An understanding of synaptic neurotransmission is fundamental to the understanding of various neuropsychiatric symptoms and disorders. It is also essential to the discovery of pharmacologic agents that modulate neurotransmission to alleviate such symptoms and conditions. Various aspects of the process of neurotransmission and the synthesis, release, reuptake, or destruction are all potential events for action of therapeutic drugs. This article reviews the basic aspects of relevant neuroanatomy, neurotransmission, and major neurotransmitter systems.
The neuron
The main components of a neuron or nerve cell are the cell body or soma, an axon, and the dendrites ( Fig. 1 ). Each neuron is enclosed in a neuronal membrane. The nucleus, the rough endoplasmic reticulum, the smooth endoplasmic reticulum, the Golgi apparatus, and the mitochondria are contained within the cell body ( Fig. 2 ). The ribosomes on the rough endoplasmic reticulum and the free ribosomes are the major sites for protein synthesis directed by the messenger ribonucleic acid (mRNA). The function of smooth endoplasmic reticulum varies depending on its location, and includes its role in finessing the protein structure and regulating concentration of certain intracellular chemicals. The Golgi apparatus plays a major role in chemical processing of the proteins. The mitochondrion is the site of cellular respiration and provides the chemical energy for the intracellular biochemical reactions. The neuronal cell membrane is a dynamic complex structure that wraps over the cytoskeleton of the cell consisting of microtubules, microfilaments, and neurofilaments. Specific genes are located on the deoxyribonucleic acid (DNA) in each chromosome inside the nucleus.


In addition to the structures of the cell body, the neuron is uniquely characterized by the axonal and dendritic processes. Each axon has 3 main parts, namely the axon hillock, the axon proper, and the axon terminal. The point of contact between the presynaptic axon terminal and the postsynaptic dendrite is called the synapse ( Fig. 3 ). The axon terminal may also end on the cell body of the postsynaptic neuron. The neurotransmitter is stored in the synaptic vesicles in the axon terminal. Axons carry the neuronal impulse to various other neurons in the brain. The information or input is carried to the cell body of the receiving neuron. On the receiving end, the information or impulse is received at the dendrites.

The synapse
At the synapse the electrical impulse from the presynaptic neuron is converted to a chemical signal that is carried to the postsynaptic receptors, where it is again converted to an electrical impulse in the postsynaptic neuron. Synaptic plasticity is an important characteristic of neurons that allows for experience dependent adaptation and learning.
There are 2 types of neuronal synapses: electrical and chemical. The electrical synapses are located at gap junctions and allow free bidirectional movement of ions across cell membranes. Their function in the brain varies at different sites; they provide for fast transmission of impulses. Most of the synaptic transmission in the brain occurs at the chemical synapses. Autoreceptors are membrane-bound receptors on the presynaptic membrane, which regulate the release and in some cases synthesis of the intrinsic neurotransmitter. Certain other receptors on the presynaptic membrane can be modulated by neurotransmitters from nearby neurons and are called heteroreceptors.
The membrane-bound postsynaptic neuroreceptors can generally be classified into 2 types: (1) Transmitter-gated (or ligand-gated) ion channels, and (2) G-protein–coupled receptors. Transmitter- or ligand-gated ion channel receptor consists of 5 subunits that are arranged as columns in a circle, thus forming a pore or a channel in the middle. The neurotransmitter binds to the specific site on the extracellular portion of the receptor, leading to opening of the channel. The effect on the postsynaptic neuron depends on which ion is preferentially passed through the channel into the postsynaptic neuron. Movement of sodium ions into the postsynaptic neuron results in depolarization of the postsynaptic cell and generation of excitatory postsynaptic potential, as seen in glutamatergic neurotransmission. On the other hand, increased permeability to chloride ions will lead to hyperpolarization of the postsynaptic cell and generation of inhibitory postsynaptic potential, as seen in γ-aminobutyric acid (GABA)-ergic neurotransmission. The neurotransmission at the ligand gated ion channels is relatively faster compared with that at the G-protein–coupled receptors.
In the case of G-protein–coupled receptors, the occupancy of the extracellular receptor site by the neurotransmitter results in activation of intracellular receptor linked G proteins ( Fig. 4 ). The activated G proteins turn on the effector proteins, which in turn activates the G-protein gated ion channels in the postsynaptic neuronal membrane or activates enzymes (such as adenylyl cyclase and phospholipase C) to form intracellular second messengers. Second messengers then lead to a biochemical cascade, eventually communicating the message to the DNA of the postsynaptic neuron. Because of their metabolic effects, the G-protein receptors are also known as metabotropic receptors.
The synapse
At the synapse the electrical impulse from the presynaptic neuron is converted to a chemical signal that is carried to the postsynaptic receptors, where it is again converted to an electrical impulse in the postsynaptic neuron. Synaptic plasticity is an important characteristic of neurons that allows for experience dependent adaptation and learning.
There are 2 types of neuronal synapses: electrical and chemical. The electrical synapses are located at gap junctions and allow free bidirectional movement of ions across cell membranes. Their function in the brain varies at different sites; they provide for fast transmission of impulses. Most of the synaptic transmission in the brain occurs at the chemical synapses. Autoreceptors are membrane-bound receptors on the presynaptic membrane, which regulate the release and in some cases synthesis of the intrinsic neurotransmitter. Certain other receptors on the presynaptic membrane can be modulated by neurotransmitters from nearby neurons and are called heteroreceptors.
The membrane-bound postsynaptic neuroreceptors can generally be classified into 2 types: (1) Transmitter-gated (or ligand-gated) ion channels, and (2) G-protein–coupled receptors. Transmitter- or ligand-gated ion channel receptor consists of 5 subunits that are arranged as columns in a circle, thus forming a pore or a channel in the middle. The neurotransmitter binds to the specific site on the extracellular portion of the receptor, leading to opening of the channel. The effect on the postsynaptic neuron depends on which ion is preferentially passed through the channel into the postsynaptic neuron. Movement of sodium ions into the postsynaptic neuron results in depolarization of the postsynaptic cell and generation of excitatory postsynaptic potential, as seen in glutamatergic neurotransmission. On the other hand, increased permeability to chloride ions will lead to hyperpolarization of the postsynaptic cell and generation of inhibitory postsynaptic potential, as seen in γ-aminobutyric acid (GABA)-ergic neurotransmission. The neurotransmission at the ligand gated ion channels is relatively faster compared with that at the G-protein–coupled receptors.
In the case of G-protein–coupled receptors, the occupancy of the extracellular receptor site by the neurotransmitter results in activation of intracellular receptor linked G proteins ( Fig. 4 ). The activated G proteins turn on the effector proteins, which in turn activates the G-protein gated ion channels in the postsynaptic neuronal membrane or activates enzymes (such as adenylyl cyclase and phospholipase C) to form intracellular second messengers. Second messengers then lead to a biochemical cascade, eventually communicating the message to the DNA of the postsynaptic neuron. Because of their metabolic effects, the G-protein receptors are also known as metabotropic receptors.
Chemical neurotransmission
Chemical neurotransmission is mediated by specific neurotransmitters in the brain, and a large number of these have been identified. There are various classes of neurotransmitters in the central nervous system (CNS); amine and monoamines are of interest in a review of psychopharmacology. Other classes of neurotransmitters, not reviewed here, use different pathways for synthesis, storage, release, and ways of communication. Still others follow nonsynaptic neurotransmission.
Synthesis of different neurotransmitters in the presynaptic neuron varies to some extent for specific neurotransmitters using specific amino acids and enzymes. Neurotransmitter synthesis is directed by mRNA and occurs in the cytosol of the axon terminal. The amine and amino acid neurotransmitters are then taken into synaptic vesicles by specialized transporter proteins. The neurotransmitter is stored in the synaptic vesicles in the axonal terminal until it is released into the synaptic cleft.
The peptide neurotransmitters are assembled from the amino acids in the rough endoplasmic reticulum and the active neurotransmitter segment is split in the Golgi apparatus. The secretory granules containing the active peptide then are carried to the axon terminal.
A nerve impulse or action potential in the axon terminal triggers the release of specific neurotransmitter into the synaptic cleft. Depolarization of the cell membrane results in activation of the voltage-gated calcium channels in the active zones to open, allowing influx of calcium ions into the presynaptic axon terminal. The synaptic vesicle is anchored to the cell membrane and the neurotransmitter is spilled into the synaptic cleft, a process called exocytosis. The process of recovery of the synaptic vesicle membrane after the neurotransmitter is released from the vesicle is called endocytosis. Once released into the synaptic cleft, the neurotransmitters can be either destroyed by enzymes or transported back into the presynaptic axon terminal by membrane-bound neurotransmitter-specific transporters.
Postsynaptic receptor occupancy by the neurotransmitter initiates the postsynaptic neurotransmission process. It is thus understood that the electrical nerve impulse in the presynaptic neuron is converted by excitation-secretion coupling into a chemical signal.
Postsynaptic receptor occupancy by the neurotransmitter (first messenger) results in the activation or formation of the second messenger in the postsynaptic neuron. Cyclic adenosine monophosphate (cAMP) and phosphatidyl inositol are examples of second messengers. The second messenger triggers a biochemical cascade and synthesis of various elements, including the transcription factors.
Each gene typically has a coding region and a regulatory region. The regulatory region has an enhancer element and a promoter element. The coding region on the DNA has the template for the corresponding RNA. Transcription factors activated by phosphorylation bind to the promoter region of the DNA, in turn activating the enzyme RNA polymerase. The action of activated RNA polymerase on the coding region results in the formation of the messenger RNA. The process of mRNA formation from DNA template is called transcription and the mRNA is called the transcript. Certain noncoding regions (introns) on the DNA transcribed onto the initial mRNA are removed by the process of RNA splicing, leaving only the coding sequences (exons) in the mRNA. mRNA leaves the nucleus and reaches various sites in the neuron to initiate protein synthesis. The process of assembling various amino acids to create specific proteins as directed by the mRNA is called translation.
These proteins are the building blocks for various elements in the postsynaptic neuron with specialized functions, including neurotransmitter receptors, the ion channels, various peptides and enzymes, transcription factors, neurotransmitter transporter, and neurotropic growth factors. The regulation of gene expression also affects the process of synaptogenesis.
Allosteric Modulation
When a neurotransmitter (primary) occupies its specific receptor site, it triggers the postsynaptic chemical neurotransmission. When a different neurotransmitter (secondary) occupies its receptor site and indirectly influences the primary neuroreceptor transmission, it is said to be modulating the primary neurotransmitter action allosterically. The secondary neurotransmitter can only influence the primary neurotransmitter action when the primary neurotransmitter occupies its specific receptor site. Allosteric modulation can either amplify (positive allosteric interaction) or diminish or block (negative allosteric interaction) the actions of the primary neurotransmitter. For example, the benzodiazepines are known to use positive allosteric modulation whereas antidepressants use negative allosteric modulation.
Nonsynaptic (Volume) Neurotransmission
In addition to the neurotransmission that occurs via the synapse, nonsynaptic or volume neurotransmission is also known to occur. In this case a given neurotransmitter diffuses within a given radius and affects the various receptors with wide-ranging actions. This process is also the basis of single drug acting on different receptors with different actions.
Divergence and Convergence
A given neurotransmitter can activate multiple subtypes of receptors each mediating a different function. In fact, this ability of a neurotransmitter, referred to as divergence, is a common phenomenon, exemplified by glutamate. On the other hand, different neurotransmitters acting on their specific receptors can activate a particular effector system, a phenomenon called convergence. Neurons also have the ability to integrate both the divergent and convergent systems.
Agonists and Antagonists
Naturally occurring neurotransmitters stimulate the receptors on which they bind and are thus said to be agonists . Similarly, pharmacologic agents that stimulate specific receptors are also called agonists. Antagonist agents block the actions of agonists. Antagonists do not have any intrinsic activity of their own, but in the presence of an agonist they block its activity. Antagonists also will block the actions of inverse agonists and partial agonists as well. A pharmacologic agent that binds to a receptor, resulting in action that is opposite to that of agonist, is called an inverse agonist . The action of an inverse agonist is not dependent on the presence of an agonist. Partial agonist binding to a specific receptor will have action that is similar to but relatively weaker than that of an agonist.
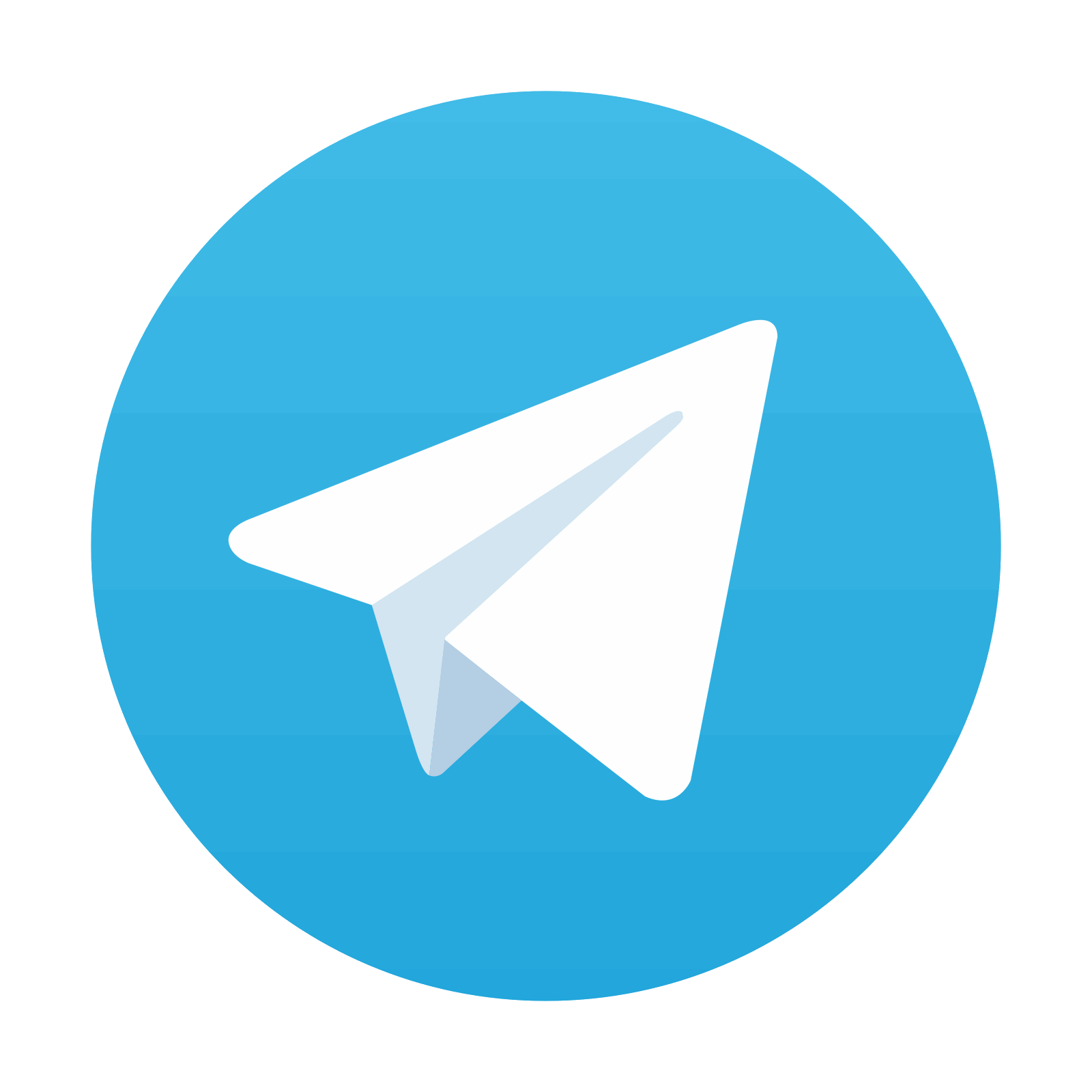
Stay updated, free articles. Join our Telegram channel

Full access? Get Clinical Tree
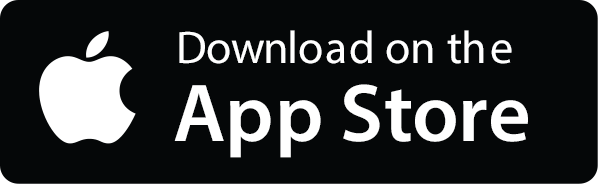
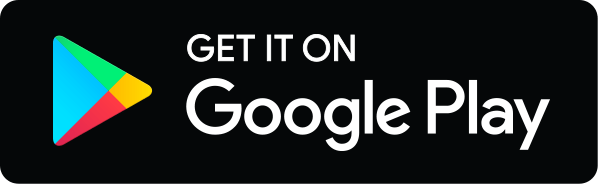