For many years, unconjugated bilirubin (UCB) was thought to be a useless waste product of heme catabolism, with no physiological function, but with potential toxicity. Toxicity resulting from unconjugated hyperbilirubinemia has been known for more than a century, since the landmark study of Schmorl describing autopsy findings from 120 jaundiced infants, as cited by Hansen.1
Interestingly, evidence obtained in the last decade revealed a beneficial role of the molecule. Indeed, physiological or modestly elevated serum levels of UCB have been shown to have a protective effect in several disorders, ironically even including neurodegenerative diseases.2 Protective effects of UCB rely on its antioxidant properties.
Above a certain threshold, toxic effects of UCB become evident. In this circumstance, prolonged exposure and accumulation of UCB can lead to reversible or irreversible cell damage, or even cell death.3 In fact, severe neonatal hyperbilirubinemia can lead to acute bilirubin encephalopathy (ABE) and kernicterus,4–6 underscoring the need for models to enhance our understanding of how hyperbilirubinemia causes permanent brain damage in some infants.
UCB binding to human serum albumin (HSA) provides UCB solubility and thus facilitates its transport to the liver. Decreased levels of HSA and lower capacity for UCB binding, usually found in neonates, especially in preterm babies, may contribute to higher tissue UCB levels. Under such conditions, there is an increase in the unbound (free) UCB (Bf) fraction, increased cellular uptake, and greater likelihood of bilirubin-induced neurological dysfunction (BIND).7–9 Bf is able to enter the cells by passive or facilitated diffusion and usually represents less than 0.1% of serum UCB.10
In neonates, serum UCB is usually elevated for the first 2 weeks of postnatal life due to increased breakdown of fetal erythrocytes, deficient HSA transport to the liver, and decreased conjugation.11 The central nervous system (CNS) is the most vulnerable site to UCB toxicity in the neonatal period, resulting in a wide array of neurological deficits collectively known as BIND.12
Despite the number of studies performed in patients and animal models, as well as in tissues and in different cellular systems, the molecular mechanisms underlying or contributing to UCB cytotoxicity are not completely understood. The disruption of several cellular functions rather than a single death-signaling pathway suggests that UCB toxicity is mediated by multifaceted mechanisms. On the other hand, there is difficulty in the extrapolation of in vitro observations to infants, namely because many studies are of doubtful relevance due to the use of nonphysiological concentrations of UCB, binding proteins, or improper experimental conditions.3
Experimental in vitro and in vivo models have been developed to study UCB toxicity and valuable data have been obtained by both. Nevertheless, each approach has its advantages and disadvantages. While animal models are believed to better mimic BIND, in vitro models are important to isolate specific pathways and mechanisms of injury.13
Monocultures of specific cell types are suitable for evaluation of acute toxicities, while co-cultures of different cell types and slices of explanted tissue that survive up to months may be used to assess chronic damage. Most important, the robustness of any finding, independent of the model used, will be enhanced when tested across other models. Combinations of both in vitro and in vivo models may then lead to a better understanding of the pathophysiology of BIND and, therefore, to effective preventive and therapeutic measures. However, a good, reproducible, integrated model that reproduces unconjugated hyperbilirubinemia and its acute toxicity and long-lasting sequelae is still needed.
In this section, we will address the strengths and weaknesses of different experimental BIND model systems that have been used to dissect the molecular mechanisms and to test the efficacy of potential drugs for prevention or treatment of BIND.
The principal hypotheses proposed to explain the mechanisms of BIND have been studied mostly in models in vitro, such as primary cell cultures or immortalized cell lines. In vitro systems offer ease of manipulation and are particularly suitable for dissecting interacting intracellular signaling cascades that ultimately lead to cell dysfunction and death. Nevertheless, pure neuronal cultures do not fully take into account the interplay between neurons and glia. Therefore, a growing number of studies have been conducted over the past two decades on the development and optimization of acute and organotypic brain slice models, which allow the realistic interaction of neurons with glia.
Cell lines are the less complex systems and are easy to obtain, but often are derived from tumors or from transformed cells, and hence resemble less the in vivo circumstance. As such, neuroblastoma cell lines often display morphological, developmental, and signaling characteristics that differ from isolated neurons.14 Advantages of using cell lines reside in the unlimited number of cells available for studies and the guarantee of a single cell type. Cell lines have also significant disadvantages. Because they have a high proliferation rate, they retain some degree of plasticity, and their properties can change with the number of passages over time.15 The mouse hepatoma Hepa 1c1c7,16 human neuroblastoma SH-SY5Y,17,18 NT2-N neurons,19 and murine lymphoma L5178Y-R20 cell lines were used in the last 5 years to study the cytotoxic effects of UCB.
Dissociated primary cell cultures are also a simplified model that allows the evaluation of cell response, reactivity, and demise on UCB exposure in a very pure and particular cell type. Thus, a major advantage of this culture technique relates to the possible evaluation of how different cell types respond to a UCB stimulus. Interestingly, it seems that the development of neural cells in vivo corresponds to their development in vitro, thus allowing the study of age-related vulnerabilities to UCB.21 Another benefit, in contrast to cell lines, is that the neural cells used for the cultures, derived from late embryonic (E18 for neurons) or early postnatal days (1–2 days for glial cells), are quite immature and better represent the conditions that might be associated with neonatal jaundice. However, if the cells are maintained in vitro, adult cell characteristics will be expressed. Another interesting aspect of such culture systems is the possibility to study regional susceptibilities to UCB when isolating cells from different brain regions, such as the cortex, hippocampus, or cerebellum. Primary cultures of dissociated neurons are the most commonly used in vitro preparations to evaluate UCB-induced neurodegeneration22,23 and for testing compounds with either protective24–26 or detrimental27 effects.
Co-cultures, where neurons are cultivated on the top of astrocytes or the glial cells seeded onto culture plates with neurons, produce more stable cultures and provide the means to directly evaluate the cross-talk between two different cell types.
Compared with the culture of dissociated neuronal cells, organotypic cultures made from slices of explanted tissue (e.g., hippocampus) represent a complex multicellular in vitro environment and have the advantage of preserving in vivo cell-to-cell interactions.28 Fresh (acute) slice preparations are used mostly for electrophysiological measurements. Organotypic slice preparations are maintained in vitro for weeks to months and routinely used to evaluate the pathophysiology of neuronal dysfunction and to screen novel therapies, replicating many aspects of the in vivo system. For review, the reader is referred to Lein et al.29 Slices are obtained from brains of neonatal pups at postnatal days 3–9 (P3–P9) and chopped into 400-μm thick sections.30,31 Advantages to be considered over animal models are the easy access, the control of the extracellular environment, and the possibility to evaluate both short- and long-term alterations.31
Recently, Chang et al.,32 by using rat organotypic hippocampal slice cultures, have shown that 24-hour incubations with UCB impairs long-term synaptic plasticity. Presynaptic damage by UCB was later observed in acute brain slices of the auditory brainstem from P16–P18 Gunn rats at 18 hours after intraperitoneal (IP) administration of sulfadimethoxine.33 These findings add to the understanding of UCB-induced deafness and temporary or permanent impairment of mental function by hyperbilirubinemia.
Given the complexities of living organisms, in vivo animal models of BIND would appear to offer the best systems to evaluate the pathophysiological mechanisms of UCB neurotoxicity and for testing therapeutic strategies, provided that they closely mimic the symptoms and temporal progression of UCB encephalopathy in human neonates. The existence of redundant compensatory and adaptive systems in transgenic mouse models may limit their usefulness as the sole approach to test specific biochemical hypotheses.
The Gunn rat, a mutant jaundiced rat of the Wistar rat strain, has provided the opportunity to study kernicterus and the mechanisms that underlie BIND. Jaundice in the Gunn rat derives from the rat’s inability to conjugate bilirubin and, like the human Crigler–Najjar Syndrome Type I, results from a mutation in the UDP-glucuronosyltransferase (UGT) 1A1 gene. Nonetheless, hyperbilirubinemia in the Gunn rat is milder (˜7 mg/dL)34 and usually not severe enough by itself to cause kernicterus or to produce neonatal morbidity. Therefore, to trigger a sudden elevation of the Bf fraction and produce UCB encephalopathy, either sulfadimethoxine9 (a displacer of UCB from albumin) or phenylhydrazine35 (an inducer of hemolysis) has been used. A limitation of the model resides in the occurrence of maximal hyperbilirubinemia only at P16, instead of the particularly vulnerable first week in human newborns.36 Nonetheless, this model is without doubt the most used by several authors to evaluate mechanisms that mediate BIND,37,38 regional brain susceptibilities to UCB,39 hearing loss,33,40 and neuroprotective therapeutic approaches to BIND.26,41–45
The Ugt1-null mouse phenotype34 mimics the irreversible damage produced by UCB in severely ill infants and in Crigler–Najjar Type I patients, leading to kernicterus. Jaundice in the range of 7–10 mg/dL at P5 is present as a phenotypical orange skin color in the first 8 hours of postnatal life and all Ugt1-null mice develop kernicterus and die within 11 days following birth. Although only showing a difference of 2 mg/dL in total serum bilirubin (TSB) from that observed in the Gunn rat, its earlier presentation seems to be sufficient to initiate CNS toxicity and lethal encephalopathy in mice neonates. Nevertheless, experiments to examine the impact of BIND in these mice have not yet been performed.
Mild to moderate hyperbilirubinemia is observed in another form of inheritable condition known as Gilbert syndrome46 associated, but not exclusively, with an additional TA insertion into the UGT1A1 promoter, generating the UGT1A1*28 allele instead of the normal UGT1A1*1 allele.47 The generation of humanized UGT1 mice expressing the UGT1A1*28 allele (hUGT1A1*28)36 may represent a better model to evaluate the mechanisms leading to more subtle CNS abnormalities. These mice have TSB levels that may exceed 15 mg/dL at P7–P14 and 10% of these animals show CNS toxicity, seizures, and death. In survivors, TSB concentrations decrease to 0.8–1.2 mg/dL at P14–P21. It seems that resistance to BIND is developed as mice mature and become capable of combating the impact of high levels of UCB. The hUGT1A1*28 mouse model offers an interesting approach to establish valid in vivo correlations between cellular alterations and harmful outcomes.
UCB has been widely reported as a beneficial molecule mainly due to its antioxidant properties at low nanomolar concentrations of Bf. Elevated levels of UCB and Bf, however, can have harmful pro-oxidant effects as well, under some conditions.
Studies dealing with the mechanisms of UCB toxicity should use UCB to HSA molar ratios (UCB:HSA) or Bf levels considered clinically relevant.48 To experimentally evaluate UCB toxicity, incubations should be prepared with a molar excess of albumin over UCB to obtain stable solutions and avoid aggregation. The most frequently used UCB:HSA are between 0.5 and 1.0.32,49 An alternative to this is to work with a concentration of Bf equal to or slightly higher than 70 nM,50–52 which is the limit of bilirubin aqueous solubility in the absence of albumin.53 Nevertheless, Bf values below or equal to 1000 nM (1 μM) are still considered clinically relevant.54 Neurotoxic effects of even purified UCB can be observed at Bf levels ranging from 71 to 770 nM in in vitro studies.55 Similar values by calculated Bf were obtained in Gunn rats treated with sulfadimethoxine56 and a considerable overlap was observed between those and the predicted values in human neonates with a TSB of 35 mg/dL.9
UCB is unstable and readily oxidized when exposed to extreme pH values or to even traces of oxygen.57,58 The rate of UCB decomposition increases with exposure to light.59 Thus, experiments should be performed under light-limited conditions to avoid or minimize photodegradation,58,60 as these photoproducts may be less toxic than UCB.61
General cellular injury by UCB appears to start at the cell membrane, considered one of the first, if not the primary or the initiating, targets of UCB toxicity,62 which is followed by interaction with the intracellular membranes that surround various organelles. First studies in erythrocytes exposed to UCB pointed to morphological alterations and perturbation in phospholipid distribution with the translocation of the inner aminophospholipid phosphatidylserine to the outer leaflet of the membrane.63 This feature is a sign of cell senescence and is recognized by macrophages. Interestingly, in a recent study on the effects of UCB in immature neurons, increased apoptosis based on the interaction between annexin V and phosphatidylserine was observed,64 thus supporting this finding.
Damage to membranes increases with the UCB concentration, bilirubin-displacing drugs, time of exposure, and acidic pH. Inhibition of cell endocytosis and glutamate uptake, alterations in membrane potential, inefficiency of membrane receptors, as well as transporters and enzymes are some of the toxic effects produced by UCB at the membrane level.11,65 Most of these effects culminate in cell death and may lead to hemolysis of erythrocytes, thus contributing to the production of UCB.63 Although in vitro studies have shown that increases in heme catabolism directly relate to the hyperbilirubinemia observed in the first 4 days after birth66 and that increased photohemolysis occurs in spherocytes treated with UCB,67 stronger evidence is needed to support its clinical significance.68
Direct effects of UCB in synaptosomal membrane systems have been observed through the production of reactive oxygen species (ROS), protein oxidation, and lipid peroxidation,69 which were later confirmed to also occur in other cells, mainly in neurons.25,70 Reduced activity of membrane-bound Na+/K+-ATPase and Mg2+-ATPase (aminophospholipid translocase, flippase), which selectively pumps the phosphatidylserine from the outer to the inner monolayer, also occurred in neocortical synaptosomes.69
Studies by electron paramagnetic resonance spectroscopy have indicated that UCB intercalates superficially in the C-5 hydrocarbon of the membrane bilayer, disrupting protein order and rendering inner regions more fluid and more permeable to water diffusion, with a maximum expression at the C-7 level.11,71
Membrane dynamic properties may be changed by UCB but do not appear to be a significant obstacle to the entry of UCB into cells. In fact, UCB passage across cellular membranes appears to involve passive diffusion through the hydrophobic lipid core.72 Therefore, UCB effects have been observed in several intracellular organelles.
Although it is not known whether the cell membranes or mitochondria are the primary target for the toxic effects of UCB, mitochondria are particularly vulnerable. Similar to the erythrocyte membrane, it seems that UCB is preferentially accommodated at the C-5 level in mitochondrial membranes, despite having the maximum effect at the C-7 level, indicating that perturbation of membrane dynamics by UCB has common features regardless of the type of membrane model.11 Physical perturbation of mitochondrial membrane results from protein order disruption, oxidative injury, mitochondria swelling, and release of cytochrome c. This latter event and the activation of caspase-9 and -3 (Figure 7-1) further suggest that UCB-induced apoptosis is mediated at least in part by mitochondria.53 Inhibition of cytochrome c oxidase (complex IV), the terminal component of the mitochondrial respiratory chain, by UCB was verified in brain and liver mitochondria,50 as well as in immature neurons.64 Reductions in the rates of oxygen consumption and inner mitochondrial membrane potential further compromise cell viability.
Figure 7-1.

Schematic drawing of intracellular pathways and subcellular compartments believed to be involved in cell damage by unconjugated bilirubin (UCB). UCB causes mitochondrial dysfunction with collapse of inner mitochondrial membrane potential (Δψm), a diminished activity of complex IV (cytochrome c oxidase), and the release of cytochrome c to the cytosol, where it activates caspase-9, which in turn activates caspase-3, triggering the initiation of apoptosis (mitochondrial intrinsic pathway). UCB can also induce apoptosis by the extrinsic pathway, whereby activation of caspase-8 is involved. The caspase-8 signal may be enhanced by the cleavage of BH3-only protein (Bid) into the truncated form (tBid) that is translocated to mitochondria, thus propagating the apoptotic signal. Another relevant mechanism of cell damage involves endoplasmic reticulum (ER) stress, which leads to an intracellular overload of Ca2+ and interferes with Ca2+-binding proteins, as calpain. In addition, it leads to the dissociation of an ER luminal chaperone, the immunoglobulin heavy chain binding protein (Bip), as well as to the activation of caspase-2 and -12, which also trigger apoptosis. While caspase-12 is activated by caspase-3 and, in turn, activates caspase-9, caspase-2 cleaves Bid into tBid. Production of reactive oxygen species (ROS) and reactive nitrogen species (RNS) appears as a common denominator in the mechanisms of cell death, being related to both mitochondrial disruption and ER stress. Oxidation of cellular components interferes with cytoskeleton proteins, as α-spectrin, thus disrupting its normal assembly. Moreover, oxidation of nucleic acid bases compromises DNA integrity, leading to its fragmentation. All these events can culminate in cell death by both apoptosis and necrosis.
The endoplasmic reticulum (ER) regulates protein synthesis, protein folding and trafficking, cellular responses to stress, and intracellular calcium (iCa2+) levels. Alterations of the Golgi complex by UCB include the presence of enlarged vacuoles and increased vesicles in animals with kernicterus.73 Hypertrophy and hyperplasia of the ER with unusual prominences of the Golgi apparatus were noticed in the liver of Crigler–Najjar Type II patients, a less severe syndrome than the Type I.74 Similar ER alterations and mitochondria abnormalities were induced in astrocytes after exposure to UCB.75 Accumulation of UCB within the cell provokes ER stress76 and many related genes have been shown to be upregulated by UCB.16 ER stress can either initiate or contribute to apoptotic cell death77 by the ER-resident caspase-2 and -12 (Figure 7-1). While caspase-2 was identified as the premitochondrial protease,78 caspase-12 was shown to be activated by calpain and caspase-3, thus activating caspase-9.79 Cleavage of proapoptotic BH3-only protein (Bid) into truncated Bid (tBid) may occur either via caspase-2 or via calpain and caspase-8 activation,80 and immunoglobulin heavy chain binding protein (Bip) derives from ER stress.81 Activation of caspase-8 and cleavage of Bid into tBid by UCB in immature cortical neurons23 and the decrease in the caspase-12 pro-form in Hepa 1c1c7 cells16 corroborate the existence of a mitochondrial- and ER-stress-mediated apoptotic pathway. Upregulated BiP in SH-SY5Y cells further reflects an ER stress response to UCB.76 Some UCB-induced genes also suggest a role for autophagy, which is believed to be implicated in cell growth inhibition.82,83
UCB-induced cell demise is in part due to oxidative stress, which causes a signal blockade on cell cycle progression and leads to subsequent DNA damage.17 Interestingly, cell cycle arrest was shown to be induced by UCB through activation of extracellular signal-regulated kinases 1 and 2 (ERK1/2) in colon cancer,84 and genes linked to cell cycle dependence are repressed in Ugt-null mice.34 High TSB levels were considered to be genotoxic in an in vivo study performed with jaundiced newborns.85 In accordance, autopsy analysis of cases of BIND revealed immunostaining for markers of DNA oxidation.86 This DNA oxidation causes S-phase progression delay and triggers apoptosis,17 which is recognized when cells show condensed and fragmented nuclei (Figure 7-2), thus revealing DNA as a potential target of UCB toxicity. Considering the potential carcinogenic and mutagenic consequences of DNA strand breaks,87 it can be hypothesized that potential side effects of a carcinogenic or genetic nature may ensue later in life, as a result of DNA damage caused by neonatal hyperbilirubinemia.
Figure 7-2.

Morphological assessment of cell death by apoptosis in astrocytes incubated with unconjugated bilirubin (UCB). Astrocytes were isolated from brain cortices of 2-day-old rats and cultured for 10 days in vitro. Cells were incubated with 100 μM human serum albumin (HSA) in the absence (control) or in the presence of 50 μM UCB. Coverslips with adherent astrocytes were collected after 4 hours of incubation and then washed. Cells were fixed with 4% paraformaldehyde and stained with Hoechst 33258 dye. Alterations in nuclei morphology were observed by fluorescence microscopy. Apoptotic cells show condensed chromatin uniformly stained (stronger blue fluorescence nuclei, arrow) and nuclear fragmentation (bright fluorescent spherical beads, arrowhead). Scale bar equals 25 μm.
High concentrations of UCB disrupt several cell functions, not only via a single cell-death pathway but also involving effects in different intracellular organelles as noted above. Despite decades of study, the determinants of damage, vulnerability, and reversibility, as well as the underlying mechanisms of BIND, still remain unclear. Icteric brain sections have revealed that UCB accumulates within neurons, neuronal processes, and microglia,88 but cell-dependent sensitivity to UCB toxicity and the role of each cell type are still not fully understood. In vitro assays have shown that UCB leads to oxidative stress, exerts immunomodulatory effects, determines an increase in iCa2+, induces the release of glutamate, and leads to cell death. Structural–functional relationship studies might help in the understanding of the pathological processes and developmental difficulties experienced by jaundiced babies.
The brain is particularly vulnerable to ROS, especially the developing brain,89 and low antioxidant defenses will favor mitochondria senescence and cell demise. Increased production of ROS, protein oxidation, lipid peroxidation, and cell death in neurons, as compared with astrocytes, may be due to the reduced stores of total glutathione.70 Recently, it was demonstrated that UCB determines glutathione depletion in neurons,90 as well as in splenocytes,91 by decreasing the reduced glutathione (GSH) and increasing the oxidized disulfide form (GSSG).
Although the precise molecular mechanisms by which UCB exerts toxicity remain unknown, generation of ROS is surely involved and an initial event in the UCB-mediated cell death in neurons.70,90 Increased production of superoxide radical anion has also been observed with the exposure of neutrophils to UCB.92 Thus, N–acetylcysteine (NAC), a glutathione precursor, N-ω-nitro-l–arginine methyl ester hydrochloride (l-NAME) that prevents the formation of excessive amounts of nitric oxide (NO) by inhibiting the NO synthase (NOS), and glycoursodeoxycholic acid (GUDCA),25 a bile acid with antiapoptotic, anti-inflammatory, and antioxidant properties, have been shown to counteract the disruption of the redox status by UCB in neurons.
Production of lipid peroxides, hydrogen peroxide, and hydroxyl radical, as well as a decrease in glutathione content and reduction of the mitochondrial membrane potential by UCB, was also shown to induce caspase-3 activation and apoptosis.93 Recently, it has been demonstrated that UCB differentially regulates several genes and determines a consistent downregulation of several antioxidant enzymes.16 Thus, it is not surprising that the increased production of ROS by UCB has been observed in HeLa cells, mouse embryonic fibroblasts, astrocytes, and neurons, among other cells.25,51,70 The ability of UCB to upregulate DJ-1,17 a redox-sensitive chaperone,94 was identified as a cell defense mechanism in the human neuroblastoma cell line. Although similar results were achieved in neurons acutely exposed to UCB, increased incubation times effected the downregulation of DJ-1,90 appearing to negatively influence the adaptive cell response mechanism of neuroprotection.
Signaling cascades initiated or regulated by iCa2+, ROS, and reactive nitrogen species (RNS) are essential to diverse physiological and pathological processes. Mitochondria are central to energy metabolism and a hub for Ca2+, which is in a delicate balance with ROS generated by mitochondria and ER; yet iCa2+ and ROS overload can lead to oxidative stress, mitochondrial dysfunction, and cell death.95 Elevated iCa2+ and ROS production were obtained in synaptosomes treated with UCB, which may in turn engender more production of ROS (Figure 7-1).11 Increased iCa2+ perturbs intracellular homeostasis and may cause ER stress. In a hyperbilirubinemic newborn mouse model, it was found that UCB-induced neurotoxicity was associated with augmented iCa2+ concentrations and activation of caspase-3 in the brain.96 Autopsy analysis of several cases of BIND revealed a decrease in the immunoreactivity for Ca2+-binding proteins,86 which are implicated in Ca2+ homeostasis.97 Furthermore, downregulation of these proteins was observed after treatment of Hepa 1c1c7 cells with UCB,16 thus leading to elevated iCa2+. Therefore, it is not surprising that taurine has been shown to limit BIND by inhibiting iCa2+ overload.96
Several studies suggest that UCB possesses multiple biological activities, which differ accordingly with the use of low/normal levels or, in opposite, of pathophysiological concentrations of UCB. Therefore, when, low concentrations of UCB having antioxidant properties are assayed, inhibition of transendothelial migration of murine splenic lymphocytes across endothelial cells is observed. These levels of UCB98 block the vascular cell adhesion molecule-1 (VCAM-1) signaling and the consequent activation of the matrix metalloproteinases (MMPs)-2 and -9 as well as suppress Th2 cytokine-mediated airway inflammation in response to an allergen challenge. In contrast, higher concentrations of UCB have been shown to be toxic to murine splenocytes and human peripheral blood mononuclear cells.91 UCB was shown to arrest the cell cycle at a pre-G1 phase and cause DNA fragmentation and caspase-3 activation in splenocytes. UCB-induced apoptosis was observed to involve either the extrinsic (upregulation of CD95 expression and increase in caspase-8) or the intrinsic apoptotic pathways (activated Bax, caspase-9 activation, increased cytoplasmic calcium, and loss of MMPs) in these cells (Figure 7-1). Similar to what has been previously obtained in primary cultures of rat cortical astrocytes,99 p38 mitogen-activated protein kinase (MAPK) activation was also demonstrated to have a key role in UCB-induced apoptosis and be related to cell depletion of glutathione.91 More important, p38 MAPK activation was associated with alterations produced by UCB in the host immune response in vivo. In fact, IP injection of UCB (25–50 mg/kg body weight [BW]) to mice triggered a significant decrease in the number of erythrocytes and leukocytes, including variations in the percentage of lymphocytes and monocytes, hemoglobin levels, spleen weight, and viability of spleen and bone marrow cells, which was attenuated by the administration of SB203580, a p38 MAPK inhibitor. This is not without precedent since MAPK transduction pathways have been identified as key players in the UCB-induced secretion of proinflammatory cytokines, such as tumor necrosis factor-α (TNF-α) and interleukin (IL)-1β and -6, as well as in cell death of UCB-treated astrocytes.99,100 The activation of the nuclear factor-kappa B (NF-κB) signal transduction pathway, one of the most important regulators of inflammatory gene expression mediating the synthesis of cytokines, may also contribute to the inflammatory response.101,102
Recent work by Loftspring et al.92,103 established that UCB is associated with increased edema, neutrophil infiltration, and intercellular adhesion molecule-1 (ICAM-1) expression, as well as with perihematomal neutrophils in an intracerebral hemorrhage (ICH) mouse model. This interacting cascade of chemical, cellular, and cytokine events may result from the degradation of heme to UCB and UCB-derived oxidative products, which further may activate the release of cytokines by microglia and astrocytes, and determine the potent and delayed inflammatory response that follows ICH. In vitro studies with microglia have shown that the initial activation by UCB is followed by a later cell death.104,105 Therefore, the reduced perihematomal microglial-immunoreactivity observed in the ICH mouse model92 may be explained on the basis of a hyperacute microglial activation ending in cell senescence.
Glutamate is the primary excitatory amino acid neurotransmitter in the CNS and a potent neurotoxin that may lead to the death of nerve cells. Extracellular accumulation of glutamate overstimulates glutamatergic receptors and increases the production of reactive and excitotoxic oxygen/nitrogen species triggering oxidative/nitrosative stress and neuronal death. Glutamate transporters, which are mainly expressed in astroglial cells, are responsible for 90% of total glutamate uptake.106 When astrocytes were incubated with UCB, accumulation of extracellular glutamate was associated with a decreased uptake and an increased and fast efflux from astrocytes.71 Release of glutamate by neurons, although lower than that produced by UCB in microglia or astrocytes, was also significant107 and may play a role in the mediation of neurotoxicity by UCB. The protective effect of l–carnitine may involve inhibition of the glutamate and/or N-methyl-d-aspartate (NMDA) receptor, preventing Ca2+ influx and causing an antioxidant effect.108
Overstimulation of NMDA receptors was initially indicated to be involved in UCB-induced apoptosis in developing rat brain neurons109 and later to be associated with increased neuronal NOS (nNOS).110 When the NMDA receptor antagonist MK-801 was used, neuronal apoptosis,111 nNOS expression, NO release, and 3′,5′-cyclic guanosine monophosphate (cGMP) production, as well as cell dysfunction and demise, were abrogated.110 Intriguingly, other studies showed that MK-801 was unable to protect neuronal viability in the presence of UCB or from abnormalities in brainstem auditory-evoked potentials (BAEPs) in Gunn rat pups.37 Nevertheless, further data obtained by gramicidin-perforated patch clamp techniques indicated that UCB facilitates presynaptic glutamate release and activates both α-amino-3-hydroxy-5-methyl-4-isoxazole propionic acid (AMPA) and NMDA receptors to produce neuronal hyperexcitation.112
The blood–brain and the intestinal barriers are dynamic and complex interfaces that strictly control and provide protection against many toxic compounds and pathogens. Epithelial permeability and polarization are determined by the apical junctional complex consisting of tight junctions (TJ) and adherens junctions, and inflammatory conditions are known to compromise the barrier function.
UCB was shown to increase the paracellular permeability in Caco-2 monolayers, widely used to mimic the intestinal epithelia, by triggering the redistribution of the TJ protein occludin.113 Disruption of intestinal integrity by UCB was shown to also involve disassembly of other TJ proteins, such as claudins and zonula occludens.114 Increased intestinal permeability by hyperbilirubinemia was also observed in neonates on the third day of life.115 Similar to the Caco-2 monolayers,113 loss of cell viability caused by UCB was observed in confluent monolayers of human brain microvascular endothelial cells, together with an increase in caspase-3 activation. Elevated levels of UCB and longer exposure times determined the release of IL-6, IL-8, and vascular endothelial growth factor, as well as the disruption of the redox status, which may contribute to the observed increased permeability.49 This deleterious action by high levels of UCB in the cerebral microvascular integrity may act synergistically with cytokines in facilitating immune cell trafficking into the CNS.
Newborns are particularly susceptible to BIND, especially if born prematurely, probably related to a greater susceptibility to toxic stimuli. This is why most of the cases of kernicterus, still reported today in the literature, have occurred in premature infants with or without associated sepsis and/or glucose-6-phosphate dehydrogenase deficiency.6,116,117
The primary concern with respect to unconjugated hyperbilirubinemia is its potential for neurotoxic effects causing irreversible and/or long-lasting neurological sequelae. Response to insult will depend on the cell type, intensity and duration of insult, neurodevelopmental maturity differences, brain regional vulnerabilities, and interactions between cells in the tissue.
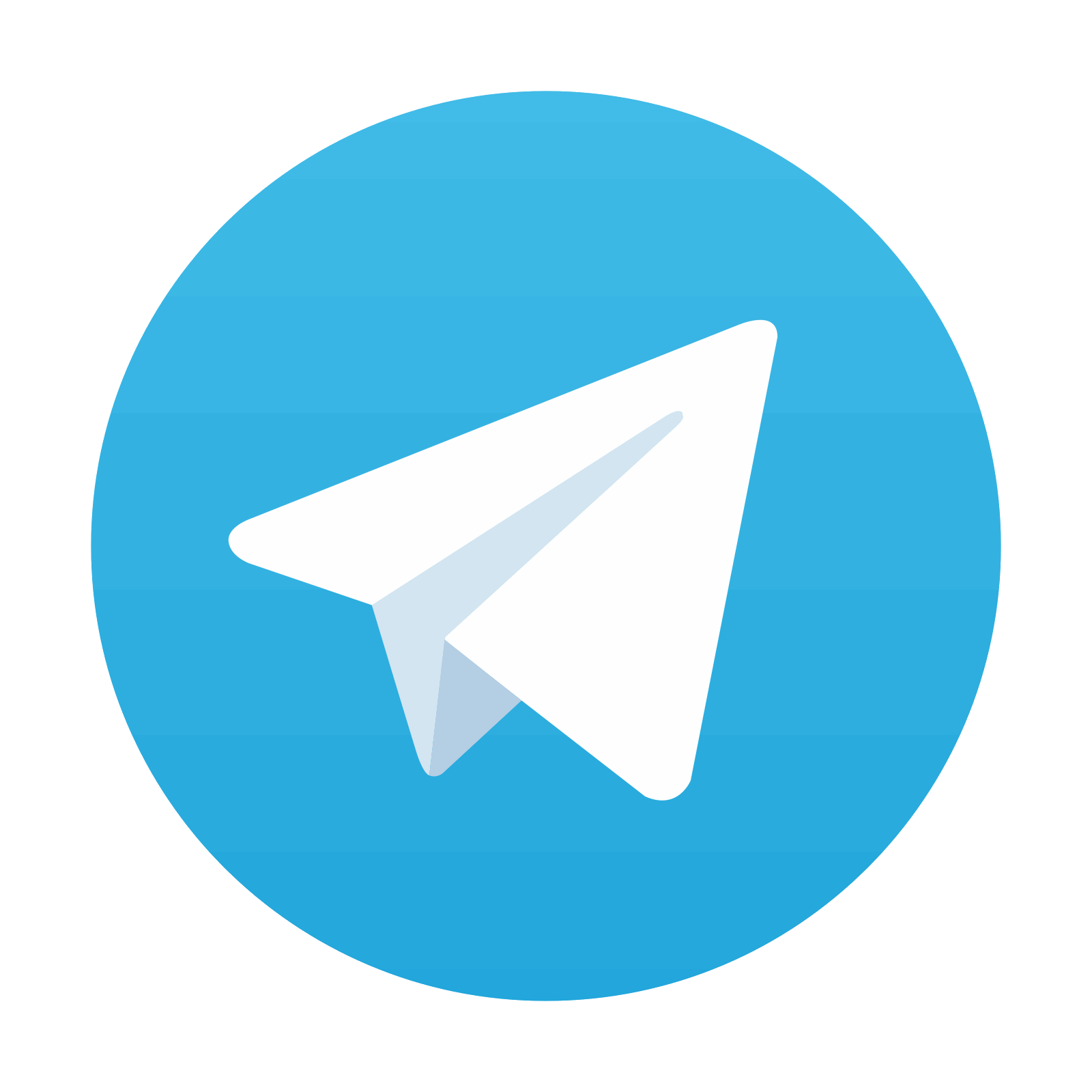
Stay updated, free articles. Join our Telegram channel

Full access? Get Clinical Tree
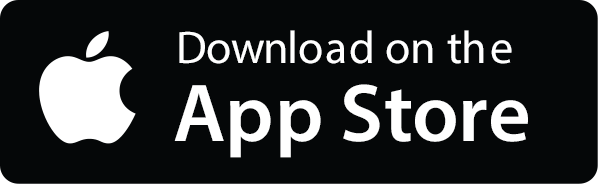
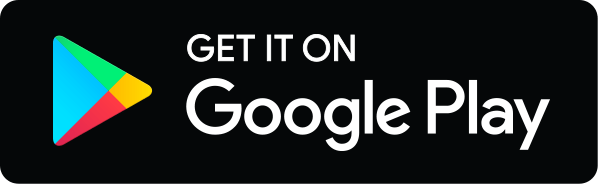