Because of its accessibility, the importance of its pathology, and the rapid availability of pathological confirmation, the breast has long served as a kind of test bed for innovations in ultrasonography. Many of these innovations have translated into mainstream applications, while others remain under development or are merely speculative.
Whole breast ultrasonography is an example of one technical innovation that is increasingly being adopted in the clinic, partly driven by the increasing use of ultrasound as a screening method for the early detection of breast cancer. In fact, whole breast ultrasound has a long history, dating back to the immersion technique of the Octason that was developed at the Commonwealth Scientific and Industrial Research Organisation (CSIRO) in Sydney, Australia.1 In this method, an array of single-element transducers (actually 8, hence the name) was mounted in a large water bath. The patient lay prone with one breast immersed, and the transducers were swept through an angle to image the tissue. It incorporated several advances, such as compounding, and allowed pulsed (spectral) Doppler, but it was expensive (owing to the complexity of designing underwater electrical apparatus) and cumbersome. Modern systems use a linear array, and the patient is scanned supine with a membrane covering the breast. The transducer is driven by a motor that sweeps it across part of the breast. The assembly is repositioned to cover the whole breast, and the data is processed into a single set for subsequent display on a workstation. Since this is a 3D data set, the images can be reformatted into nonacquired planes such as the coronal plane. The main advantage of this type of system is that scanning does not require the same skill as conventional breast scanning, so it can be delegated to a less highly trained technician and read subsequently by a radiologist. This advantage makes the workflow similar to that of mammography or MR and is favored in some heath care systems.
It would appear that ABUS has overcome the problem of operator dependency and gives reproducible, standardized imaging.2 However, recent advances in this technology and image quality have increased interest in the role that ABUS may play in the management of breast lesions. One of the first commercially available systems was developed by Siemens Healthcare and termed the Automated Breast Volume Scanner (ABVS) (Figure 44-1).3
The feasibility and image quality of ABVS are reasonable and comparable to handheld ultrasound, so it has also been proposed as a suitable screening tool for breast cancer (Figure 44-2).4-6 A recent large-scale Japanese study that randomized a total of 72,998 women to either mammography only or ultrasound and mammography for breast cancer screening over a 2-year period showed that there was a 20% increase in pickup detection rate for breast cancers when both were used together.7
Recently in the United States, the somo-v ABUS system (GE medical systems) received FDA 510(k) approval for breast cancer screening as an adjunct to mammography for asymptomatic women with dense breast tissue. One limiting factor for the use of ABUS as a screening tool is the potential high recall rate for patients for second-look handheld ultrasound; however, this will probably diminish with advancing technology and ever improving image quality.8
There are other potential areas where this technology may prove useful. If volumetric measurements are an available tool with this system, this could be useful for measuring tumor volume and assessing response to neo-adjuvant therapy. In addition, it could also be useful for assessing the integrity of breast implants and providing the surgeon a 3D view of the breast prior to surgery (Figure 44-3).
In one implementation (Hitachi), the original prone position is adopted, and the transducer rotates around the center of the breast, which is compressed against a slippery membrane. This has the advantage of scanning radially, which respects the breast’s lobar anatomy and replicates the handheld radial scanning that is favored by many.
There are disadvantages of ABUS when compared with handheld ultrasound, particularly the difficulty in imaging the axillary tail, the lack of Doppler or elastography, and the fact that a conventional scan must be used for biopsies, which means that it fits less well into the one-stop clinic model.
The advent of plane wave ultrasound (“AngioPLUS,” Supersonic Imagine) and new Doppler algorithm and filters (“Superb Microvascular Imaging, SMI” Toshiba Medical Systems; B-Flow, GE Medical Systems) have improved the visualization of the small, slower flowing vessels within the vascular bed. Some studies have suggested that the vascularity of lesions may help in distinguishing benign from malignant lesions, and a recent study suggested that the ability to detect flow using SMI in apparent “avascular” lesions graded by conventional color or power Doppler may be clinically useful in the B1-RADs classification of lesions. However, in many centers, most solid-appearing lesions are subjected to needle sampling irrespective of the presence of vascular flow. An application where these new Doppler technologies may be advantageous is in the monitoring of tumor response to treatment where the neoangiogenic tumor vessels are more clearly depicted when compared to conventional Doppler (Figure 44-4). Quantitative methods would be necessary for it to be of clinical value, and these are in development.
Elastography was first developed for the breast; its use is based on the premise that cancers are stiff while benign lesions are soft. While this is not entirely true as it is the surrounding fibrotic reaction of a breast cancer which is stiff and occasionally the centre of the breast cancer may be soft, it holds up well enough for the addition of elastography to conventional ultrasound to improve accuracy and has been widely adopted and incorporated into guidelines such as the 2013 Bl-RADS Lexicon.9 The basic principles are straightforward, though this cannot be said of the mechanical properties of tissue, which are exceedingly complex and not fully understood. One important property is the fact that compression increases the stiffness of tissue10; it is very important to avoid precompression of the breast by probe pressure. Even mild precompression can make a soft lesion such as a fibroadenoma appear as stiff as a cancer. For all breast elastography, a light touch with plenty of gel is essential.
Two forms of ultrasound elastography are widely used: strain and shear wave.11,12 In strain elastography, the tissue is deformed by probe pressure, and the extent to which the tissue distorts is measured. Stiff tissue distorts very little while soft tissue distorts much more readily. The distortion is measured either using a Doppler method or by tracking the displacement of the speckle that forms the images and is performed on raw or RF data. The method requires some skill and thus a training period, but once mastered, it adds only a few seconds to the conventional scan. Strain elastography is displayed as a color-coded overlay on the B-mode image, and conventionally blue codes are used for stiff tissue (less distortion) while red is used for soft tissue.13
It is important to appreciate that strain elastography displays the relative stiffness of the components of the scan, and thus a large region of interest should be used so that there is normal tissue around the lesion being studied. Although not intrinsically quantitative, the ratio between the stiffness of the surrounding fat or glandular tissue and the lesion can be measured; the strain ratio tends to be higher for cancers than for benign lesions (Figure 44-5).14 In addition, a useful observation is that the strain image of cancers appears larger than the B-mode equivalent, perhaps because the infiltrating margin of a cancer tends to stiffen the normal-appearing margin of the tumor. This size ratio is easily appreciated on the combined display, and suspicion should be raised when a lesion seems to be larger on the elastogram than on B-mode.
Figure 44-5.
Strain elastogram of an infiltrating ductal carcinoma showing full blue coloration indicating a stiff lesion. Two measurement ROIs have been placed, one in the lesion and one in the surrounding tissue. The system calculates the ratio, here a high 13.25, which is suggestive of a malignancy.

Cysts produce characteristic artifacts on strain elastography. Their pattern seems to depend on the type of scanner used; many show a striped pattern of red, green, and blue colors, while in others a bull’s eye pattern in grayscale is shown. Though this seems to be some form of artifact, it is a useful identifier of a cyst that is atypical on B-mode.
Shear wave elastography uses a different approach, and an understanding of the physics is helpful. These are transverse waves, ie, the particle motion is at right angles to the direction of travel, exactly as for the ripples on a water surface. Thus, they are quite different from conventional ultrasound waves and, in addition, they travel much more slowly (at 1-10 m/s) as well as being very rapidly attenuated in tissue. Their usefulness in elastography stems from the fact that shear wave speed is related to the tissues’ stiffness: they travel faster in stiffer tissues. Thus, measurement of the shear wave speed translates into a measure of tissue stiffness, and this method is quantitative. The data is read in m/s, and this can be converted into kilo Pascals (kPa), making some reasonable assumptions.
Shear waves are readily produced by any mechanical disturbance of the bodies’ tissues and are created physiologically with muscle contraction, including cardiac action. In MR elastography, a vibrator, similar to a loudspeaker, is attached to the body surface to emit a low frequency sound. For ultrasound, most applications use acoustic radiation force, which displaces tissue away from the ultrasound beam as its energy is absorbed. This acoustic radiation force impulse (ARFI) moves the tissue just a few microns when color Doppler-like pulses are applied, so the MI is within recommended limits. The resultant shear waves may be tracked using conventional ultrasound pulses, but the high speeds in very stiff tissues (such as schirrous carcinomas) can be too fast to be tracked, resulting in failed shear wave speed measurements. This can be overcome, at least partially, by using ultrafast imaging in which the transmitted beam is not focused at all, but instead “illuminates” the entire field of view. Focusing is then performed on the signals received from all the transducers’ elements. Frame rates of several thousand per second can be achieved, and the shear wave display can be refreshed at more than a frame per second. The shear wave display is also usually presented as a color-coded overlay on the B-mode image, and this is quantitative so that measurement regions of interest can be placed. Conventionally, stiff is coded as red and soft as blue, in contradistinction to the strain elastography convention. To avoid confusion, the color bar needs to be checked before interpreting elastographic images.
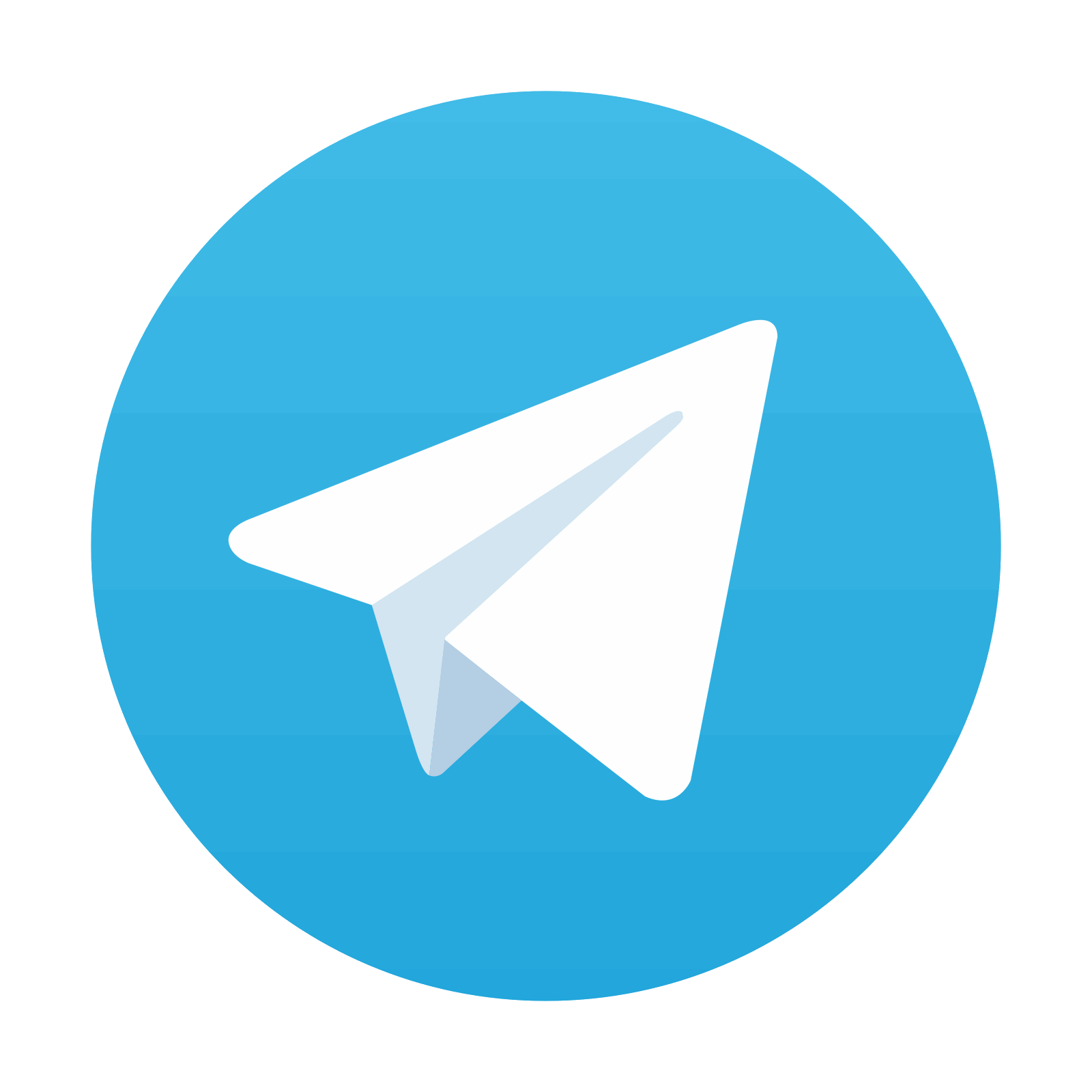
Stay updated, free articles. Join our Telegram channel

Full access? Get Clinical Tree
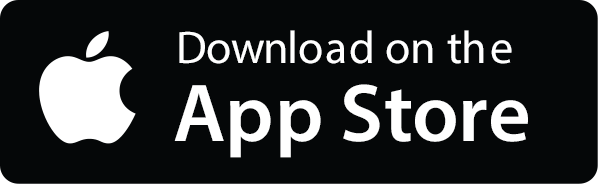
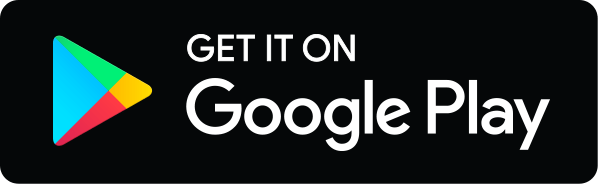