A physician’s knowledge of prenatal brain development in the context of maternal-placental health and disease greatly enhances the neurologic assessment of the newborn. Although acute neurologic signs after birth should be investigated aggressively for peripartum or neonatal causes, pathologic processes may also occur during prenatal life that subsequently modify neonatal brain functions. Any discussion of the neurologic evaluation of the newborn, therefore, must take into account historical and physical examination findings that integrate intrauterine and extrauterine periods, during which inherited and acquired components may synergistically alter fetal brain development in ways specific to gestational maturity. The evaluation of the neonate must consider familial, maternal, fetal, placental, and environmental factors to better determine the developmental niche of the fetus or neonate when stress or disease favorably or unfavorably alters structure and function because of time-sensitive strengths or vulnerabilities.
This chapter begins with a discussion of prenatal brain development, followed by three sections that each highlight a different perspective on accurate neurologic diagnosis: consideration of fetal neurologic consultations in the context of prenatal organ development and disease risk, serial and systematic bedside examinations, and laboratory investigations of the newborn, with emphasis on classic components of neurologic assessment. Finally, selected neurologic conditions are described that underscore the importance of integrating historical and examination findings in the evaluation of brain disorders of the fetus and neonate.
Stages of Prenatal Brain Development
Maturation of the brain is defined through descriptions of sequential and overlapping developmental processes, beginning with conception and involving continual interactions of the gene environment. Beginning during gestation and following trimester-specific stages of development of the embryo and fetus, anatomic, biochemical, and physiologic processes occur: neural induction followed by neuronogenesis, programmed cell death and neuroblast migration, formation of axons and dendrites, continuous energy generation to provide membrane excitability, synaptogenesis, neurotransmitter biosynthesis, and myelination of axons. These prenatal time periods are fundamental for brain development. However, postnatal processes of programmed cell death, continued synaptogenesis, and neurotransmitter maturation highlight important brain maturational events needed for continued preservation of appropriate structure and function. Regional differences in the rate of maturation of the nervous system also must be recognized. Different brain structures do not express equivalent function at specific times during the development of the fetus, premature infant, and full-term neonate. Table 18-1 summarizes the major prenatal developmental sequences in brain maturation that occur in the cerebrum and cerebellum and lists representative disorders at each stage. Both volume and gyral-sulcal complexity increase during prenatal development, with prominent changes in the last 3 months of gestation ( Fig. 18-1 ) reflecting major molecular and histologic maturational changes during the formation of maturing cortical-subcortical cellular connections.
Stage | Peak Time of Occurrence | Major Morphologic Events in Cerebrum | Major Morphologic Events in Cerebellum | Main Corresponding Disorders ∗ |
---|---|---|---|---|
Uterine implantation | 1 wk | |||
Separation of three layers | 2 wk | Formation of neural plate | Enterogenous cysts and fistulae | |
Dorsal induction Neurulation | 3-4 wk | Formation of neural tube, neural crest, and derivatives Closure of anterior (day 24) and posterior (day 29) neuropores | Paired alar plates | Anencephaly, encephalocele, craniorachischisis, spina bifida, meningocele |
Caudal neural tube formation | 4-7 wk | Canalization and regressive differentiation of cord | Rhombic lips (day 35), cerebellar plates | Diastematomyelia, Dandy-Walker syndrome, cerebellar hypoplasia |
Ventral induction | 5-6 wk | Forebrain and face (cranial neural crest) Cleavage of prosencephalon into cerebral vesicles (day 33) Optic placodes (day 26), olfactory placodes Diencephalon | Fusion of cerebellar plates | Holoprosencephaly, median cleft face syndrome |
Neuronal and glial proliferation | 8-16 wk | Cellular proliferation in ventricular and subventricular zone (interkinetic migration) Early differentiation of neuroblasts and glioblasts | Migration of Purkinje cells (9-10 wk) Migration of external granular layer (10-11 wk) | Microcephaly, megalencephaly |
Migration | 12-20 wk | Radial migration and accessory pathways (e.g., corpus gangliothalamicum) Formation of corpus callosum | Elaboration of the dendritic tree of Purkinje cells (16-25 wk) | Lissencephaly-pachygyria (types I and II), Zellweger syndrome, glial heterotopia, microgyria (some forms), agenesis of corpus callosum |
Organization † | 24 wk to postnatal | Late migration (to 5 mo) Alignment, orientation, and layering of cortical neurons Synaptogenesis Glial proliferation-differentiation well into postnatal life | Monolayer of Purkinje cells (16-28 wk) Migration of granules to form internal granular layer (to postnatal life) | Minor cortical dysplasias, dendritic and synaptic abnormalities, microgyria (some forms) |
Myelination | 24 wk to 2 yr postnatally | Dysmyelination, clastic insults |
∗ Disorders do not necessarily correspond to abnormal development. They may also result from secondary destruction or disorganization.
† Programmed cellular death takes place throughout the second half of pregnancy and the first year of extrauterine life.

Fetal Neurologic Consultations
The pediatric neurologist can fulfill a useful role as a subspecialty consultant for the fetus with a suspected brain disorder, given that neurologic disease may occur before the intrapartum period, either from a primary brain disease or secondarily from systemic diseases. Brain disorders detected in the neonatal period may also reflect fetal brain damage that occurred before dysfunction is first documented. Alternatively, medical conditions during the antepartum or intrapartum periods can predispose the fetus or neonate to express brain dysfunction at a later period, with either de novo or compounded brain injury. The pediatric neurologist must therefore consider maternal, placental, and fetal diseases on which a neonatal encephalopathy may be superimposed ( Box 18-1 ). This section describes how the neurologist uses an integrative approach to fetal neurology, emphasizing perspectives from other subspecialties such as maternal-fetal medicine, pathology, and neonatology, as well as additional pediatric subspecialties. Evaluation of future strategies for either fetal or neonatal brain resuscitation will need to consider the developmental context in which a suspected brain injury occurred during the antepartum, intrapartum, or neonatal period.
Lesions related to maternal pathologic conditions
-
Systemic diseases
- •
Maternal anemia
- •
Toxemia with chronic hypertension
- •
Renal diseases
- •
Repeated seizures during second trimester of pregnancy
- •
Severe hypoxia
- •
-
Maternal trauma
- •
Direct trauma to abdomen
- •
Maternal accidents
- •
-
Gas intoxication
- •
Carbon monoxide intoxication
- •
Butane intoxication
- •
Lesions related to fetal conditions
-
Twin gestation (especially with one macerated twin)
-
Prenatal arterial occlusions
-
Blood dyscrasias
- •
Hemolytic disease with or without fetal-maternal blood group incompatibility
- •
Thrombocytopenia (genetic, isoimmune, or of infective origin)
- •
-
Nonimmune hydrops fetalis
Lesions related to placental or cord abnormalities
-
Fetomaternal hemorrhage
-
Chronic placental insufficiency with fetal distress
-
Placental abruption
-
Cord knotting
Tools for Fetal Neurologic Diagnosis
Diagnostic techniques for evaluation of the fetus have improved over the past several decades, providing morphologic, biochemical, and physiologic diagnoses. Many medical conditions can be better documented during the prenatal period.
Indications for the use of these tests are enumerated in both obstetric and pediatric guidelines ( Box 18-2 ). Most of the following investigative tools are invasive and have specific indications.
- 1.
Amniocentesis is usually performed at 16 weeks’ gestational age or earlier if necessary. Fluid can be used for karyotyping as well as a variety of biochemical investigations. The most commonly performed tests are α-fetoprotein level and screening studies for specific chromosomopathies or neural tube defects. (See Chapter 2 .)
- 2.
Chorionic villous sampling can be undertaken at 8 weeks’ gestation or beyond, with specimens used for chromosomal studies by direct examination or culture as well as for biochemical analysis.
- 3.
Fetal blood sampling guided by ultrasonography can be performed from 18 weeks’ gestation onward to clarify ambiguous amniocentesis or chorionic villous sampling results and diagnose fetal infections, isoimmunization, or other hematologic problems.
- 4.
Ultrasound examination using transvaginal probes can detect structural abnormalities as early as the embryonic period, but abdominal probes are routinely used at 15 to 20 weeks’ gestation and the examination can be repeated as required. Evaluation of fetal maturity and the assessment of intrauterine growth are powerful measures for the antenatal diagnosis of many neurologic and nonneurologic fetal conditions. Table 18-2 lists selected brain malformations that can be diagnosed antenatally by ultrasonography. Newer techniques using three- and four-dimensional ultrasonography can show cerebral and noncerebral anatomic structures in greater detail. Although abnormal fetal ultrasonographic findings might be discovered fortuitously, previous pregnancy complications or current medical difficulties during early pregnancy usually prompt the proactive performance of one or more studies. In routine practice, the sensitivity of ultrasonography is not uniformly satisfactory, but reliability has improved ( Fig. 18-2 ). The addition of Doppler-visualized placental and umbilical blood flow adds valuable information regarding fetal stress secondary to uteroplacental insufficiency.
Table 18-2Major Malformations That Can Be Diagnosed Antenatally by UltrasonographyAdapted from Aicardi J, Bax M, Gillberg C, et al: Diseases of the nervous system in childhood, ed 2, New York, 1998, MacKeith Press, with permission.Diagnosis Earliest Gestational Age at Which Diagnosis Is Possible ∗ (wk) Percentage Diagnosed by 20-24 wk † Anencephaly 12-16 100 Encephalocele 12-20 75-100 Meningomyelocele 14-32 60-95 Hydrocephalus 20-36 25 Microcephaly ‡ 18-36 25 Callosal agenesis 20 Probably high Lissencephaly 20 Occasional reports ∗ Associated malformations are often the most obvious and can lead to discovery of central nervous system abnormalities.
† Frequency estimates using best technique available in 1990.
‡ This group includes cases of lissencephaly, true genetic microcephaly, and microcephaly caused by early destructive lesions.
Figure 18-2 Standard planes for viewing cerebral structures. A, Thalamic view at 20 menstrual weeks showing thalamus-hypothalamus complex (t), ambient cistern (solid arrow), insula (open arrow), tips of the anterior frontal horns of the lateral ventricles (v), and cavum septi pellucidi (c). B, Ventricular view at 18 weeks. The ventricle measurement is indicated (arrowheads). The tips of the anterior frontal horns (arrows) and cavum septi pellucidi (c) are visible. C, Cerebellar view at 18 menstrual weeks. The cerebellar hemispheres (arrows) and cisterna magna (m) are indicated. D, Coronal view at 19 weeks through the coronal suture showing anterior frontal horns (black arrows) and large nerve trunks; the fornices (white arrows) are clearly visible below the cavum septi pellucidi (c). E, Midsagittal view through the metopic suture at 19 weeks showing the normal corpus callosum (arrows) containing the cavum septi pellucidi in its arc below the corpus callosum.(From Rumack CM, Wilson SR, Charboneau JW, et al, editors: Diagnostic ultrasound, ed 4, St Louis, 2011, Mosby, Figure 34-2.) - 5.
Fetal neuroimaging provides additional anatomic information for the clinician regarding normal and abnormal structure. Magnetic resonance imaging (MRI) technology provides greater detail regarding gray and white matter structures, and can now detect anatomic correlates of pathophysiologic mechanisms related to asphyxia and/or inflammation that affect water diffusion and lead to edema (e.g., diffusion-weighted imaging).
- 6.
Biochemical, cytogenetic, and molecular biological techniques can assess for specific enzymes or other biochemical products that bear a relationship to specific genetic conditions. Techniques of DNA analysis make possible prenatal diagnosis of specific inherited biochemical abnormalities, detection of mutant genes, and linkage studies with fragment-length polymorphisms or other DNA markers for a specific familial condition.
- 7.
Techniques are available to assess for physiologic fetal well-being versus distress. In utero surveillance of physiologic functions of the fetus can also be achieved using fetal ultrasonography. Specific fetal activities that can be monitored include gross body movements, eye movements, sucking movements, heart rate patterns, and respiratory patterns, and quantitative determination of amniotic fluid volume is possible. Components can be combined to calculate a fetal biophysical score, which may reflect fetal physiologic well-being. Early or midgestation assessments are controversial; during the last trimester, studies of fetal behaviors may help document altered functional brain maturation by defining dysfunctional fetal organization of different behaviors.
- 8.
Intrauterine fetal therapies involve direct intervention on the fetus by a variety of techniques to treat specific conditions. For instance, fetal exchange transfusion is used to treat the anemia and secondary consequences of hematologic disorders. Withdrawal of fluid from body cavities (e.g., pleural, peritoneal), catheterization of the bladder, and, in rare situations, fetal surgery for hydrocephalus can be considered.
General risk factors ∗
∗ Mostly for chromosomal anomalies.
Maternal age 35 years or older at time of expected delivery †
† Risk of Down syndrome increases significantly after 35 years. Other chromosomal abnormalities are also increasingly common after this age with double or triple the overall risk.
Specific risk factors
Previous child with malformation or chromosomal abnormality
History of stillbirth or neonatal death
Structural abnormality in mother or father (e.g., neural tube defect)
Balanced chromosomal translocation in one parent
Family history of inherited disease in first-degree relatives
Maternal diabetes mellitus, phenylketonuria, exposure to teratogens, or some infectious diseases
Risk factors specific to certain ethnic groups
Tay-Sachs disease (screening in Ashkenazi Jews of Eastern European origin)
Sickle cell disease (screening in African and African American blacks)
Thalassemia (screening in some Mediterranean and some Asian populations)
The Consultation Process
Advances in neuroscience at the bench and bedside throughout the last several decades have refocused attention on the fetal origins of neonatal brain diseases. Discussions of the causes for fetal and neonatal brain injury have been reviewed. Stevenson et al highlight maternal, placental, and fetal factors that may contribute to encephalopathy and subsequent functional impairments in the newborn. A consensus report by a multidisciplinary task force reviewed medical literature regarding neonatal encephalopathy and cerebral palsy, emphasizing antepartum and intrapartum factors that need to be considered in determining the pathogenesis and pathophysiology of neonatal brain disorders. Pediatric neurologists who provide either fetal or neonatal consultations need to consider antepartum and intrapartum factors when offering recommendations for brain resuscitation to either the perinatologist or neonatologist, as novel therapeutic opportunities are made available.
Initiation of Fetal Neurologic Consultations
A physician’s formulation of a medical differential diagnosis always begins with fact finding and incorporates relevant historic information into the diagnostic fabric of the patient’s medical presentation. Although the investigation begins with the history of the present illness, consideration of medical and family histories may augment the physician’s understanding of the present abnormal medical condition. These general guidelines can be applied to fetal neurologic consultations. The information obtained regarding the preconception and pregnancy health of the mother, fetal well-being, and placental function must always include details of acquired environmental stresses and adverse family medical histories to allow the physician to unravel the diagnostic puzzle.
The pediatric neurologist will be called upon to offer opinions on fetal neurologic issues as part of the interdisciplinary fetal consultation team. Such input may be requested at any time during the mother’s pregnancy. This multispecialty team (sometimes referred to as the fetal board ) generally holds regular meetings, coordinated by maternal-fetal specialists, to discuss case histories of maternal-fetal pairs. The pediatric neurologist can provide an important perspective to this multidisciplinary group regarding neurologic diagnoses, while also considering input from the perinatologist, neonatologist, and other subspecialists. The pediatric neurologist can clarify findings that may suggest normal variation of the brain rather than a disease entity and provide experienced views regarding long-term prognosis.
The neurologist provides a balanced perspective on a neurologic diagnosis that is based on the structural and functional expressions of a brain disease or anomaly. Although structural brain anomalies, such as myelomeningocele, can be detected by ultrasonography, anticipation of postnatal bulbar dysfunction cannot always be predicted from prenatal imaging in the absence of polyhydramnios. Cranial nerve deficits evident on postnatal examinations will drastically alter the neurologist’s prognostic assessment.
Fetal neurologic consultations can also be initiated because of the presence of systemic maternal or fetal disease entities. Hypertensive disorders or autoimmune disorders in the mother during pregnancy, for example, predispose some fetal patients to thrombophilia, and thrombo-occlusive disease occurs in the brains of some children. Careful serial documentation of brain structures and cerebrovascular integrity around the circle of Willis by fetal ultrasonography needs to be performed to detect possible blood flow compromise with parenchymal brain injury. Systemic fetal diagnoses such as hydrops fetalis, for example, raise suspicion of cerebrovascular compromise of the fetal brain because of reductions in end-diastolic placental flow documented by ultrasonographic Doppler studies.
Information regarding the structural-functional expression of fetal brain disorders must be communicated by the pediatric neurologist not only to other subspecialists on the fetal board but also to the primary care physician in family practice or pediatrics who will oversee the general health care of the child after birth. Finally, the child neurologist has the opportunity to establish an early relationship (during the prenatal period) with the family of a child with a brain disorder, which will facilitate continuity of care after the birth both in the neonatal unit and the outpatient service.
Timing of Fetal Neurologic Consultations
On occasion, preconceptional consultations will be requested when the medical diagnoses of the parents or other siblings with neurologic disorders may affect the decision making of parents who wish to conceive additional children. More likely, the pediatric neurologist begins the consultation during the second or third trimester, depending on prenatal diagnostic findings supplemented by other information, after referral by the high-risk perinatal service. This consultation may include a review of the results of abdominal imaging, Doppler flow studies showing placental perfusion, nonstress tests to assess state stability in the fetus, and cytogenetic testing interpreted by maternal-fetal specialists and geneticists. Given the greater diagnostic accuracy of genetic screening tests or transvaginal imaging performed during the first trimester, brain disorders may be detected during the embryonic period of development (i.e., sooner than 56 days after conception). At the other extreme, consultations may be initiated only after birth when neurologic problems are first detected or suspected.
The grave prognostic implications of triploidy of chromosomes 13 to 15 or 18, for example, may be pointed out by the geneticist or maternal-fetal specialist, who will ask the neurologist to discuss the low chances for survival and poor quality of life because of associated brain anomalies such as the holoprosencephaly syndromes. Cardiologists and nephrologists may identify anomalies in these organ systems that carry associated risks to the nervous system. The deletion syndrome involving the short arm of chromosome 22 (velocardiofacial syndrome), for instance, is associated with cardiac and other organ system anomalies in addition to those in the central nervous system (CNS) in a percentage of children.
Diagnostic Process of Fetal Neurologic Consultations
Fetal Considerations: CNS-Specific Anomalies
Care of fetal patients with primary CNS anomalies requires input from the neurologic consultant. Estimates are that 50% of all cases presented to a multidisciplinary fetal board involve primary CNS anomalies. An opinion may also be requested from the pediatric neurosurgery service, since surgical intervention after birth may be considered for hydrocephalus or myelodysplasia. Even if the CNS anomaly is the starting point for the multidisciplinary term, a brain anomaly may be a surrogate marker for non-CNS abnormalities or genetic syndromes. For example, the presence of holoprosencephaly may suggest triploidy of chromosomes 13 to 15 or 18, non-CNS organ anomalies (e.g., cardiac lesions), or cholesterol dysmetabolism associated with underdeveloped genitalia (Smith-Lemli-Opitz syndrome).
Structural abnormalities in the brain can be detected by prenatal imaging (See Table 18-2 ) and represent a spectrum of disorders that involve different parts of the CNS, with implications for involvement of multiple organ systems. Knowledge of prenatal brain development provides perspective regarding timing, pathogenesis, and pathophysiology of congenital or acquired brain lesions. The pediatric neurologist, however, may not always accurately predict postnatal developmental trajectories during fetal consultations. Continuity of care for children with neurologic disorders provides an accurate long-term perspective on functional plasticity at successively older ages.
An example of a more nonspecific, and consequently problematic, prenatal imaging finding is fetal ventriculomegaly. At the initial ultrasonographic study, documentation of enlarged ventricles does not allow immediate insight into whether a progressive process representing hydrocephalus exists or compensatory fetal ventriculomegaly has arisen because of a failure of adequate brain growth with resultant enlargement of the ventricular and cisternal spaces. Hydrocephalus in utero requires serial ultrasonographic evaluations to document progressively decreasing cortical thickness and progressively increasing ventricular size. Only after 24 weeks’ gestation do changes in the occipital-frontal circumference reliably indicate progressive ventriculomegaly suggesting hydrocephalus. Ventriculomegaly is also a nonspecific anatomic finding that can be a marker for associated nervous system anomalies such as myelomeningocele, Dandy-Walker malformation, Chiari malformation, and a variety of genetic syndromes ( Fig. 18-3 ). Fetal MRI may delineate CNS anomalies better than fetal ultrasonography.

Ultrasonographic detection of other major body anomalies as well as cytogenetic and serologic findings from amniocentesis may suggest syndromic, chromosomal, and infectious disorders associated with ventriculomegaly. The perinatal team must develop the best delivery strategy and postnatal treatment of the infant with suspected progressive ventriculomegaly.
A second nonspecific ultrasonographic finding that may suggest a variety of CNS anomalies is the intracranial cystic lesion. Usually detected during the second or third trimester, these lesions can arise from multiple causes ranging from congenital to acquired conditions. When such intracranial lesions have been detected by transabdominal ultrasonography, fetal MRI studies should be performed to more definitively visualize intracranial lesions and surrounding brain structures. One must always distinguish destructive from congenital lesions. A cystic lesion is more likely to be congenital if it occurs during the first half of pregnancy (e.g., encephaloclastic lesions such as schizencephaly or arachnoid cysts) ( Fig. 18-4 ), whereas acquired lesions from intravascular occlusive events generally occur later during the second half of pregnancy (e.g., encephalomalacia from stroke syndromes associated with maternal preeclampsia or fetal thrombotic vasculopathy of the placenta). Thrombophilia predisposes the fetus to intravascular occlusive events within either the arterial or venous circulation of the brain. Fetal stroke occurs in approximately 1 in 4000 live births and can be associated with multiple maternal, placental, and fetal diseases.

Fetal Circulatory and Vascular Disorders
Bothing ischemic and hemorrhagic cerebrovascular lesions can result from circulatory disorders of the mother, fetus, or placenta.
The consequences of intrauterine circulatory and vascular disorders related to systemic diseases of the mother, including maternal anemia, hypertensive disorders of pregnancy, and uncontrolled maternal seizures leading to severe hypoxia, may present only after birth in the immediately neonatal period. Direct trauma to the mother’s abdomen, indirect consequences of maternal accidents, and gas intoxication by carbon monoxide or butane poisoning are other examples of maternal pathologic conditions that promote fetal vascular brain injury. Vascular lesions related to fetal conditions include vascular disruptions associated with multiple gestation, particularly when one macerated twin is present ( Fig. 18-5 ); prenatal arterial occlusions caused by altered angiogenesis; blood dyscrasias due to hemolytic disease or thrombocytopenia; and hydrops fetalis with hematologic, infectious, or other congenital causes. Finally, placental or cord anomalies, including fetal-maternal hemorrhage, chronic placental insufficiency with fetal distress, placental abruption, true cord knots, and long or short cords may contribute to vascular compromise in the fetus in either the antepartum or intrapartum period (see Box 18-1 ).

Circulatory disturbances affect the fetal brain differently depending on the stage of brain development. Brain injuries before the seventieth day in utero result in abnormal migratory patterns of neuronal groups within the white matter or neocortex without major cavitation, whereas later injuries result in destructive (encephaloclastic) lesions caused by ischemia or hemorrhage. For example, schizencephaly may be the result of faulty vascular supply to the developing neocortex before the seventieth day of gestation, which leads to a “true porencephaly” with an ependymal lined cleft or tract between the intraventricular and subarachnoid spaces.
In general, the migration of neocortical cells, angiogenesis of the blood vessels, and gliogenesis of supportive cellular elements occur in an overlapping manner. Therefore, it may be difficult to predict precisely the specific brain injury because of the unknown timing of the insult or insults that precede or follow critical stages of brain maturation. Circulatory disturbances generally damage the periventricular white matter of the preterm infant. Ischemic or hemorrhagic injuries in the preterm brain tend to occur between 26 and 34 weeks’ gestation, whether they occur prenatally in the fetal brain or postnatally in the preterm infant’s brain. On the other hand, cortical, subcortical, and basal ganglia regions are more susceptible to injury after 34 weeks’ gestation in either the near-term or term infant, because of the more advanced maturation of brain vasculature. These vascular lesions may also be produced before or after birth, depending on the timing of the insult after 36 weeks’ gestation. This topic is discussed in greater detail in the section on asphyxia.
Clinical manifestations of fetal circulatory and vascular disorders may be difficult to detect, either before or after birth. The presence of unexpected alterations in fetal movements, as perceived by the mother or with abdominal ultrasonography, may be a helpful sign, but such alternations are observed largely through serendipity. Fetal growth restriction, hydrops fetalis, and hydramnios are examples of other, more obvious suspicious features. Multiple gestation pregnancies and maternal trauma can also be associated with circulatory abnormalities in the fetal brain. However, vascular lesions may result even in the absence of documented maternal, fetal, or placental disorders.
After birth, specific clinical and laboratory findings may suggest in utero brain injury related to circulatory disturbances. Marked neonatal anemia, microcephaly at birth, marked rigidity or spasticity, or isolated seizures in the absence of post–hypoxic-ischemic brain disorder raise suspicions of antepartum disorders. Early neuroimaging (computed tomography [CT] or MRI) within 48 hours after birth should distinguish an acute from a chronic brain lesion. Specific types of CT or MRI images (e.g., inversion-recovery sequences or diffusion-weighted views) may distinguish acute stages of cellular edema with transmembrane diffusion of intercellular and intracellular fluid contents (whatever the cause, but presumably after a recently occurring pathogenetic process such as asphyxia, infection, trauma) from gliotic scarring, irregular ventricular borders, ventriculomegaly, or brain maldevelopment, which imply a remote brain injury.
Inherited Metabolic and Neurodegenerative Diseases of Fetal Onset
Although it may be difficult to definitively identify children with metabolic or neurodegenerative disorders during fetal life, certain clues can raise concerns before or after birth. Specific disease entities may present in the neonatal period with hypotonia, decreased levels of arousal, or intractable neonatal seizures inappropriate to events around birth. Decreased arousal or coma after formula feedings may suggest a biochemical disorder involving carbohydrate, protein, or fat metabolism. A constellation of minor brain or somatic anomalies may heighten the physician’s clinical suspicions. Limb contractures, an underdeveloped thorax, or decreased muscle mass, for instance, may suggest congenital neuromuscular diseases. Careful ophthalmologic evaluation of the anterior chamber of the eye may document an anterior chamber (i.e., embryotoxon) anomaly associated with peroxisomal diseases, or colobomata of the iris or retina, which represent a nonspecific arrest in ocular development caused by either metabolic and genetic or developmental disorders. Association with nonimmune hydrops or intrauterine growth restriction may also be a clue to the diagnosis. Careful inspection of placental specimens may document destructive lesions, vascular lesions, congenital anomalies, or storage material that reflects the timing or cause of disease states ( Fig. 18-6 ).

Fetal Considerations: Non-CNS Anomalies
The fetus with brain disorders may initially show abnormalities of other organs. Important diagnostic clues for the fetal or neurologic consultant are provided by a variety of systemic fetal conditions, as suggested by Table 18-3 . Regardless of which organ system is the starting point for a multidisciplinary medical discussion, associations with the nervous system must be considered, because non-CNS anomalies may be surrogate markers for CNS disease. Seventy-eight percent of fetal neurologic consultations provided to 166 maternal-fetal pairs involved systemic disease conditions, with or without CNS anomalies. For example, children with the midgut anomaly omphalocele can have associated neural tube defects.
Nonneurologic Finding | Neurologic Diagnosis |
---|---|
Cardiac rhabdomyoma | Tuberous sclerosis |
Hypoplastic left heart syndrome | Brain malformations (e.g., microgyria, agenesis of corpus callosum) |
Multicystic dysplastic kidney | Brain malformations with specific genetic syndromes vs. destructive brain lesions |
Diaphragmatic hernia | Brain malformations (e.g., cerebellar hypoplasia) |
Polyhydramnios | Brain malformations with genetic syndromes or destructive brain lesions |
Hydrops fetalis | Congenital syndromes (e.g., Turner syndrome) or destructive brain lesions, usually of vascular or infectious origin (e.g., asphyxia, parvovirus infection, metabolic disorders) |
Cleft lip and palate | Midline brain malformations (e.g., holoprosencephaly) |
Arthrogryposis | Neuromuscular disease or destructive brain lesions (e.g., congenital muscular dystrophies) |
Multiple gestation pregnancy | Destructive brain lesions of white or gray matter (e.g., periventricular leukomalacia) |
Omphalocele/gastroschisis | Neural tube defects |
Generalized systemic medical conditions also predispose the fetal brain to harmful effects. For example, multiple gestation pregnancies may result in twin-to-twin transfusion syndrome, which is seen in 5% to 15% of all twin pregnancies, with the most severe form occurring in 1% of monochorionic gestations. Twin-to-twin transfusion syndrome has hematologic consequences that lead to overperfusion or underperfusion of the siblings. Polycythemia and hydrops fetalis are two possible complications, and these conditions predispose the unborn child to cerebrovascular injury from hypoperfusion caused by either hyperviscosity or ischemia, possibly with accompanying thrombophilia. Significant growth discrepancy with growth restriction in the donor twin may result. End-diastolic volume, as determined by Doppler flow ultrasonography, may become compromised, with loss of placental flow to one or both twins. If one twin dies in utero, the surviving fetus will have a significantly increased risk of cerebrovascular injuries and may later exhibit cerebral palsy. Associated placental abnormalities also have been described in children who experienced brain injury manifesting as motor deficits.
Another systemic presentation of fetal disease with adverse consequences to the brain is hydrops fetalis. The diagnosis of hydrops fetalis requires documentation of fluid accumulation in serous cavities and edema of the soft tissues of the fetus, which can be documented by transabdominal ultrasonography ( Fig. 18-3 ) (see also Chapter 2 ). Most clinical series include isolated fetal ascites in the definition of hydrops fetalis, with an incidence of approximately 1 in 2500. The causes of most cases of hydrops fetalis remain nonspecific, involving maternal, fetal, or placental disorders. Associated conditions include congenital heart disease, triploidy, Turner syndrome, cystic hygroma, twin pregnancies, hematologic disorders, diaphragmatic hernia, gastrointestinal problems, maternal diabetes, placental-cord diseases, congenital infections, and inborn errors of metabolism.
Hydrops fetalis is an important pathologic condition that may predispose the child to fetal or neonatal encephalopathies. Cerebral injury and genetic or metabolic diseases of the brain may present in general as hydrops fetalis on fetal ultrasonography. Fetal brain vasculopathies may manifest as stroke syndromes or intracranial hemorrhage in patients with hydrops fetalis. Neonatal encephalopathies expressed after birth in neonates with hydrops fetalis may also reflect remote fetal brain injury, usually from hypoperfusion or thrombo-occlusive disease that occurred during the third trimester due to hydrops fetalis.
Infectious Diseases
Both the embryo (<8 weeks’ gestation) and fetus (>8 weeks’ gestation) are vulnerable to a number of infectious agents. Infections during the first and early second trimesters result in congenital malformations more commonly than in destructive lesions. Later infections during the third trimester generally result in destructive changes in the brain. The inflammatory response provoked by infectious agents leads to glial scarring of the brain, usually after 26 to 28 weeks’ gestation.
The brain may appear markedly atrophic, with calcification of neurotic areas, as documented by CT scans of the head after birth (see also Chapter 14 ). Major destructive lesions may also be noted on fetal sonograms or neonatal neuroimaging scans (see Fig. 18-4 ). The most common infections that can affect fetal brain integrity and development are those caused by cytomegalovirus, rubella virus, herpes simplex virus, Toxoplasma gondii, Treponema pallidum, and human immunodeficiency virus (HIV).
Clinical manifestations in the neonate of fetal infectious disease may include organomegaly, intrauterine growth restriction, jaundice, microcephaly, intracranial calcification, osseous lesions, encephalitis, and chorioretinitis, particularly with cytomegalovirus infection. Specifically for rubella, any combination of brain, eye, heart, or ear involvement is possible, and some infants may show irritability, lethargy, and hypotonia at birth, whereas others experience only hearing loss, depending on when the fetus was infected. Infants with herpes simplex infections may be acutely ill during the period immediately after delivery or may become symptomatic over the first several weeks of life. Intractable seizures and coma may also highlight their neonatal clinical course. Congenital toxoplasmosis can take a severe neonatal form that includes hepatosplenomegaly, fever, and purpura. Ventricular dilatation may exist in utero, as documented by fetal ultrasonography, and chorioretinitis is a common feature seen on retinal examination in the newborn period. The clinical presentation of an infant with congenital syphilis also includes hepatosplenomegaly, retinitis, and osteochondritis, but these may appear only after several months of life, along with irritability, vomiting, cranial nerve deficits, and chronic hydrocephalus. HIV infection can be transmitted as early as 15 weeks’ gestation; most HIV-infected neonates are asymptomatic with positive serologic results for up to several years. Diagnosis in the neonatal period is generally difficult. However, some infants receive a large viral load in utero and may be born prematurely with microcephaly and calcification of the basal ganglia.
Less common viruses that also affect the brain but have no consistent pattern of fetal injury include influenza virus, measles virus, hepatitis virus, variola virus, and enteroviruses and adenoviruses. Infection with one virus, parvovirus B19, has been associated with nonimmune hydrops fetalis and may indirectly affect fetal brain integrity by causing uteroplacental insufficiency leading to vasculopathies.
Exogenous and Endogenous Toxic Disorders
Although the placenta is usually an effective barrier between maternal and fetal circulations, specific exogenous or endogenous toxins may nonetheless reach the fetus to produce malformations or destructive disturbances, depending on the timing of the toxin exposure. Examples of such exogenous agents are therapeutic agents, industrial pollutants, and recreational substances. Some of the main agents producing neurologic injury are listed in Table 18-4 , which also describes the various patterns of damage that may occur. An example of endogenous toxicity is maternal diabetes mellitus. All forms of diabetes may produce problems for the fetus and neonate. Careful control of maternal diabetes can prevent most major congenital malformations of the brain and spinal cord, yet developmental and destructive insults can result during fetal life, during parturition, or after birth, including immaturity of brain development, sacral agenesis, intracranial venous thromboses from hyperviscosity-polycythemia syndrome, and peripheral nerve injury from shoulder dystocia caused by impaction of a hypotonic-macrosomic fetus against the pelvic inlet during delivery. Postnatal polycythemia, hypoglycemia, and hypocalcemia all may result in neonatal seizures or coma, or both.
Category | Pattern of Damage |
---|---|
THERAPEUTIC AGENTS | |
Antiepileptic drugs (phenytoin, barbiturates, carbamazepine, diones, sodium valproate) | Fetal growth restriction, small head, dysmorphism of face and fingers, clefts, congenital heart disease, and other defects |
Benzodiazepines | Poorly defined |
Warfarin and other Coumarin derivatives | Punctate chondrodystrophy, deafness |
Vitamin A | Hydrocephalus, ear, and heart anomalies (uncertain) |
Retinoic acid, isotretinoin | Central nervous system migration disorder |
INDUSTRIAL POLLUTANTS | |
Methylmercury | Abnormal neuronal migration, deranged cortical organization |
Polychlorinated biphenyls (PCBs) | Microcephaly, large fontanelles, behavioral disturbances |
Carbon monoxide | Hypoxic-ischemic lesions |
RECREATIONAL SUBSTANCES | |
Alcohol | Fetal growth restriction, facial dysmorphism, brain malformations with excess neuronal migration, and other central nervous system defects |
Narcotics (heroin, codeine, methadone) Other street drugs (amphetamines, pyribenzamine, phencyclidine) Cocaine | Virtually all such substances may produce fetal growth restriction, and narcotics may produce withdrawal symptoms in neonates. In addition, cocaine can induce placental abruption and fetal death and may be responsible for skull and brain malformations and vascular damage with infarcts or hemorrhage. |
Toluene and other inhalants | Microcephaly, minor craniofacial anomalies, limb anomalies |
Tobacco | Fetal growth restriction, possible effects on cognitive development |
Immunologic and Blood Disorders in the Fetus
Blood type incompatibility has classically been associated with erythroblastosis fetalis and can be prevented by isoimmunization and the administration of globulins. Blood dyscrasias of either an immune or a nonimmune origin can also contribute to hydrops fetalis; the association of hydrops fetalis with cerebrovascular lesions in the fetus was described earlier. Nonimmune hydrops fetalis may occur after infection with parvovirus B19 or as a result of various inherited metabolic disorders, cardiac diseases, or chromosomal diseases such as Turner syndrome. Fetal-maternal and twin-to-twin transfusion syndromes are also frequently implicated in fetal brain disease, which usually results from ischemic or hemorrhagic injuries caused by venous stasis (i.e., hyperviscosity-polycythemia syndrome) or arterial ischemia (i.e., anemia). Intrauterine thrombocytopenia resulting from isoimmunization against fetal platelets or idiopathic causes may produce fetal brain damage. The inflammatory process has been implicated in cerebral palsy.
Intrauterine Growth Restriction
Fetal growth restriction increases the risk of neurologic problems. Intrauterine growth restriction is defined in various ways, usually as somatic growth that is less than the 2nd percentile or at least 2 standard deviations less than the mean for gestational age (see also Chapter 5 ). Symmetric growth restriction and asymmetric growth restriction imply different time courses for a disease state. A fetus with early gestational disorders from chromosomal or syndromic conditions is more likely to demonstrate balanced growth restriction (i.e., both head and somatic growth compromised). Asymmetric growth restriction occurs during the last trimester in response to acquired deficits, which tend to spare head growth. Infants who are small for gestational age as a compensatory response to stress in utero may escape subsequent neurologic sequelae, whereas other infants with intrauterine growth restriction may experience brain injury. However, a subset of infants with more subtle reductions in somatic dimensions (i.e., lower ponderal index) may have more pervasive disorders or diseases that occur closer to parturition. The fetus with intrauterine growth restriction exhibits malformations or dysmorphic syndromes at a much higher rate than the general population (5% to 15%). Therefore, the possible association of intrauterine growth restriction and brain injury should be considered in the context of other historical and physical examination factors.
Maternal-Placental Considerations
Fetal neurologic consultations can be initiated because of maternal disorders such as diabetes mellitus, pregnancy-induced hypertension, or specific organ diseases. A woman’s history of miscarriages in early pregnancy may suggest genetic or acquired risks to the fetus resulting from inherited forms of thrombophilia or recurring infection. Thrombo-occlusive disease (e.g., stroke, heart disease) at younger ages in family members also suggests the possibility of these disease entities in the mother.
Placental anomalies also suggest increased risks to the fetus. An abnormally large or small placenta, anomalies of the umbilical cord, and structural anomalies to the placenta may adversely affect fetal well-being. Velamentous insertion of the cord, for example, puts the child at risk for later exsanguination if umbilical vessels separate during the delivery process. Vascular changes within maternal or fetal placental vascular beds, such as chorioangiosis, maternal floor infarction, and fetal thrombotic vasculopathy, imply hypoperfusion of the fetus.
Consultations during the Peripartum and Neonatal Periods
Knowledge of adverse events around the puerperal period is important to the pediatric neurologist who later participates in neurointensive neonatal consultation. The mother’s report of the quality and quantity of fetal movements before the onset of labor, as well as specific information from fetal surveillance close to and during labor, need to be considered. Abnormal findings on nonstress tests and biophysical profiles may provide important clues to fetal distress during the period preceding or during parturition. Accurate information regarding the length of labor and delivery and any associated complications must be provided for the neurologist’s consideration. Fetal heart rate patterns, scalp and cord pH values, Apgar scores, and the degree of neonatal resuscitative efforts can help characterize an evolving neonatal brain disorder in the context of adverse conditions before and during labor and delivery. Fetal distress, as reflected by fetal heart rate abnormalities, uncommonly indicates intrapartum brain injury and instead usually reflects antepartum causes. For the individual neonate, however, severe and abrupt changes in markers of fetal well-being, such as scalp pH or fetal heart rate, denote risks for acute brain injury.
During the period immediately after birth, the neurologist must be cognizant of the infant’s extrauterine adaptation following the stresses of labor and delivery. Altered arousal, altered muscle tone, and seizures are the three principal clinical components of an evolving encephalopathy and sometimes occur after a stressful experience during parturition. However, the infant’s initial metabolic acidosis, neonatal depression, and hypotonia may not evolve into a persistently altered state of depressed arousal, hypotonia, and seizures. Rapid resolution of metabolic acidosis and improvement in Apgar scores without the need for resuscitation indicate that a neonatal encephalopathy is less likely to develop. Specific examination findings may preferentially reflect fetal brain disorders even in the encephalopathic newborn. Intrauterine growth restriction, joint contractures, and hydrops fetalis are examples of clinical findings that suggest longer-standing fetal diseases, which predispose the fetus to intrapartum distress and neonatal depression with hypotonia immediately after birth and during delivery room stabilization. The rapid expression of hypertonicity, sometimes with cortical thumbs, in an initially neurologically depressed neonate who rapidly returns to wakefulness after vigorous resuscitation usually suggests chronic fetal neurologic disorder.
By contrast, after an intrapartum asphyxial stress as documented by severe metabolic acidosis at birth (pH of <7.00), a depressed 10-minute Apgar score (<3), sustained hypotonia, and unresponsiveness with seizures throughout the next 3 to 7 days suggest an evolving hypoxic ischemic encephalopathy. These general encephalopathic features consisting of a depressed sensorium and hypotonia (with or without seizures), however, may also be expressed by infants with fetal (i.e., antepartum) brain injury that is superimposed on neonatal brain dysfunction after a stressful labor and delivery. It may be impossible in a particular infant to differentiate neonatal encephalopathy from preexisting antepartum brain injury that occurred only hours to a few days before the start of active labor. A presumption of fetal brain injury might be supported by the presence of preexisting maternal disease, chronic placental-cord abnormalities, specific persistent neuroimaging findings, or neuropathologic findings on postmortem examination. Intrapartum asphyxial stress certainly can add further brain injury to previous damage in some infants. Newer neuroimaging techniques using diffusion-weighted MRI and magnetic resonance spectroscopy may help establish the timing of injury that occurred closer to events during labor and delivery.
The pediatric neurologist must unify all pertinent data from the antepartum, peripartum, and neonatal periods to arrive at the most accurate interpretation of the neonatal neurologic examination findings. Comparisons between prenatal brain images (obtained via abdominal ultrasonography or fetal MRI) and postnatal images are essential. Diffusion-weighted MRI images may help identify any acute or subacute cerebral edema. Although fetal MRI provides better resolution of brain structures than abdominal ultrasonography, continued brain development in utero may mislead the neurologist, who consequently offers inaccurate or incomplete anatomic diagnoses after birth. The postnatal evaluation of the infant must be incorporated into the neurologic consultation. Phenotypic features of either genetic or acquired medical conditions may not have been appreciated during fetal life.
Neurointensive care recommendations by the pediatric neurologist will involve recommendations for neuroimaging studies, neurophysiologic testing with electroencephalography or evoked potentials, as well as biochemical studies to investigate metabolic disturbances. Pathologic examination of the placenta and umbilical cord during the first day of life are essential to provide additional information on the etiology and timing of a fetal or neonatal brain disorder. All recommendations will be integrated with those of the neonatal team.
Approach to Neurologic Examination of the Newborn
Although the nervous system of the newborn is structurally and functionally immature, general strategies for performing a neurologic examination should parallel the bedside approach used with the older infant and child. Neuronal networks in the neonatal brain with limited dendritic and synaptic interconnections, as well as immature myelination patterns, contribute to the expression of immature neurologic function. Clinical neurologic signs therefore reflect subcortical structures to a larger extent and cortical function to a more limited extent. Judicious coordination of careful neurologic clinical examination and neurobehavioral state assessment, with electroencephalographic (EEG) and polygraphic analyses, can provide better assessment of brain function.
To reinforce the discussion in the previous section concerning fetal brain development and the unique susceptibilities of the fetal brain to maldevelopment or injury, the present discussion of the clinical evaluation of the newborn explores how the newborn’s clinical repertoire reflects prenatal, peripartum, and postnatal disease processes.
Systematic neonatal examination techniques must be followed in a sequential and repetitive fashion, emphasizing specific levels of the neuraxis ( Box 18-3 ). The preterm infant has a more limited clinical repertoire because of greater immaturity; yet, serial examinations can optimize the validity of normal or suspicious findings. Knowledge of the evolution of signs and symptoms over the early postnatal period can contribute to the clinician’s diagnostic and prognostic abilities. Abnormalities found on neurologic examination reflect the moment-to-moment functional integrity of the infant and may have little bearing on the location or extent of brain damage that occurred during fetal or neonatal life; this becomes more demonstrable only at older ages.
-
Level of alertness
-
Cranial nerves
- •
Olfaction (I)
- •
Vision (II)
- •
Optic fundi (II)
- •
Pupils (III)
- •
Extraocular movements (III, IV, VI)
- •
Facial sensation and masticatory power (V)
- •
Facial motility (VII)
- •
Audition (VIII)
- •
Sucking and swallowing (V, VII, IX, X, XII)
- •
Sternocleidomastoid function (XI)
- •
Tongue function (XII)
- •
Taste (VII, IX)
- •
-
Motor function
- •
Tone and posture
- •
Motility and power
- •
Tendon reflexes and plantar response
- •
Primary neonatal reflexes
-
Moro reflex
-
Palmar grasp
-
Tonic neck response
-
- •
-
Sensory function
Estimation of Gestational Age
Estimation of the maturity of an infant is crucial to understanding the neurodevelopmental niche in which the infant is situated when an examination is performed. Responses to the neonatal neurologic examination change with the infant’s maturational level. Disease entities also express different characteristics in newborns who are born appropriate for gestational age compared with those who are small for gestational age. Finally, specific types of insults to the brain have varying impact on different parts of the nervous system, depending on the child’s gestational maturity.
The most useful historical data for estimating gestational age, especially in preterm infants, is the date of the mother’s last menstrual period. Techniques for estimating gestational maturity by clinical examination are based on careful anthropometric measurements such as body weight, length, and head circumference, as well as observations of external characteristics such as body hair, skin texture, skin creases, and areola size. Ponderal index and body mass index reference curves for large neonatal populations identify more pervasive expressions of growth restriction not detected using standard growth curves. Laboratory evaluations also provide estimates of maturity; these include radiographic studies of bone growth, neurophysiologic measures of EEG or nerve conduction velocities, and neuroimaging descriptions of sulcal, gyral, and myelination features.
Characteristics of the Head
Examination of the head focuses on four areas: skin characteristics, head circumference, shape of the head, and rate of head growth.
The skin should be carefully inspected for the presence of (1) dimples or tracts, which can be associated with brain malformations; (2) subcutaneous masses that reflect trauma, tumors, or encephaloceles; and (3) cutaneous lesions, which may be associated with specific congenital vascular abnormalities or neurocutaneous syndromes, such as Sturge-Weber syndrome, linear sebaceous nevus syndrome, or incontinentia pigmenti. Description of skin lesions may help diagnose a medical condition with important manifestations in the brain. For instance, the port wine stain of Sturge-Weber syndrome can be associated with abnormalities in choroidal vessels in the eye and meninges, which may result in glaucoma and cortical lesions. Skin lesions may evolve with maturation. For example, pale macular lesions present in Sturge-Weber syndrome become more deeply stained red or purple with age ( Fig. 18-7 ).

Head circumference should be considered as a surrogate measure of brain and cerebrospinal fluid (CSF) volumes. Generalized or localized scalp edema affects the accuracy of the head circumference measurement. True macrocephaly or microcephaly can be estimated by determining whether the child’s head size is larger than the 97th percentile or smaller than the 3rd percentile, respectively.
The shape of the head also requires careful inspection. Skull deformities may result from acquired or congenital processes. Molding of the skull may be caused by a difficult vaginal descent and extraction. Scalp edema, cephalohematomas, or subgaleal hematomas may have occurred. Knowledge of all cranial sutures is necessary ( Fig. 18-8 ). Craniosynostosis (premature closure of a cranial suture) may be a congenital cause of a malformed shape. Sagittal synostosis is the most common type, leading to an elongated shape with a high forehead. Different head shapes result from closure of coronal, metopic, or lambdoidal sutures; several sutures may also be fused. Genetic disorders or endocrinologic syndromes such as Treacher Collins syndrome and congenital hypothyroidism can be associated with craniosynostosis.

Finally, the rate of head growth is extremely important to note on serial examinations. Appropriate postnatal growth rates are difficult to define, but certain generalities should be considered. Modest head shrinkage, reflected by overriding sutures, can be seen during the first several days in the near-term or term infant. Increases in head growth by a mean of approximately 0.5 cm in the second week, 0.75 in the third week, and 1 cm per week thereafter, should be expected for a healthy premature infant. The clinician must recognize that a sick preterm infant with systemic disease may require initial time for “catch-up” head growth, which may exceed the expected rate. However, extremes of growth arrest or excessive growth must be considered as part of a pathologic process (e.g., continued nutritional deprivation, genetic influences, or progressive hydrocephalus). The possibility of a pathologic condition should be considered in all infants with changes in head growth that are more than 2 standard deviations above or below the mean for all infants at that corrected age.
Among newborns of extremely low gestational age, microcephaly at 2 years, but not at birth, is associated with motor and cognitive impairment at age 2 years.
Comparison of relative head and face proportions may help elucidate genetic or acquired syndromes or conditions. For example, squaring of the forehead with frontal bossing may support a diagnosis of hydrocephalus, rickets, or skeletal dysplasias. Underdevelopment of the mandibular structures suggests congenital syndromes such as Pierre Robin sequence.
Levels of Alertness
As with a patient at any older age, the formal neurologic examination of the newborn should include an assessment of the level of alertness. Terms such as state or vigilance have been used in defining criteria for level of alertness, and these criteria usually describe the two initial states of quiet (state 1) and active (state 2) sleep, followed by progressively increased levels of arousal from an awake-sleep transition (state 3) through quiet wakefulness (state 4) and vigorous crying with wakefulness (state 5). The infant varies in levels of alertness depending on feeding or environmental stimuli, as well as disease states. Behavioral or polygraphic criteria help define these state transitions ( Table 18-5 ). Recent events in the nursery, such as exposure to painful procedures, bathing, feeding, or medication administration, also affect the infant’s state of alertness. Scores on qualitative scales of arousal and attention in the neonate and infant have important prognostic implications.
Eyes Open | Respiration Regular | Gross Movements | Vocalization | |
---|---|---|---|---|
State 1 | − | + | − | − |
State 2 | − | − | ± | − |
State 3 | + | + | − | − |
State 4 | + | − | + | − |
State 5 | ± | − | + | + |
Abnormalities in levels of alertness are one of the more common neurologic deficits noted in the newborn period. Such abnormalities may be subtle, and their detection requires consideration of environmental influences as well as the maturity of the infant. General categories of increased or decreased levels of arousal include hyperalertness, lethargy, stupor, and coma. The appearance of the infant in a resting state, his or her arousal response, and the quality and quantity of motor responses allow the examiner to estimate whether the infant should be considered normally alert versus hyperalert and irritable or stuporous versus asleep. Exaggerated, diminished or absent arousal responses and reduced motor responses are noted in the infant who is abnormally hyperalert or lethargic. The distinction between stupor and coma is also based on the quality and quantity of motor responses relative to the infant’s gestational maturity. After 28 weeks’ gestational age, stimulation consistently results in the infant’s waking for several minutes. By 32 weeks’ gestational age, no stimulation is needed for arousal. After 36 weeks’ gestational age, increased alertness is readily observed, and well-formed sleep-wake cycles are noted by term age. In general, the examiner should assume bilateral cortical dysfunction or subcortical disturbance of the reticular activating system within the gray matter of the diencephalon, midbrain, and upper pons if wakefulness cannot be achieved, even with vigorous stimulation. Diffuse or multifocal disease processes, including those with infectious, vascular, dysgenetic, metabolic, or toxic causes, can result in altered arousal.
Cranial Nerves
There are 12 pairs of cranial nerves that are identified by their specific functions within the cortex and brain stem. Cranial nerve functions and abnormalities referable to a particular region of the brain are elaborated in the following sections.
Olfaction (I)
Olfaction is often ignored in the neurologic evaluation of the neonate, but it may be affected by various disease conditions. A sensory stimulus such as a cotton pledget soaked with peppermint extract elicits a consistent response in an infant of 30 to 32 weeks’ gestational age, with a sucking arousal or withdrawal response; more immature infants normally lack this response. Olfactory discrimination has been demonstrated in the newborn, who prefers odors from the mother, and rapid associative learning occurs within 48 hours. An absence of this response should be considered in infants in whom the olfactory bulbs and tracts may not have developed, as is sometimes noted in disturbances of midline brain development such as holoprosencephaly. This condition could be present in infants of diabetic mothers, who have a higher risk of this type of brain anomaly.
Vision (II)
Specific visual responses are subserved by the second cranial nerves. Blinking to light begins by approximately 25 to 26 weeks of gestation ; by 32 weeks, infants sustain prolonged eye closure as long as the light source remains present. By 34 weeks of gestation, infants can track a large red object, and by 37 weeks, they can follow ambient light. Optokinetic nystagmus elicited by a rotating drum or striped cloth may be seen after 36 weeks’ gestational age. Anatomic localization for visual fixation and following responses does not require the occipital cortex and may be subserved by subcortical structures such as the superior colliculus of the midbrain and the pulvinar, which link with the retina and optic nerves.
It is far more difficult to study the visual abilities of acuity, color perception, contrast sensitivity, and visual discrimination in the newborn. Estimations of these responses can be obtained by careful observation of functional abilities using age-specific visual fixation devices. By 35 weeks’ gestational age, newborns prefer complex patterns with curved contours over straight lines. However, acuity, binocular visual acuity, and appreciation of depth perception vary widely in the newborn, and these rapidly improve only during the first 3 to 4 postnatal months. Therefore, the evaluation of visual function may be hampered by the difficulty of assessing these visual functions at the bedside. Nonetheless, infants with periventricular leukomalacia involving parieto-occipital regions exhibit delayed visual acuity when studied later in infancy.
Funduscopic examination of cranial nerve II is an extremely valuable aspect of the neurologic assessment of the newborn. Alterations in the color, depth of cup, and circumference of the optic discs may reflect significant disease processes. Careful inspection of the anterior chamber, retinal grounds, and external eye structures by a pediatric ophthalmologist is essential. Corneal clouding, glaucoma, cataracts, colobomata, and chorioretinitis are examples of ophthalmologic findings that have clinical importance for both acquired and genetic fetal disorders. Indirect ophthalmologic evaluation by the pediatric ophthalmologist more fully examines the fundus, particularly the posterior pole of the retinal surface. Major abnormalities of the optic fundus during the newborn period include colobomata, optic disc hypoplasia or atrophy ( Fig. 18-9 ), retinal and preretinal hemorrhages, chorioretinitis, retinopathy of prematurity, and retinoblastoma. Congenital malformations associated with optic disc hypoplasia usually occur early during the first trimester of pregnancy (e.g., septo-optic dysplasia, agenesis of the corpus callosum). As many as 50% of affected infants subsequently exhibit other neurologic disorders. Atrophy suggests an acquired injury or ongoing metabolic or degenerative process. Retinal hemorrhages may suggest increased ocular venous pressure, blood dyscrasias, or asphyxia, but they can also be present in 20% to 40% of all newborns. Chorioretinitis may suggest a congenital infection, and retinopathy of prematurity is characterized by dilatation and tortuosity of vessels resulting from a variety of causes in premature infants. The uncommon presentation of retinoblastoma or an embryotoxon would be helpful in the diagnosis of a neoplasm or a genetic or metabolic disease, respectively. Retinoblastoma in neonates usually presents with a white pupil and strabismus. Embryotoxon signifies an arrest in development within the anterior chamber, which requires documentation with an indirect ophthalmoscope.

Pupils (II and III)
Pupillary function is associated with both the second and third cranial nerves and appears by 30 weeks’ gestational age, with consistent responses occurring between 30 and 32 weeks of gestation. Abnormalities in function of the pupillary pathways are reflected by changes in the overall size and symmetry of the pupils; bilateral cortical disease such as asphyxia and medication effects must be considered. Unilateral changes may imply autonomic dysfunction in central or peripheral nerve portions of the pupillary pathways. These can be associated with brachial plexus injuries, with herniation involving unilateral mass lesions (such as from infarction or intracranial hemorrhage), and with the mass effect of supratentorial brain structures along the course of the third cranial nerve in which the parasympathetic fibers are encased.
Extraocular Movements (III, IV, and VI)
Attention must be directed to the infant’s eye position, spontaneous eye movements, and movements elicited by oculovestibular maneuvers (i.e., doll’s eye reflex) or oculocaloric testing (i.e., cold or warm water response). Three cranial nerves interconnect within the brain stem to subserve these functions, and the doll’s eye reflex can be observed in the infant as early as 25 weeks’ gestational age. The eyes normally move conjugately in the direction opposite head movement, depending on the infant’s degree of prematurity. Caloric stimulation can be performed after 30 weeks, and spontaneous roving eye movements are expected after 32 weeks.
Abnormalities in extraocular movements include dysconjugate gaze, skew or downward deviation, and opsoclonus. These disorders of ocular motility can be seen in healthy neonates and usually resolve over the first 6 months of life, but they may indicate persistent gaze palsies related to ocular strabismus or intracranial diseases in the brain stem or cortical visual pathways. Intermittent abnormal eye movements may also be associated with seizure abnormalities. Some nonseizure pathologic processes associated with abnormal eye movements are hydrocephalus, mass lesions, and genetic and metabolic diseases.
Facial Sensation and Masticatory Power (V)
The trigeminal nerve has both sensory and motor components. Facial sensation is best assessed by eliciting facial grimaces to noxious stimuli. The three divisions of the trigeminal nerve must be distinguished from the first and second cervical root sensory distributions over the posterior scalp and neck, respectively. Masseter and pterygoid muscle strength also reflect fifth nerve motor function and may be affected if the neonate exhibits suck and swallow abnormalities. Corneal reflexes are also subserved by the autonomic pathway in the fifth cranial nerve, which becomes functional by the twenty-fifth to twenty-sixth week of gestation.
Facial Motility (VII)
Facial nerve function involves the amplitude and symmetry of both spontaneous and sensation-elicited facial movements. Major causes of facial weakness in the neonatal period arise at all levels of the neuraxis (i.e., cerebral, nuclear, peripheral nerve, neuromuscular junction, and muscle). For example, bilateral facial weakness may be secondary to severe hypoxic-ischemic encephalopathy, but bilateral or unilateral facial weakness may also be associated with brain stem hypoplasia or aplasia of the motor nuclear groups in a disorder known as Möbius syndrome. Injury to the facial nerve may also occur during labor or delivery as a result of compression of the face against the maternal sacrum or forceps compression. Finally, weakness at either the neuromuscular junction or the muscle may be associated with congenital myasthenia, myopathies, or mitochondrial disorders. Asymmetry of the infant’s face while crying may be associated with hypoplasia or absence of a specific muscle, the depressor angularis oris, on the side of the face that does not depress.
Auditory (VIII)
A startle reaction or blink in response to loud sudden noise is present at 28 weeks’ gestational age. The lemniscal or auditory pathway that subserves this sensory function is functionally active early in the third trimester of development. Auditory acuity, localization, and discrimination are present in the neonate. Detection of significant hearing deficits may be quite important in the examination of the neonate. Four categories of disorders causing nerve VIII disturbances are familial forms of deafness, bilirubin-induced injury to the auditory pathway, congenital infections, and congenital defects of the head and neck. Premature infants have a distinctly increased incidence of significant hearing loss. The full-term infant may experience hearing loss from asphyxia-related causes that occur any time during the perinatal period; for example, one specific pulmonary problem, persistent pulmonary hypertension of the newborn , may result in peripheral injury to nerve VIII. Measurement of the brain stem auditory evoked response may more precisely localize the site of the deficit to the peripheral nerve or auditory pathway.
Sucking and Swallowing (V, VII, IX, X, and XII)
Sucking involves coordinated action of breathing, sucking, and swallowing, with two coordinated phases that are linked in a synchronous action. The swallow response has a voluntary phase followed by an involuntary phase, both of which physiologically improve with maturity. Although rooting occurs as early as 28 weeks of gestation, the synchronous action of swallowing does not appear until 30 to 34 weeks and is not coordinated with breathing until 37 weeks. Gag reflexes subserved by cranial nerves IX and X are essential for the response of the posterior pharyngeal muscles, particularly to close the larynx and prevent aspiration; the gag reflex appears at 28 weeks’ gestational age. Disturbances affecting suck and swallow functions may originate throughout the neuraxis, ranging from the cerebral cortex through the brain stem nuclear groups to peripheral nerve, neuromuscular junction, and muscle levels.
Sternocleidomastoid Function (XI)
The function of the sternocleidomastoid muscle, which allows flexion and rotation of the neck and head, is mediated by cranial nerve XI. Disorders in this function are usually related to a contracture or weakness of the muscle, such as from fetal positioning or trauma to the muscle. There are also infants with congenital torticollis that reflects congenital, musculoskeletal, and brain abnormalities referable to the cervicomedullary junction. Malformations during the embryonic or fetal periods can result in specific anomalies. Careful inspection of the posterior occiput of the skull, neck, and scapulae should be integrated into consideration of cranial nerve XI function. Associated anomalies such as a deep posterior occipital shelf, torticollis, or elevated and internally rotated scapulae may be markers for Chiari malformation, Klippel-Feil syndrome, Sprengel deformity, syringobulbia, or basilar impression.
Tongue Function (XII)
Tongue movements are usually best assessed by observing spontaneous tongue movements or the infant’s suck on the examiner’s fingertip. Abnormalities in tongue function usually involve neurons of the hypoglossal nerve or muscle. Atrophy or fasciculations may suggest a pathologic condition in the brain stem nuclear group subserving tongue movement, ranging from congenital conditions (e.g., progressive spinal muscular atrophy) to destructive processes (e.g., brain stem infarction). The size of the tongue may imply syndromic or metabolic disorders (e.g., macroglossia with hypothyroidism, Beckwith-Wiedemann syndrome, or specific lysosomal storage diseases). The clinician should also consider that the tongue may appear enlarged because the oral cavity is reduced in size due to hypognathism (e.g., Pierre Robin syndrome).
Motor Examination
Major features of the motor examination of the newborn involve descriptions of muscle tone, posture of limbs, motility, muscular power, deep tendon reflexes, and plantar responses. As with all aspects of the neurologic examination, observations of motor function take into account gestational maturity, level of alertness, and findings of serial examinations. Medications, feeding, disease states, and presence of noxious stimuli must also be considered, because they affect the neonate’s motor responses.
Tone and Posture
Tone is best assessed by passively manipulating the limbs with the head in the midline. It is important to avoid head turning, which elicits a tonic neck reflex and gives a false sense of asymmetry in tone. Eighty percent of infants spontaneously prefer right-sided head turning. Developmental aspects of tone have been carefully documented by earlier researchers; in general, a caudal to rostral progression in tone development occurs with increasing gestational maturity. Minimal resistance is present at 28 weeks of gestation. By 32 weeks, distinct flexor tone begins in the lower extremities. By 36 weeks, flexor tone is prominent in the lower extremities and palpable in the upper extremities. When an infant’s level of maturity is assessed, observations of tone and posture imply measurement or observation of the angle of a limb around a joint, both at rest and with gentle traction.
The quantity, quality, and symmetry of motility and muscular power are also important aspects of the motor examination. The initial myoclonic movements of the preterm infant evolve into larger-amplitude, slower movements in the near-term and term infant. Also, as the infant matures, alternating rather than symmetric movements begin to be observed. By term, an awake infant has the ability to momentarily lift the head off the chest and hold it upright for several seconds while being supported in a vertical position.
Deep Tendon Reflexes and Plantar Responses
Deep tendon reflexes are initially elicited in the preterm infant after 32 to 34 weeks’ gestational age. Pectoralis, biceps, brachioradialis, knee, and ankle reflexes should be assessed. Symmetric ankle clonus of 5 to 10 beats may be an acceptable finding in a healthy full-term newborn. The plantar response is usually extensor in the newborn; therefore, it is assigned a pathologic significance only if asymmetry is noted.
In general, abnormalities found on motor examination include altered muscle tone with or without weakness, abnormalities in deep tendon reflexes and plantar responses, and abnormal spontaneous movements. A brief description of these abnormalities follows.
One must always consider normal maturational changes in motility, tone, and reflexes before ascribing pathologic significance. Therefore, the presence of low tone or hypotonia—the most common motor abnormality in neonates—must be considered with respect to possible patterns of muscle weakness that usually are present with low tone. Levels of the neuraxis that may be involved in hypotonia include focal or bilateral cerebral areas, brain stem, spinal cord, low motor neuron, nerve root, peripheral nerve, neuromuscular junction, and muscle ( Table 18-6 ). Focal injury to the cerebrum results in contralateral hemiparesis. Parasagittal cerebral injury, usually from asphyxia, affects the border zone vascular regions over the cerebral convexities, which results in weakness of the upper extremities more than the lower extremities. Periventricular injury, primarily located in deep white matter structures, largely affects tone and strength in the lower extremities. Spinal cord involvement usually spares the face and other functions of cranial nerves while involving sphincteric as well as motor and sensory functions below the site of the spinal lesion. Other lower motor neuron effects include primarily focal weakness situated in nerve roots or, more commonly, generalized weakness localized to the peripheral nerve, neuromuscular junction, or muscular levels.

Full access? Get Clinical Tree
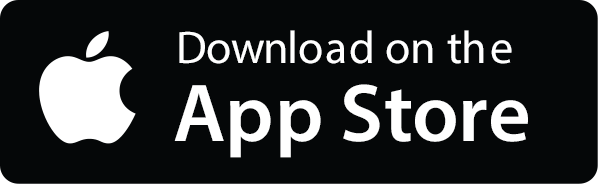
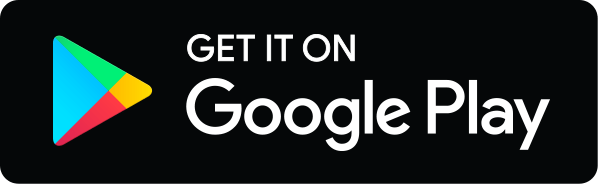