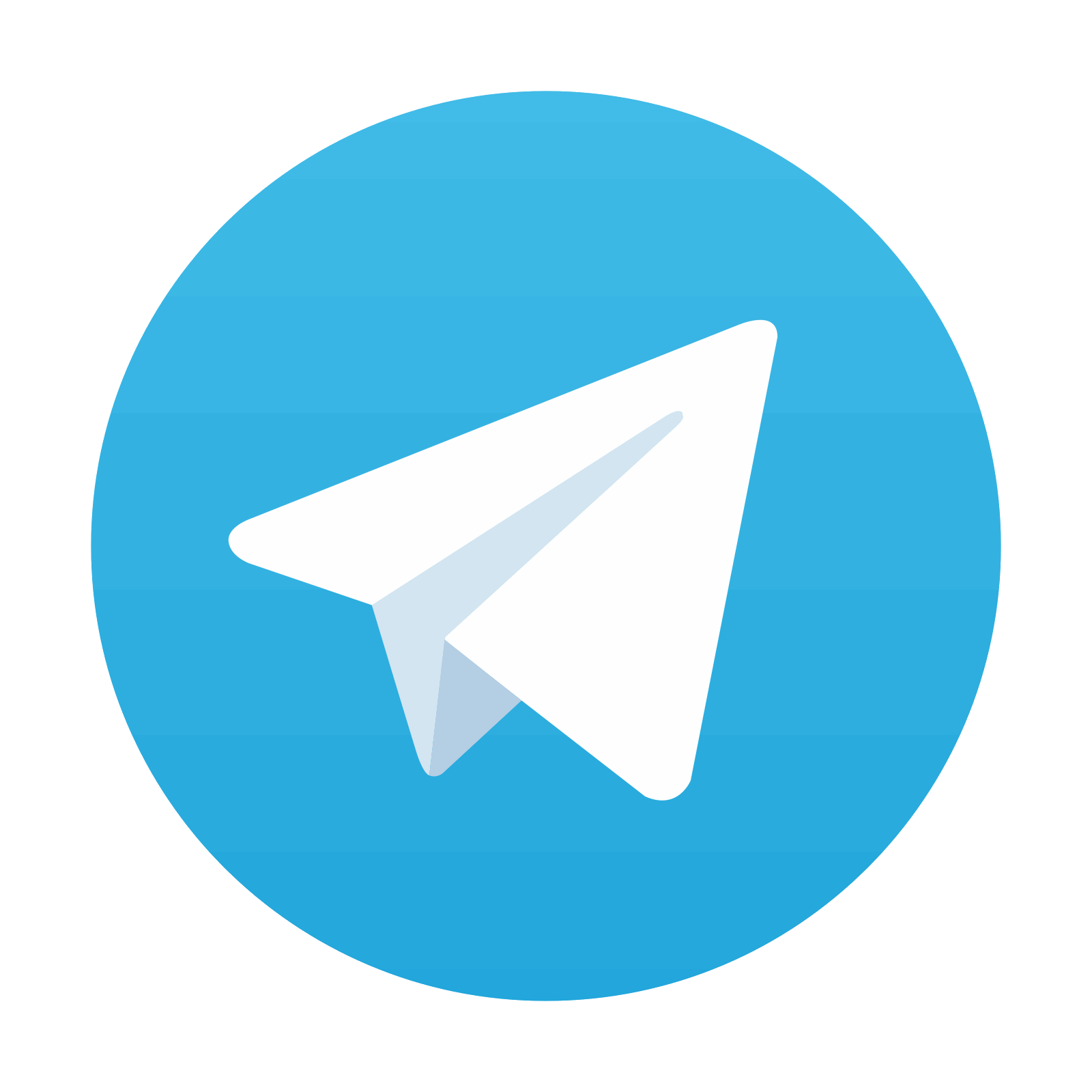
Stay updated, free articles. Join our Telegram channel

Full access? Get Clinical Tree
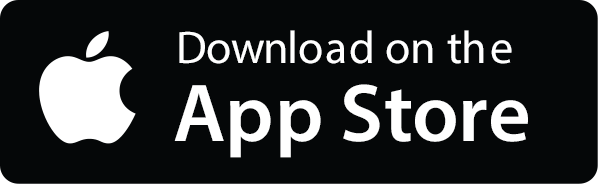
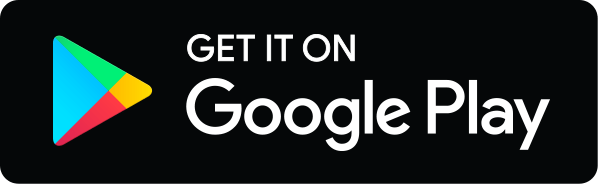