Bacterial Meningitis beyond the Newborn Period
Ralph D. Feigin
Meningitis is an inflammation of the meninges. Between 1986 and 1995, the U.S. incidence of bacterial meningitis in children aged 1 month to 5 years decreased 87%, primarily because of the introduction of conjugate vaccine for Haemophilus influenzae type b (Hib) for children younger than 2 years of age. However, because death can occur in more than 5% of cases and morbidity may occur in 30% of survivors, it is still a feared childhood infection. Because of their different characteristics, neonatal and tuberculous meningitis are discussed in Chapters 72 (Meningitis) and 177, respectively.
EPIDEMIOLOGY
Common causes of bacterial meningitis in children older than 1 month of age are Neisseria meningitidis, Streptococcus pneumoniae and, until recently, Hib. Most cases occur in children between 1 month and 5 years of age, with the highest risk being in infants aged 6 to 12 months. Table 136.1 shows the incidence by age for the five most common organisms. Since the introduction of conjugate Hib vaccine, the average age of U.S. cases has increased to approximately 25 years, up from 15 months in 1986. Before the introduction of conjugate Hib vaccine, approximately 65% of U.S. bacterial meningitis was caused by Hib, with the remainder caused primarily by N. meningitidis and S. pneumoniae. Other countries had different rates. For example, in Spain, N. meningitidis caused approximately 68% of cases of childhood bacterial meningitis. Nonspecific risk factors for meningitis from these organisms include young age, close contact with carriers or those with invasive disease, and host factors such as asplenia or immunodeficiency.
N. meningitidis is a gram-negative diplococcus with 12 capsular polysaccharide serotypes. Approximately 90% of meningococcal disease is caused by groups A, B, and C. Serogroup prevalence differs throughout the world and changes with time. The most prevalent forms in the United States are groups B and C, with some serogroup Y outbreaks reported. Increases in rates of serogroup C have been reported in Canada, the United States, and the United Kingdom. U.S. group A epidemics occurred as recently as the mid-twentieth century. Epidemics of group A N. meningitidis occur every 8 to 12 years in the “meningitis belt” of sub-Saharan Africa, where attack rates of 500 per 100,000 can occur.
The incidence of meningococcal meningitis in U.S. children aged 1 to 23 months is 4.5 per 100,000. The U.S. childhood mortality rate has been estimated at 8%. Meningococcal disease is more common in males and is increased in frequency in the later teens. The disease generally is acquired from carriers who can harbor the organism for months. The U.S. carriage rate is 15%, but it increases to more than 30% during outbreaks, and the carriage rate of unrelated subgroups increases as well. The incidence of disease peaks in winter, and the disease occurs in newly infected individuals rather than by breakthrough in chronic carriers. The risk of developing severe meningococcal disease is 1% in family contacts, approximately 1,000 times greater than the community risk. The risk for day-care contacts is 1 per 1,000. The incubation period is from 1 to 10 days.
Host factors, such as terminal complement deficiency (C5 to C9), complement-depleting diseases, or properdin deficiency, increase susceptibility to disease. Individuals with such deficiencies may develop disease late; in one study, patients developed disease at an average age of 17 years. Influenza or mycoplasma outbreaks in the preceding 2 to 3 weeks are associated with subsequent meningococcal outbreaks, possibly because of impaired pharyngeal defenses or lowered immunity.
S. pneumoniae is a gram-positive diplococcus with approximately 90 capsular polysaccharide serotypes. Sepsis and meningitis occur most frequently with serotypes 4, 6B, 9V, 14, 18C, 19F, and 23F. The meningitis rate in U.S. children aged 1 to 23 months is 6.6 per 100,000. The U.S. childhood mortality rate has been estimated at 15%. Meningitis is associated with viral upper respiratory infections and is most prevalent in winter. Carriage is transient, and it is a risk factor for development of infection.
Host factors are important with S. pneumoniae meningitis. The risk of developing meningitis in the U.S. black population is increased 5 to 36 times, and this risk is independent of income
or population density. In the absence of antibiotic prophylaxis, one in 24 children with sickle cell disease may develop S. pneumoniae meningitis by age 4 years, a rate 36 times that of black children without sickle cell disease.
or population density. In the absence of antibiotic prophylaxis, one in 24 children with sickle cell disease may develop S. pneumoniae meningitis by age 4 years, a rate 36 times that of black children without sickle cell disease.
TABLE 136.1. ESTIMATED AGE-SPECIFIC INCIDENCE OF BACTERIAL MENINGITIS (CASES PER 100,000 POPULATION), UNITED STATES, 1995 | ||||||||||||||||||||||||||||||
---|---|---|---|---|---|---|---|---|---|---|---|---|---|---|---|---|---|---|---|---|---|---|---|---|---|---|---|---|---|---|
|
The incidence of meningitis caused by penicillin-resistant S. pneumoniae has increased dramatically in recent years. In 1998, Centers for Disease Control and Prevention (CDC) surveillance from multiple areas around the United States found that 24% of invasive pneumococcal infections were nonsusceptible to penicillin and 14% of isolates were nonsusceptible to cefotaxime. Rates of resistance were highest in children younger than 5 years of age.
Hib is a gram-negative coccobacillus. Historically, it was the leading cause of bacterial meningitis in many developed countries. The incidence in children younger than 5 years of age ranged from 20 to 30 per 100,000 in certain countries to 409 per 100,000 in Alaskan Eskimos. In countries where Hib immunization is started when children are 2 months of age, a dramatic decrease in the incidence of disease has occurred. In Canada, a 98% reduction in the number of infections caused by Hib occurred from 1985 to 1995. The United States has experienced a similar decline, with a current incidence of 0.4 per 100,000 in children between 1 and 23 months. The U.S. mortality rate has been estimated at 2.3%. In countries where Hib vaccination is not yet widespread, the disease continues to be a common occurrence, with a peak incidence occurring in late autumn or early winter. The normal carriage rate of 2% to 5% is declining because the vaccine also protects against nasopharyngeal colonization. The risks of transmission of infection to a family contact are 6% and 2% for members younger than 1 year and 4 years of age, respectively. The risk of secondary infection developing in home contacts within 30 days of an index case is 585 times greater than the age-adjusted risk in the general population.
Host factors are important because meningitis occurs with increased frequency in children with diabetes mellitus, Cushing syndrome, and coma secondary to drug overdose. Genetic factors also may play a role. In one study, HLA-B12 was found in 52% of children with invasive H. influenzae disease versus 16% in a control group. HLA-Bw40 was present in 24% of children without invasive disease, but it was absent in those with systemic Hib infection. Additional studies have identified other genetic risk factors.
Other bacteria, such as group B streptococcus, Listeria monocytogenes, Salmonella, and Fusobacterium necrophorum, can cause meningitis in normal children. Host factors, both congenital and acquired, can be important in the development of meningitis caused by these bacteria. Skin flora should be suspected in children with a dermoid sinus, meningomyelocele, or hydrocephalus and a cerebrospinal fluid (CSF) shunt. Meningitis occurring after cochlear implantation has been reported. Cystic fibrosis or burn patients may develop Staphylococcus aureus or Pseudomonas aeruginosa meningitis after colonization. In a humidified atmosphere, P. aeruginosa or Serratia marcescens infection may occur. Children with sickle cell disease, congenital asplenia, or splenosis are especially susceptible to Salmonella infection, in addition to H. influenzae and S. pneumoniae. Children who have reticuloendothelial malignancies, are undergoing chemotherapy, or have indwelling catheters may develop meningitis from organisms of low virulence, such as Streptococcus mitis. In immunocompromised children, Bacteroides fragilis is a frequent anaerobic cause of meningitis. Congenital or acquired anatomic defects, such as a stapedial footplate defect or cribiform plate fracture, should be investigated in cases of recurrent meningitis.
Meningitis with two bacterial types in a CSF culture may occur in 1% of cases. Meningitis with a bacteria and a virus or fungus occurs rarely. The clinical course usually is that of bacterial meningitis.
PATHOGENESIS
Initially, upper respiratory tract infection occurs. Bacteremia follows, with opsonization and phagocytosis inhibited by bacterial capsules. Meningeal seeding occurs, most likely in the cerebral capillaries and choroid plexus. Invasion from a contiguous infection (e.g., mastoiditis) also can occur. Meningitis that develops after otitis media usually is from bacteremia rather than direct invasion. Meningitis also can develop subsequent to skull or vertebral column osteomyelitis.
Organisms initially are found in the lateral and dorsal longitudinal (sagittal) sinuses. Central nervous system (CNS) blood flows may be reduced by 25% to 50%. Dural inflammation slows the flow from the subarachnoid space to the sinuses, thereby permitting spread of infection. A meningeal exudate occurs over the brain. The spinal cord may be encased in pus. Purulent material may develop in the ventricles (ventriculitis) and the ventricular wall and around the veins and venous sinuses.
Cerebral cortex damage produces the neurologic sequelae of meningitis, such as impaired consciousness, seizures, retardation, and nerve deficits. These symptoms result from complications such as nerve inflammation, cerebral edema, increased intracranial pressure (ICP), cerebral vascular changes, hypoglycorrhachia (reduced CSF glucose), acidosis, and the host inflammatory response.
Nerve inflammation leads to meningeal symptoms and signs. Early pressure on peripheral nerves may lead to motor or sensory deficits. Subarachnoid cranial and spinal nerves often are involved in the inflammatory process. Deafness and vestibular disturbances occur most commonly, and optic nerve involvement also may occur. Hearing loss appears to result from inflammatory cells and mediators that reach the inner ear from the CSF via the cochlear aqueduct. Cochlear damage with loss of the organ of Corti may result. Inflammation and swelling of the facial nerve in the stylomastoid foramen and the auditory nerve in the internal acoustic meatus may compromise their blood supply. Transtentorial herniation also may cause nerve compression.
Cerebral edema results from vasogenic, interstitial, and cytotoxic processes. Vasogenic edema results from increased permeability of the blood brain barrier. Interstitial edema occurs with decreased CSF resorption as proteins, leukocytes, and debris interfere with the function of the arachnoid villi. Cytotoxic edema results from host and bacterial toxic factors, which increase intracellular water and sodium and loss of intracellular potassium.
Increased ICP often exceeds 300 mm H2O. Hypoxemia and ischemia from decreased perfusion may result. Papilledema is a rare occurrence because of the brief duration of increased pressure. Hydrocephalus rarely occurs beyond the neonatal period. Communicating hydrocephalus may result from adhesive arachnoid thickening about the basal cisterns. Obstructive hydrocephalus may result from fibrosis and reactive gliosis obliterating the aqueduct of Sylvius or the foramina of Magendie and Luschka. In some cases, meningitis is associated with the syndrome of inappropriate antidiuretic hormone release (SIADH), causing water retention and a relative sodium loss by the kidney. SIADH increases the risk of developing electrolyte abnormalities, increased ICP, and seizures. Dehydration also can occur from increased insensible losses and decreased intake.
Cerebral vascular changes include vasculitis and major vascular events such as venous sinus occlusion or subarachnoid hemorrhage from necrotizing arteritis. Cerebral necrosis can result with or without vascular thrombosis. Combined with increased intraventricular pressure, it may lead to infarction or dissolution of the cerebrum. Autoregulation also may be
destroyed, in which case, cerebral perfusion is a function of the systolic blood pressure.
destroyed, in which case, cerebral perfusion is a function of the systolic blood pressure.
Hypoglycorrhachia and acidosis result in part from increased glucose use and decreased glucose transport across the inflamed choroid plexus. Increased glucose use results in the excess production of lactate production and the depletion of the high-energy compounds adenosine triphosphate and phosphocreatine. The resultant acidosis may contribute to a loss of cerebral autoregulation and tissue damage. Increased concentrations of lactate also occur from anaerobic metabolism secondary to hypoxemia.
Increased CSF protein is caused partly by the flow of albumin-rich fluid into the subdural space secondary to inflammation and increased vascular permeability. With resolution of the inflammatory process, vascular permeability decreases, but continued transudation from newly formed subdural capillaries may persist. Subdural effusions may occur by these processes.
Host inflammatory responses in the CSF seem to rely on two mechanisms to clear bacteria. One requires a type-specific antibody, a functional classic complement system for opsonization, and competent neutrophils for phagocytosis. The second system is dependent on the interaction of nonspecific antibody and the alternative complement system for opsonization of the organism. Clearance by this system occurs without neutrophils.
Low concentrations of CSF antibody and complement initially permit bacterial proliferation. A low level of release occurs of cell wall fragments, consisting of endotoxin from gram-negative bacteria and teichoic acid from gram-positive bacteria. The interaction between these fragments and the blood brain barrier endothelium results in increased barrier permeability. Serum albumin, complement, and other proteins flow into the CSF. Early increased regional blood flow and ICP also may occur.
The host inflammatory response remains only partially understood and appears to be somewhat different for each type of organism. It includes a complex interaction of leukocytes, cytokines, complement, arachidonic acid products, platelet-activating factor (PAF), nitric oxide (NO), toxic oxygen products, and excitatory amino acids. Pathologic changes have been noted in the cerebral cortex, consisting of reactive microglia and astrocytes, without evidence of cortical bacterial invasion. Experimental studies show that neurologic outcome may be a function of subarachnoid inflammation and that neutrophils may do little to control the subarachnoid infection. Bacterial concentrations do not differ between normal and neutropenic animal models. In experimental models, leukocyte migration inhibition is associated with improved outcomes, including increased rates of survival and decreased brain edema, disruption of the blood brain barrier, and tissue damage.
Antibiotic therapy results in a large release of bacterial fragments, which promotes production of the cytokines IL-1β (IL-1β) and tumor necrosis factor–alpha (TNF-α). These cytokines trigger a cascade of mediators, including IL-6, IL-8, IL-10, IL-12, phospholipase A2, and interferon-gamma. IL-1β and TNF-α also stimulate endothelial cells to activate receptors that promote leukocyte attachment, leading to leukocyte migration into the CSF. In experimental studies, antibodies to IL-1β and TNF-α prevented the development of CSF leukocytosis. Although IL-1β and TNF-α are not directly toxic to neurons, CSF IL-1 levels are correlated with CSF inflammation, increased TNF-α concentration, and adverse outcome. CSF TNF-α causes increased vascular permeability by virtue of the toxic effects on endothelial cells and, in vitro, it causes myelin and oligodendrocyte necrosis. In one study, it was correlated with the number of febrile hospital days. Increased soluble TNF-α receptor, a regulatory protein for TNF-α, has been correlated with adverse neurologic sequelae.
Complement is involved early in the host response. In experimental studies, complement depletion delayed the appearance of leukocytes in the CSF. The complement cascade product C5a is a potent early chemoattractant for leukocytes.
The ILs (6, 8, 10, 12) and interferon-gamma have multiple roles, a few of which are described here. IL-6 works with IL-1 to promote B-cell growth and induces acute-phase reactants such as C-reactive protein (CRP). It also produces fever and activates the complement and clotting cascades. IL-8 is a chemoattractant that also stimulates the production of reactive oxygen metabolites and neutrophil degranulation. IL-10 regulates TNF-α levels and decreases NO and reactive oxygen metabolite synthesis. It also increases the release of soluble TNF-α receptors. IL-12 enhances cytolytic activity in T cells, natural killer cells, macrophages, and lymphokine-activated killer cells. IL-12 also stimulates release of interferon-gamma, which, among other actions, promotes phagocytosis and the secretion of reactive oxygen metabolites. CSF interferon-gamma levels and the ratio of TNF-a to IL-10 were found to be significantly different between patients with pneumococcal meningitis and those with Hib or meningococcal meningitis.
Phospholipase A2 leads to the production of arachidonic acid from cell membranes; it then is converted to prostaglandins (PG), thromboxanes, and leukotrienes (LT). PGE2 and prostacyclin reduce TNF-α mRNA production, providing a feedback system. In experimental studies, PGE2 given with C5a resulted in decreased CSF leukocytosis. Arachidonic acid products provide a late stimulus for the recruitment of leukocytes that is independent of complement. Certain inhibitors of arachidonic acid (such as the cyclooxygenase inhibitor oxindanac) were associated with decreased leukocytosis and improved experimental survival. Indomethacin, a PGE2 inhibitor, reduced cerebral edema but did not affect ICP. LTB4 promotes the migration of neutrophils, among other actions, and can be produced by neutrophils. This process is inhibited by dexamethasone.
Phospholipase A2 also leads to PAF production. Whereas the role of PAF in meningitis is not clear, in vitro it induces chemotaxis and platelet degranulation, triggers the coagulation cascade, and increases capillary permeability. In high concentrations, PAF is toxic to neurons and causes endothelial tissue damage.
IL-1β, TNF-α, PGE2, PAF, NO, and LTB4 are among the many mediators that increase ICP and cerebral edema by altering cerebral blood flow and blood brain barrier permeability. In experimental meningitis, an early increase in cerebral blood flow and blood pressure occurs within hours of initial development of infection. This increase may be mediated by NO, which is elevated in bacterial meningitis. When TNF-α was injected into the cisterna of experimental animals, NO mediated oxygen uptake and CSF–to–blood glucose ratios were reduced, whereas ICP and lactate concentrations were increased.
Other factors in the host response include neutrophil enzymes, oxygen radicals, and excitatory amino acids, all of which may cause neuronal death. Superoxide and hydrogen peroxide are secreted by brain microglial cells and leukocytes. The experimental infusion of superoxide dismutase prevents early increased regional blood flow, loss of autoregulation, ICP changes, and edema. These findings may point to an early role for peroxynitrite, a toxic metabolite formed from superoxide anions and NO. Increased concentrations of certain amino acids also have been found in bacterial meningitis. In one adult study, patients who had high CSF glutamate concentrations several days after starting antibiotics developed severe neurologic complications or died. Glutamate is an excitatory amino acid implicated in neuronal damage. It can be secreted by macrophages.
Significant additional discoveries may be made in the coming years. Pharmaceutical interventions designed to block inappropriate portions of the host response are being developed.
CLINICAL MANIFESTATIONS AND COMPLICATIONS
Meningitis can develop slowly over the course of several days, or it can be fulminant, with onset occurring within hours. The fulminant form is associated with severe brain edema, which can lead to cerebral herniation.
Fever and meningeal inflammation symptoms occur in 85% of patients. Symptoms of patients with meningitis are summarized in Table 136.2. They include headache, irritability, confusion, hyperesthesia, photophobia, nuchal rigidity, and seizures. Nuchal rigidity may appear late, especially in a young child. Seizures occur in 30% of cases at some time before or during the course of illness; in 20% of cases, seizures occur before admission. Infants may have a bulging fontanelle, but such appears late and in only approximately 30% of infants. In one study, bulging fontanelles occurred in 13% of children without meningitis. Older children frequently have headaches, and symptoms may proceed to altered mental status, hypertension, and bradycardia from increased ICP. In one series, approximately 15% of patients were semicomatose or comatose at admission, conditions more common with pneumococcal or meningococcal disease. Papilledema is a rare development; if present, a search for other processes (e.g., brain abscess, venous sinus occlusion, subdural empyema) should be performed. Transient or permanent cranial nerve damage may cause deafness, vestibular disturbance, ataxia, and extraocular or facial nerve paralysis. Optic-nerve arachnoiditis may lead to optic atrophy and blindness. Focal neurologic deficits may exist on admission in 15% of children and in 30% of children with pneumococcal meningitis. When focal signs are present without seizures, cortical necrosis, occlusive vasculitis, or thrombosis of cortical veins probably has occurred. Infants may have only restlessness, irritability, poor feeding, or unstable temperature. Adolescents may present with behavioral abnormalities that may be confused with drug abuse or psychiatric disorders.
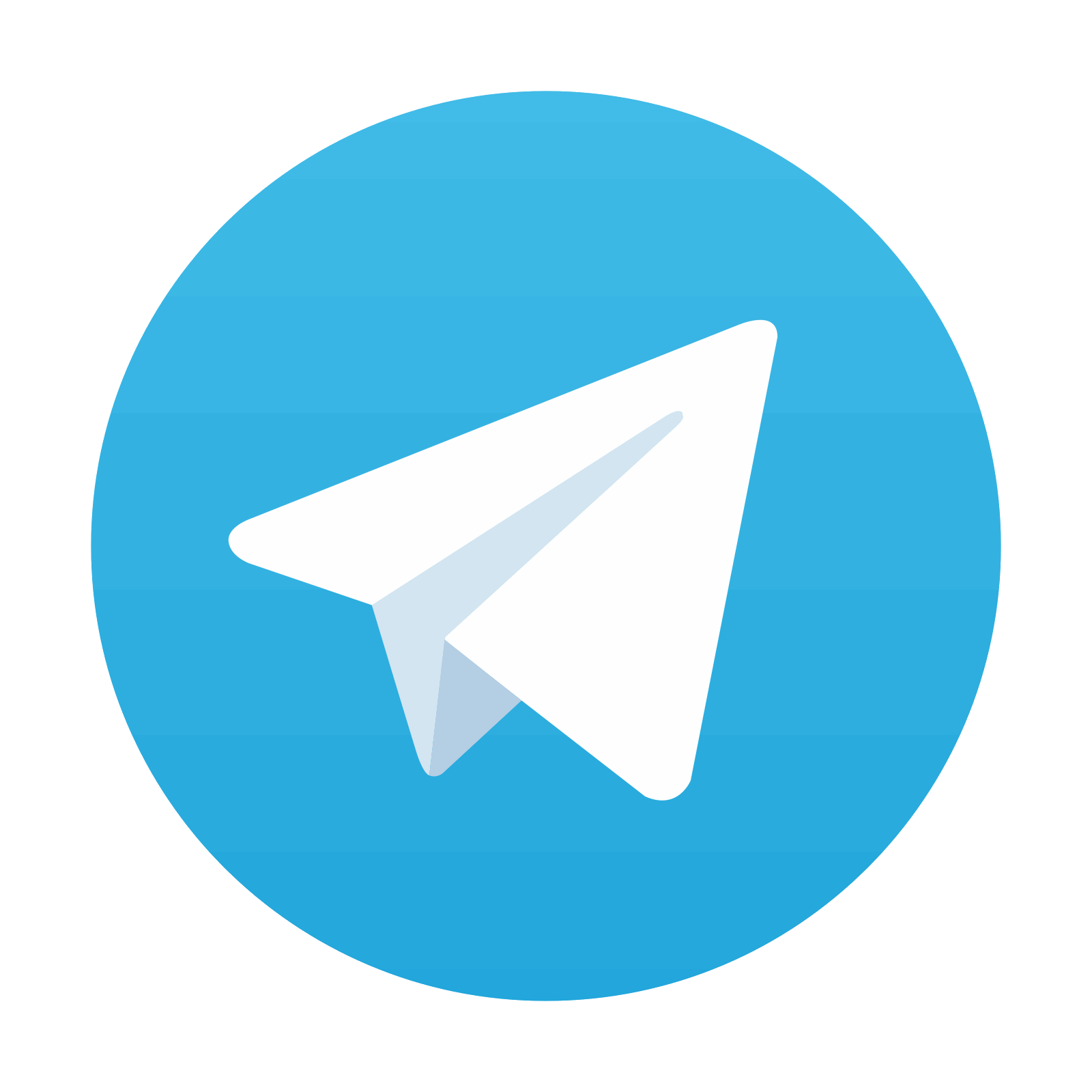
Stay updated, free articles. Join our Telegram channel

Full access? Get Clinical Tree
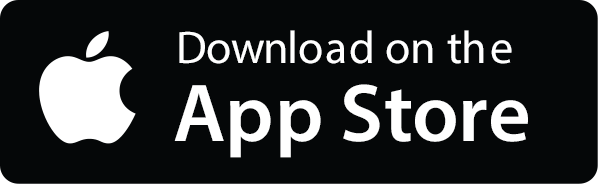
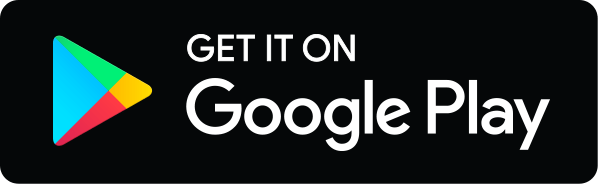