Bacterial meningitis is an inflammation of the meninges affecting the pia, the arachnoid, and the subarachnoid space that occurs in response to bacteria and bacterial products. A discussion of infections of neonates, including neonatal bacterial meningitis, is presented here; infections of the central nervous system (CNS) caused by mycobacteria and fungi are discussed in other chapters.
Incidence and Epidemiology
Before the discovery and use of antibiotics, bacterial meningitis generally was fatal. Antibiotic therapy has dramatically improved the prognosis in patients with bacterial meningitis, although bacterial meningitis continues to be a significant cause of morbidity and mortality in infants and children. The number of deaths attributed to many other infectious diseases in the United States decreased by 10- to 200-fold between 1935 and 1968, whereas the number of reported deaths caused by bacterial meningitis decreased by only half during the same period.
The epidemiology of bacterial meningitis has been changed by several preventive measures introduced since the early 1990s, such as introduction of the Haemophilus influenzae type b conjugate vaccines and the pneumococcal conjugate vaccines for infants, and the screening of pregnant women for group B Streptococcus and intrapartum antibiotic prophylaxis since 1996. The meningococcal conjugate vaccines were introduced in 2005, but the incidence of meningococcal meningitis was decreasing before the availability of conjugate vaccines.
Before the widespread use of the H. influenzae type b conjugate vaccines, H. influenzae type b was the most common cause of bacterial meningitis in children in the United States, Canada, and Scandinavia, but this pattern was not universal. Data from England from 1968 to 1977 showed that Neisseria meningitidis was the most common cause of bacterial meningitis for children and young adults, and meningococcal meningitis accounted for 48% of meningitis in children in England. A recent surveillance in England and Wales from 2004 to 2011 reports that meningococcal meningitis remains more common than pneumococcal meningitis (45% vs. 35% of bacterial meningitis, respectively).
Studies in the 1970s and 1980s showed that three pathogens ( H. influenzae, Streptococcus pneumoniae, and N. meningitidis ) are predominant in causing meningitis in infants, children, and young adults, whereas group B Streptococcus , Escherichia coli, and Listeria monocytogenes are the most common bacteria causing meningitis in neonates. Between 1986 and 1995, the incidence of bacterial meningitis declined markedly, largely owing to the introduction of H. influenzae type b conjugate vaccines in infants. After the introduction of the heptavalent pneumococcal conjugate vaccine (PCV7), including pneumococcal serotypes 4, 6B, 8C, 9V, 14, 19F, and 23F, to infants in 2000, invasive pneumococcal disease in the United States declined by 75% among children younger than 5 years of age as well as by 31% among adults 65 years or older and in unvaccinated populations through herd immunity.
In 1972, the Centers for Disease Control and Prevention (CDC) estimated that in the United States, 29,000 cases of meningitis were caused by H. influenzae type b, 4800 cases were caused by S. pneumoniae, and 4600 cases were caused by N. meningitidis. However, an estimate of an average of 4100 cases of bacterial meningitis, including 500 that were fatal, occurred annually in the United States during 2003 to 2007. The data obtained from a population-based observational study using ICD9 coding between 1997 and 2010 indicate that the incidence of pneumococcal meningitis fell from 0.8 per 100,000 people in 1997 to 0.3 per 100,000 people by the end of 2010, while the incidence of meningococcal meningitis decreased from 0.72 per 100,000 in 1997 to 0.12 per 100,000 people in 2010, and the incidence of H. influenzae meningitis has remained at 0.058 per 100,000 people.
Population-based studies in South Carolina, Minnesota, Vermont, and New Mexico in the 1970s suggested that the actual incidence of bacterial meningitis ranged from 5.4 to 7.3 cases per 100,000 population. Studies reported from the CDC in 2011 indicated that the incidence of bacterial meningitis ranged from 6.91 cases per 100,000 population for children aged 2 to 23 months to 0.43 case per 100,000 population for adolescents aged 11 to 17 years ( Table 31.1 ).
Age | 1998–99 | 2006–07 | % Change 2006–07 vs. 1998–99 |
---|---|---|---|
<2 mo | 74.46 | 80.69 | 10 |
2–23 mo | 14.20 | 6.91 | −51 |
2–10 y | 1.55 | 0.56 | −64 |
11–17 y | 1.03 | 0.43 | −58 |
18–34 y | 0.99 | 0.66 | −33 |
35–49 y | 1.23 | 0.95 | −23 |
50–64 y | 2.15 | 1.73 | −19 |
≥65 y | 2.64 | 1.92 | −27 |
All ages | 2.00 | 1.38 | −31 |
A recent report of bacterial meningitis in the United States from 1998 to 2007 showed that the overall incidence of meningitis declined by 31% from 2.00 cases per 100,000 population in 1998 to 1999 to 1.38 cases per 100,000 population in 2006 to 2007, but the incidence remained unchanged in patients younger than 2 months of age (see Table 31.1 ). The incidence of bacterial meningitis was highest for patients younger than 2 months of age and for black patients of any age. The median age of patients increased from 30.3 years during 1998–99 to 41.9 years during 2006–07, and this shift was significant, suggesting that the burden of bacterial meningitis is borne more by older adults. However, the case-fatality rate did not change significantly, from 15.7% in 1997–98 to 14.3% in 2006–07.
In 2003 to 2007 in the United States, the most frequent cause of bacterial meningitis in children younger than 2 months of age was group B Streptococcus (86.1% of cases), and N. meningitidis caused 45.9% of cases among those 11 to 17 years of age. Among the other pediatric age groups, S. pneumoniae was the most common cause ( Table 31.2 ). The case-fatality rate was 6.9% among pediatric patients, and nearly 10% had underlying immunocompromising or chronic medical conditions. E. coli and L. monocytogenes are other common causes of meningitis in neonates 2 to 6 weeks of age.
Pathogen | 1998–99 | 2006–07 | % Change 2006–07 vs. 1998–99 |
---|---|---|---|
S. pneumoniae | 1.09 | 0.81 | −26 |
N. meningitidis | 0.44 | 0.19 | −58 |
Group B streptococcus | 0.24 | 0.25 | 4 |
H. influenzae | 0.12 | 0.08 | −35 |
L. monocytogenes | 0.10 | 0.05 | −46 |
Epidemiology of Meningitis Caused by Streptococcus pneumoniae
S. pneumoniae is the most common pathogen causing bacterial meningitis in the United States. The risk for development of sepsis or meningitis caused by S. pneumoniae depends to some extent on the serotype with which the child is colonized and against which there is no opsonic antibody. Although more than 90 pneumococcal serotypes have been identified, invasive disease, including sepsis and meningitis, in children younger than 6 years old was associated most commonly with serotypes 14, 6B, 19F, 18C, 23F, 4, and 9V in the United States. The 7-valent pneumococcal conjugate vaccine (PCV7), added to the national vaccination schedule in 2000, contains each of the just-mentioned most common serotypes.
Nasopharyngeal colonization in children mainly depends on age (young age), crowding, attendance at daycare centers, and ethnicity (the Native American population). The serotype distribution of colonization isolates is by and large a reliable indicator for invasive disease, antibiotic resistance profiles, and potential vaccine coverage. Daycare attendance, underlying disease, and lack of breast-feeding were risk factors for acquiring invasive pneumococcal infections in a large case-control study that included children from the United States and Canada. Although cigarette smoking is a major risk factor for the development of invasive pneumococcal disease in adults, whether exposure to cigarette smoke increases the risk for developing systemic pneumococcal infection in children remains unclear.
In the prevaccine era (before 2000 in the United States), the age-specific incidence for pneumococcal meningitis was 21, 12, 6, 2, and 0.5 cases per 100,000 for the age groups 0 to 6 months, 7 to 12 months, 13 to 24 months, 25 to 60 months, and older than 60 months, respectively. The overall incidence of S. pneumoniae meningitis, however, decreased by 26% from 1.09 cases per 100,000 population in 1998 to 1999 to 0.81 case per 100,000 in 2006–07 in the United States. This decline included a decrease of 62% among children 2 to 23 months of age ( Table 31.3 ). The incidence of meningitis from S. pneumoniae PCV7 serotypes declined by 92% from 0.61 case per 100,000 population in 1998–99 to 0.05 case per 100,000 population in 2006–07. However, the incidence of meningitis from non-PCV7 serotypes increased by 62% from 0.48 case per 100,000 population in 1998–99 to 0.77 case per 100,000 population in 2006–07. Pneumococcal meningitis had a case-fatality rate of 17.9% in 1998–99 and 14.7% in 2006–07.
Age | 1998–99 | 2006–07 | Percent Change 2006–07 vs. 1998–99 |
---|---|---|---|
2–23 mo | 9.69 | 3.67 | −62 |
2–10 y | 0.54 | 0.36 | −34 |
11–17 y | 0.20 | 0.21 | 7 |
18–34 y | 0.40 | 0.27 | −33 |
35–49 y | 0.83 | 0.76 | −8 |
50–64 y | 1.47 | 1.34 | −9 |
≥65 y | 1.88 | 1.43 | −24 |
All ages | 1.09 | 0.81 | −26 |
Among children aged 2 months to 10 years, S. pneumoniae was the most common cause of bacterial meningitis. PCV7 serotypes accounted for 15.5% of the pediatric cases overall and 13.0% of cases in the 2- to 23-month age groups. The case-fatality rates were similar among children infected with PCV7 isolates and those infected with non-PCV7 isolates (10.7% and 7.6%, respectively). Use of PCV7 also reduced invasive pneumococcal disease, including meningitis among unvaccinated populations through herd immunity (see Table 31.3 ). PCV13 serotypes (PCV7 serotypes plus serotypes 1, 3, 5, 6A, 7F, and 19A) accounted for 60.0% of cases in children between 2 and 23 months of age and 57.2% in children of any age. PCV7 and PCV13 serotypes accounted for 16.0 and 41.6% of the adult meningitis cases, respectively. The overall fatality rate was 16.4%, and the rate increased linearly with increasing age (8.9% among patients 18 to 34 years of age to 22.7% among those 65 years and older, P < .001). Vaccine serotype coverages by PCV7 and PCV13 are less in European and other countries than those in the United States. Surveys of nasopharyngeal carriage of S. pneumoniae in Australia revealed that the percentages of serotypes included in PCV13 were 40% in 2- to 15-year-old children and 29% in adults.
Pneumococcal infections generally occur sporadically. Household contacts of a patient with pneumococcal disease are not considered to be at increased risk for acquiring secondary infection. The occurrence of concurrent pneumococcal disease (meningitis and bacteremia) in the household setting has been reported, however. Although uncommon in the United States, S. pneumoniae has been associated with epidemics in locations such as Northern Ghana.
The incidence of systemic infection with penicillin-resistant S. pneumoniae has been increasing steadily worldwide since it first was reported in Australia in the 1960s. Systemic infection with penicillin-resistant pneumococci has become an increasing problem in the United States since the mid-1980s. In 1995, a CDC multistate surveillance study showed that 35% of cerebrospinal fluid (CSF) isolates of S. pneumoniae were resistant to penicillin.
The first report of meningitis caused by resistant pneumococci was published in 1974, and numerous case reports appeared subsequently. Patients with systemic infections caused by penicillin-resistant pneumococci were more likely to have received a course of antibiotics within 1 month before acquiring their infection than were matched control subjects who had infections caused by pneumococci but whose isolates were susceptible to penicillin.
By 1998, CDC surveillance of multiple areas around the United States found that 24% of pneumococcal isolates associated with invasive infections were nonsusceptible to penicillin; 14% of isolates were nonsusceptible to cefotaxime. As with many studies, rates of resistance were higher among children younger than 5 years old. In contrast, penicillin- and cefotaxime-nonsusceptible pneumococcal isolates were 10.5% and 8.7%, respectively, in 2010. In a prospective study involving eight children’s hospitals nationwide during a 6-year period starting September 1993, a significant increase in the proportion of isolates nonsusceptible to penicillin or ceftriaxone was found. In the sixth year of the study ending August 1998, 37% and 11% of invasive isolates were nonsusceptible to penicillin and ceftriaxone, respectively. Isolates of penicillin-resistant and third-generation cephalosporin-resistant S. pneumoniae have been recovered in other regions of the world as well. Treatment failures in patients with S. pneumoniae meningitis resistant to penicillin and third-generation cephalosporins have led to changes in the empiric therapy of suspected bacterial meningitis, as discussed in the section on treatment .
Data from the Active Bacterial Core Surveillance of the Emerging Infections Program Network after introduction of PCV7 showed an 87% decrease in rates of antibiotic-resistant disease by vaccine serotypes. These results show that rates of invasive pneumococcal disease with penicillin-nonsusceptible strains and strains nonsusceptible to multiple antibiotics “peaked in 1999 and decreased by 2004.” In children younger than 2 years old, invasive pneumococcal disease with penicillin-nonsusceptible strains decreased from 70.3 to 13.1 cases per 100,000. The proportion of disease caused by penicillin-nonsusceptible strains varied by location within the United States from 9.4% in California to 29.5% in Tennessee. The incidence of invasive S. pneumoniae disease caused by cefotaxime-resistant S. pneumoniae strains increased in children younger than 2 years of age, however, in the postvaccination period, from approximately 10% in 1999 to almost 15% in 2003. The decreases in disease caused by vaccine-type S. pneumoniae strains were coupled with an increase in nonvaccine serotype S. pneumoniae strains, especially with serotype 19A (increased from 2.0 to 8.3 cases per 100,000).
Recent data from 1998 to 2009 from continuous surveillance of invasive pneumococcal disease in eight geographic areas of the United States indicate that reductions in rates ranged by site from 19.0 to 29.9 cases per 100,000 population during 1998–99 to 11.2 to 18.0 cases per 100,000 population during 2009 (rate reduction of 5.1 to 15.3 cases per 100,000 population). Reductions among children aged younger than 5 years ranged from 35.7 to 117.2 cases per 100,000 population across the sites. Reductions in rates due to PCV7 serotypes were seen in all age groups and at all sites, ranging from 12.0 to 21.4 cases per 100,000 population during 1998–99 to less than 2.0 cases per 100,000 population during 2009 (92% to 98% reductions). Serotype 19A rates ranged from 0.4 to 1.5 cases per 100,000 population during 1998–99 to 1.3 to 3.4 cases per 100,000 population during 2009 (rate difference of 0.9 to 2.8 cases per 100,000 population). Rates due to all other serotypes ranged from 6.3 to 10.3 cases per 100,000 population during 1998 to 1999 to 8.3 to 13.6 cases per 100,000 population during 2009 (rate difference of −0.04 to 5.67 cases per 100,000 population). Across the sites, the greatest increases were seen in the 50- to 64-year-old and older than 65-year-old age groups. In February 2010, PCV13 was licensed and replaced PCV7 in the United States; PCV13 significantly reduced invasive pneumococcal disease among all age groups, including those younger than 2 years and those older than 65 years ( Fig. 31.1 ), supporting the herd protection theme against pneumococcal disease. Screening for underlying immunologic investigation maybe needed for children with invasive pneumococcal disease, particularly in those older than 2 years because immunodeficiencies have been discovered in up to 26% of cases.

Epidemiology of Meningococcal Meningitis
In the United States, the incidence of N. meningitidis meningitis decreased by 58% from 0.44 case per 100,000 population in 1998–99 to 0.19 case per 100,000 population in 2006–07 ( Fig. 31.2 ; also see Table 31.2 ). Since 2005, declines have occurred among all age groups and in all vaccine-containing serogroups. The rates of meningococcal meningitis caused by serogroups B, C, and Y declined by 55%. Meningitis with N. meningitidis had a case-fatality rate of 10.1%, and 11% to 19% of survivors sustain long-term sequelae (e.g., neurologic disability or limb loss). N. meningitidis remained a major cause of bacterial meningitis among older children and young adults between 11 and 17 years of age (45.9% of cases), despite the fact that rates declined significantly between 1997 and 2007. Because the declines were similar among all major serogroups and because quadrivalent meningococcal vaccines (A, C, Y, and W135) do not include serogroup B, these declines are not likely from a vaccine effect. Serogroups B, C, and Y were most common in the United States, each accounting for approximately one-third of the cases. However, the proportion of cases caused by each serogroup varies by age group. Serogroup B caused 70.5% of the cases among children younger than 11 years of age. Serogroups included in quadrivalent meningococcal vaccines accounted for 66.7% of infections among children 11 to 17 years of age. Serogroups B and C were the predominant causes of meningococcal meningitis among adults 18 to 34 years of age, accounting for 34.4% and 45.9% of cases, respectively, whereas in adults 35 years of age or older, serogroups B and Y were the most common, each serogroup accounting for 30.4% of cases. As the proportion of children receiving quadrivalent meningococcal conjugate or serogroup B meningococcal conjugate vaccines continues to increase, additional reductions in the incidence of meningococcal disease are likely to occur (see Fig. 31.2 ).

From 1990 to 2002 in the United States, mortality rates for meningococcal disease were 0.1 death per 100,000 population per year, with 58% of deaths occurring among individuals younger than 25 years old. Infants had a higher mortality rate than that of individuals in different age groups, but they experienced a decline from 1.3 deaths per 100,000 in 1990 to 0.42 death per 100,000 population in 2002. Meningococcal disease mortality rates increased each year in individuals between the ages of 10 and 19 years, which was followed by a decline in mortality rates in the years following and throughout most of adulthood.
In the United States, in the 1990s, serogroup Y became more common, and the overall incidence of serogroup Y infection increased from 2% during 1989–91 to 37% during 1997–2002. A study of the epidemiology of meningococcal disease in New York City from 1989 to 2000 revealed a threefold decrease in rates of serogroup B disease and almost no cases in children younger than 5 years old. CDC data reveal that 75% of meningococcal infections in individuals older than 11 years of age are caused by serogroups C, Y, and W-135, all of which are included in the currently available conjugate vaccines. An increased risk for contracting meningococcal infection also exists in college students, especially freshmen who reside on campus in dormitories. Following outbreaks of serogroup B meningococcal disease on two college campuses in 2013, two serogroup B conjugate vaccines have been licensed, MenB-FHbp and MenB-4C, in October 2014 and January 2015, respectively, and recommended for certain groups of persons 10 years of age or older who are at increased risk for group B meningococcal disease, as either a two-dose series of Mem-4C or a three-dose series of MenB-FHbp.
The meningococcus is pathogenic only in humans. The carriage rate for N. meningitidis in the civilian population has been estimated at various times to range from 1% to 15%. Carriage rates in military personnel during epidemic periods have been considerably greater. Meningococcal carriers generally are adults (>21 years old) who harbor the organism for months, and meningococcus colonizes the nasopharynx asymptomatically in up to 40% of the adult population.
No correlation has been noted between meningococcal meningitis and crowding within households, but disease seems to be more prevalent in urban than in rural areas. In a civilian population, meningococcal meningitis generally is a disease of children and young adults who have been exposed to an adult carrier, usually in the same family, or to individuals with disease or who are carrying the organism in a daycare setting. The estimated likelihood of severe meningococcal disease in family contacts occurring simultaneously with the first case is 1%. The rate is 1000-fold greater than the risk in the community. The risk of meningitis being acquired in daycare center contacts of children with meningococcal disease is 1 per 1000.
Another change in the epidemiology of meningococcal disease in the United States relates to the increasing number of outbreaks (>10 cases per 100,000 population during 3 months), generally caused by serogroup C. Although these outbreaks account for only 2% to 3% of the total number of cases, they cause tremendous public concern and anxiety, which frequently result in misunderstanding of the nature of an outbreak by the media and the public.
An otherwise unprecedented outbreak of meningococcal disease occurred in a sixth-grade elementary school classroom. Five children from a class of 24 developed meningococcal meningitis. In addition, two siblings of one of the index patients also developed meningococcal infection. Detailed epidemiologic investigation suggested that close contact in the classroom (nose-to-nose distances of ≤34 inches) correlated with an increased rate of carriage of N. meningitidis and with an increased risk for development of invasive meningococcal disease.
Major outbreaks of meningococcal meningitis have occurred worldwide. In the late 1980s and early 1990s, major epidemics of meningococcal meningitis caused by a specific clone (III-1) of serogroup A N. meningitidis occurred throughout sub-Saharan Africa. The origins of a pandemic spread of clone III-1 were traced to epidemics in Asia in the early 1980s, with spread through the Near East. An outbreak occurred during the annual pilgrimage to Mecca in 1987, with pilgrims carrying clones back to their countries of origin, including the United States and the United Kingdom. Epidemics of closely related strains of clone III-1 serogroup A meningococcal meningitis occurred in the Sudan, Ethiopia, and Chad in 1988 and in Kenya in 1989. A report in 1992 described an epidemic of clone III-1 in the Central African Republic in an area traditionally outside the “meningitis belt.” These epidemics generally begin during the dry season and decline at onset of the rainy season. According to the World Health Organization (WHO), “in major African epidemics, attack rates range from 100 to 800 per 100,000 population, but individual communities have reported rates as high as 1000 per 100,000.” Major outbreaks have occurred in Brazil, in Finland, and at multiple sites in Africa. A clonal outbreak of serogroup W135 (ET-37ST-11 clonal complex) occurred in 2000 among Hajj pilgrims returning to Europe from Saudi Arabia. In 2001, outbreaks with serogroup W135 also occurred in Niger and Burkina Faso.
Shifts within a community or within a country as a whole from one serogroup to another also are associated with an increased incidence of disease for several years after the new serogroup is introduced into the community. The mechanisms underlying the changing patterns of meningococcal serogroups that cause diseases are unknown.
Protection from meningococcal infection depends on a functional complement system as well as the humoral antibody response. The serum bactericidal activity assay (SBA), which measures killing of N. meningitidis in the presence of specific antibodies and addition of complement is the standard immunologic correlate of protection. SBA titers of 4 or higher with the use of human complement (hSBA) or 8 or higher with the use of rabbit complement (rSBA) are considered protection against subsequent diseases. Meningococcal infections occur more frequently in patients with a deficiency of the terminal components (C5–C9) of the complement system, and the risk for developing meningococcal infection in persons with deficiency of complement factor C5–C9 is approximately 600-fold higher compared with the general population. An increased risk for contracting meningococcal meningitis also has been reported in individuals with an inherited deficiency of C9 and with properdin deficiency. Individuals with a complement-depleting underlying illness also are at particular risk for acquiring invasive diseases. Screening for complement deficiency in pediatric patients with meningococcal disease is recommended.
Epidemiology of Haemophilus influenzae Meningitis
The most dramatic change in the epidemiology of bacterial meningitis since the advent of antibiotics has occurred in the past two decades as a result of licensure of conjugate vaccines against H. influenzae type b ( Fig. 31.3 ). The first vaccine available was H. influenzae type b capsular polysaccharide (polyribosylribitol) phosphate [PRP], which was licensed in 1985 for use in children 18 to 59 months of age. Subsequently, vaccines with improved immunogenicity for children of younger ages were developed by covalently linking the capsular polysaccharide with protein antigens. In 1990, the first conjugate, PRP diphtheria CRM 197 protein conjugate (HbOC), was approved for infant use. In 1991, the Advisory Committee on Immunization Practices (ACIP) and the American Academy of Pediatrics (AAP) recommended universal infant immunization starting at 2 months of age with either HbOC or PRP-meningococcal protein conjugate (PRP-OMP) vaccines. Currently, two different conjugate vaccine preparations are available in the United States alone or in combination with other vaccines: PRP-OMP and PRP-T (tetanus toxoid). One Hib-MenCY-TT is licensed for use in children aged 6 weeks through 18 months at increased risk of meningococcal disease (e.g., complement component deficiencies, functional or anatomic asplenia, outbreaks).

Before universal vaccination was initiated, the incidence of H. influenzae meningitis varied worldwide. The incidence of H. influenzae meningitis in Scandinavian children younger than 5 years averaged 16 to 28 cases per 100,000 children from 1975 to 1984. The incidence of H. influenzae type b meningitis in three Pacific Island countries was 70 to 84 cases per 100,000 children younger than 5 years in 2003 and 67 to 158 cases per 100,000 in Indonesian children younger than 5 years during 1998–2002. The incidence of H. influenzae meningitis in The Netherlands was 22 cases per 100,000 children younger than 5 years.
The incidence of H. influenzae meningitis is markedly higher in nonindustrialized populations. Before the vaccination era, Alaskan Eskimos had an annual incidence of 282 cases per 100,000 children younger than 5 years of age. The Navajo and White Mountain Apache Native Americans had a much higher incidence compared with that of Native Americans in other regions of the United States, and the incidence among Australian Aboriginals and among certain African populations, such as those in Gambia and Senegal, was 3 to 10 times higher than that of populations in the United States and Europe. The incidence of H. influenzae meningitis was decreased in Gambia from more than 60 cases per 100,000 children to none per 100,000 in children younger than 5 years after implementation of H. influenzae type b vaccination in May 1997. After the WHO recommendation in November 2006, H. influenzae type b vaccine was included routinely in the immunization schedule, and the impact of H. influenzae type b vaccination in prevention of H. influenzae type b meningitis has been demonstrated throughout the world. A resurgence of invasive H. influenzae type b disease was seen in the absence of a booster dose in the United Kingdom. Subsequently, a booster campaign with H. influenzae type b vaccine was introduced that offered one dose to all children aged 6 months to 4 years of age, and it resulted in decreased invasive H. influenzae type b disease among older children and adults in the United Kingdom.
Good epidemiologic data concerning the incidence of H. influenzae infection are, however, lacking in many other areas of the world. Measuring the extent of H. influenzae disease often is difficult, time-consuming, and expensive. In an attempt to decrease complexity, cost, and time required to collect data concerning the incidence of H. influenzae infection, the Haemophilus influenzae type b Rapid Assessment Tool was developed. Increasing numbers of countries are using such tools to estimate the local H. influenzae disease burden and to help determine the need for implementation of a local vaccination program.
In one study, the incidence of H. influenzae meningitis was 3.5-fold higher in blacks than in whites, but this distribution of cases seemed to be related more closely to poverty than to race. In whites, no increase in incidence occurred in overcrowded households, but the incidence was higher in rural than in urban areas, which allowed the postulation that the increased incidence in rural whites and in blacks was related to lack of access to early medical care. The Haemophilus influenzae Study Group noted that the number of cases of H. influenzae meningitis in children younger than 5 years reported through the National Bacterial Meningitis Reporting System began declining rapidly in 1988. In another CDC surveillance project conducted from 1989 to 1997, the race-adjusted incidence of invasive H. influenzae type b disease among children younger than age 5 years declined from 34.0 to 0.4 per 100,000, a 99% reduction (see Fig. 31.3 ).
Serotype replacement has been seen with an increase in incidence of non–type b cases. In a statewide surveillance done in Alaska from 1983 to 2011, no H. influenzae type a disease was identified before 2002, but from 2002 to 2011, 32 cases were identified, whereas from December 2009 to December 2011, 15 cases were identified. A report from Active Bacterial Core Surveillance sites from 1998 to 2007 indicated that small increases in the incidence of serotypes a, e, and f were observed, and the largest of these increases was in serotype f and was primarily among adults aged 18 years or older.
The overall incidence of H. influenzae meningitis decreased by 35% between 1998 and 1999 and 2006 to 2007, from 0.12 case per 100,000 population to 0.08 case per 100,000 population (see Table 31.2 ); 9.4% of the cases were caused by serotype b. The epidemiology of invasive H. influenzae disease in the post– H. influenzae type b vaccine era seems to be shifting from H. influenzae type b in children toward non–type b strains in older individuals. For example, among adult cases of H. influenzae meningitis, a majority (73.8%) of the H. influenzae isolates were not typable, whereas serotypes e and f were the most common serotypes identified (with each found in 11.5% of cases). The case-fatality rates were significantly higher among adults with typable H. influenzae than among cases from nontypable H. influenzae (18.8% vs. 2.2%; P = .02).
National trends in mortality from H. influenzae meningitis were evaluated from 1980 to 1991. From 1980 through 1987, mortality rates from H. influenzae meningitis decreased an average of 8.5% a year, from 1.72 per 100,000 children in 1980 to 0.94 per 100,000 children in 1987. From 1988 to 1991, mortality rates decreased an average of 48% a year, with a death rate of 0.11 per 100,000 children in 1991. The estimated case-fatality rate for H. influenzae meningitis was 3.3% from 1980 to 1987 and 2.3% from 1988 to 1991. In comparison, mortality rates from S. pneumoniae meningitis decreased 10% annually from 1980 to 1987 and 3% annually from 1988 to 1991. Similarly, mortality rates from meningococcal meningitis decreased by 13% annually from 1980 to 1987 and 12% annually from 1988 to 1991.
The dramatic decrease in the incidence of H. influenzae meningitis has been affected by several factors. The precipitous drop that occurred shortly after universal immunization of infants strongly suggests that this practice has affected the epidemiology of this disease. Conjugate vaccination protects against nasopharyngeal colonization, decreasing the carriage rate of H. influenzae type b and diminishing the reservoir for transmission and providing immunity from infection. These factors would also lessen the likelihood of development of infection in unvaccinated populations through herd immunity. Other changes in medical practice, such as the widespread use of outpatient antibiotics and improvements in supportive care, may have some effect, as shown by the decrease in case-fatality rates for H. influenzae meningitis, the steady decrease in mortality rates before vaccination, and the decrease in instances of meningococcal and pneumococcal disease.
The risk for spread of severe H. influenzae illness among household contacts of patients with H. influenzae meningitis was studied prospectively in 19 states. The risk in children younger than 1 year of age was 6%; in children younger than 4 years, 2.1%; and in children younger than 6 years, 0.5%. The risk for H. influenzae disease occurring in household contacts younger than 6 years is similar to the risk for secondary meningococcal disease occurring in all household contacts, indicating a need for effective antimicrobial prophylaxis. Spread of H. influenzae disease in daycare centers also is well documented. The precise risk for acquiring secondary H. influenzae type b infection in daycare centers is unclear.
Recurrent invasive H. influenzae type b disease has been reported. Detailed studies suggest that age and high incidence of disease alone are not the only factors contributing to the recurrence of the disease. Patients who have recurrent disease caused by H. influenzae type b may represent a subset of a population with unusual disease susceptibility. In addition, children who develop invasive infection caused by H. influenzae type b after receiving appropriate conjugate vaccine frequently have subnormal immunoglobulin concentrations and should undergo immune evaluation.
Pathophysiology
Organisms Encountered
Almost all microorganisms that are pathogenic to humans have the potential to cause meningitis, but a relatively small number of pathogens (i.e., group B Streptococcus , E. coli, L. monocytogenes, H. influenzae, S. pneumoniae, and N. meningitidis ) account for most cases of acute bacterial meningitis in neonates and children. The reasons for this association remain incompletely understood. In immunocompromised hosts, infection with other organisms may occur. The specific organism sometimes may be predicted on the basis of the type of deficit that is present in the host.
Routes of Infection
Bacterial infection of the normally sterile leptomeningeal spaces can occur from hematogenous spread, direct extension from paranasal and dental infections as well as skull base fracture, direct implantation (e.g., after surgery, cochlear implant), or rarely secondary to infections in the epidural or subdural spaces. Bacterial meningitis in children with otitis media generally follows bacteremia, although direct invasion of the meninges may occur as a complication of otitis media.
The meninges are seeded with microorganisms during a bacteremic period. Bacterial meningitis has been induced in experimental animals (e.g., infant rats and monkeys ) after intranasal inoculation of H. influenzae type b. Bacteremia developed hours before meningitis could be detected histologically, a finding that supports the concept that meningitis follows hematogenous dissemination from nasopharyngeal colonization or infection. Marginating bacteria could be detected by fluorescent staining initially in the lateral and dorsal longitudinal (sagittal) sinuses and subsequently spread to the leptomeninges. Several lines of evidence from human cases of group B Streptococcus and E. coli meningitis and animal models of experimental hematogenous meningitis, however, reveal that cerebral capillaries are the portal of entry into the brain for circulating group B Streptococcus and E. coli, and bacterial penetration into the brain was demonstrated without exhibiting increased blood-brain barrier (BBB) permeability and accompanying host inflammatory cells.
Meningitis can also develop after bacterial invasion from a contiguous focus of infection, as in infection of the mastoid or paranasal sinuses or as a complication of otitis media. For example, S. pneumoniae has been shown to enter the CNS through a nonhematogenous route in experimental animals after intranasal infection and otitis media. Fracture through the paranasal sinuses as a result of head trauma may precede development of meningitis caused by S. pneumoniae and H. influenzae, which may be recurrent. Direct invasion also may occur in individuals with dermoid sinus tracts or meningomyeloceles, when a direct communication between the skin and the meninges is present. In this setting, infection usually is produced by organisms found on the skin. Recurrent meningitis has been reported in patients with basic ethmoidal encephaloceles or a congenital defect in the stapedial footplate. Surgical obliteration of the fistula with temporal muscle and fascia prevented the recurrence of meningitis. Meningitis also may develop subsequent to osteomyelitis of the skull or vertebral column. Rarely meningitis may develop in the normal host with commensal microorganisms after having a tooth extracted or getting dental fillings.
Neurosurgical procedures, particularly procedures designed for diversion of CSF in children who have hydrocephalus, may lead to development of meningitis. Chemical meningitis also may occur after neurosurgical procedures, especially procedures involving the posterior fossa. In these patients, evidence of inflammation develops rapidly with elevation of temperature occurring on the first postoperative day.
Infection of the CNS may result from environmental contamination or manipulation. Meningeal infection may be acquired in utero transplacentally or during delivery through contact with the cervix or vaginal canal, which may be colonized with a variety of organisms, particularly group B Streptococcus , E. coli, and L. monocytogenes. A neonate, a patient with cystic fibrosis, or a burned child may develop septicemia and meningitis as a result of persistent heavy colonization with Staphylococcus aureus. A humidified atmosphere promotes the colonization and growth of such organisms as Serratia marcescens and Pseudomonas aeruginosa. Placing a patient in this setting leads to an increased frequency of infection with these organisms. Indwelling catheters can predispose a patient to infection by bacterial (and fungal) organisms that generally are of low virulence in the normal host.
Pathogenesis
Most cases of bacterial meningitis develop through four steps: (1) colonization of the mucosal membranes such as upper respiratory or gastrointestinal tract, (2) invasion of the blood from the mucosal colonization, resulting in a threshold level of bacteremia, (3) traversal of the blood-brain barrier, and (4) inflammation of the meninges and brain. Less commonly, infection of the leptomeninges can occur by contiguous spread or hematogenous dissemination from another remote site.
Mucosal Colonization
Most meningitis-causing bacteria colonize the mucosal membranes of the nasopharynx ( S. pneumoniae, N. meningitidis, and H. influenzae ) and gastrointestinal tract ( E. coli and L. monocytogenes ). This attachment is mediated by specific microbial cell surface components and affected by co-colonization with other microorganisms and host immune response.
Several pneumococcal factors have been shown to enhance nasopharyngeal colonization and binding to nasopharyngeal epithelium: pneumococcal phosphorylcholine, IgA1-protease, capsular switching, pneumolysin, pneumococcal surface protein (Psp) C (also known as choline binding protein [CbpA]), pneumococcal surface adhesin A (PsaA), pneumococcal adherence and virulence factor A (PavA), neuraminidase A (NanA), and pili. Additionally, prior exposure to influenza virus has been associated with increased colonization and secondary invasive pneumococcal disease. Pneumococcal phosphorylcholine binds to the platelet-activating factor (PAF) receptor on activated host epithelial cells, whereas the PspC binds to epithelial polymeric immunoglobulin receptor (pIgR). The pIgRs are expressed in a decreasing gradient from the upper to lower respiratory tract, whereas the opposite pattern of expression is observed for the PAF receptor, suggesting that pIgR serves mainly as a pneumococcal receptor for the nasopharynx but the PAF receptor facilitates attachment and invasion of the pulmonary epithelium.
N. meningitidis strains use IgA1 protease, pili, and outer membrane proteins Opa, Opc, and porin A and B for binding to cell surface receptors on nasopharyngeal mucosal cells and apparently are transported across specialized cells within phagocytic vacuoles. Human embryonic antigen cell adhesion molecules (CEACAMs or CD66) are cell-surface molecules in nasopharyngeal epithelial cells that interact with meningococcal Opa proteins.
Bacteremia
When in the bloodstream, the common meningitis-causing organisms ( S. pneumoniae, H. influenzae, N. meningitidis, E. coli K1, and group B Streptococcus ) are capable of evading host defense mechanisms, which involves capsular polysaccharides and other bacterial factors, resulting in a high level of bacteremia ( Table 31.4 ).
Pathogens | Bacterial Factors |
---|---|
E. coli | Capsular polysaccharides, O-LPS, type 1 fimbriae, NlpI, SslE |
Group B streptococcus | Capsular polysaccharides, C5a peptidase?, SodA |
S. pneumoniae | Capsular polysaccharides, pneumolysin |
N. meningitidis | Capsular polysaccharides, LOS, factor-H binding protein, PorA |
Several studies of humans and experimental animals suggest a relationship between the magnitude of bacteremia and development of meningitis due to E. coli, group B Streptococcus , H. influenzae type b, and S. pneumoniae. For example, a significantly higher incidence of E. coli meningitis was noted in neonates who had bacterial counts in blood greater than 10 3 colony-forming units (CFU)/mL (6 of 11; 55%) compared with those with bacterial counts less than 10 3 CFU/mL (1 of 19; 5%). Similarly, meningitis due to S. pneumoniae was observed more frequently in patients whose bacterial counts in blood were greater than 1 × 10 2 to 5 × 10 2 CFU/mL (6 of 7; 86%) than in those with fewer bacterial counts in blood (2 of 50; 4%). For H. influenzae type b, the level of bacteremia is around 10 2 CFU/mL of blood. Consistent with these clinical findings, a high degree of bacteremia was a primary determinant for meningeal invasion of experimental hematogenous meningitis caused by E. coli, group B Streptococcus , H. influenzae type b, and S. pneumoniae. All patients with N. meningitidis meningitis had N. meningitidis detected in their blood, thus also suggesting a relationship between bacteremia and development of meningitis caused by N. meningitidis. The basis for requiring a threshold level of bacteremia for bacterial penetration into the brain is currently unknown.
Bacterial Traversal of the Blood-Brain Barrier
The bacteria in the bloodstream subsequently traverse the BBB, most likely at the cerebral capillaries ( S. pneumoniae, N. meningitidis, E. coli K1, and group B Streptococcus ) and choroid plexus ( H. influenzae ). Meningitis-causing bacteria cross the BBB transcellularly, paracellularly, and/or by means of infected phagocytes (the so-called Trojan horse mechanism). Transcellular traversal of the BBB has been demonstrated for most meningitis-causing bacteria in infants and children, including group B Streptococcus , E. coli, and S. pneumoniae, whereas N. meningitidis has been shown to utilize transcellular and paracellular mechanisms for traversal of the BBB, and L. monocytogenes penetration of the BBB has been shown to utilize transcellular and Trojan horse mechanisms.
Recent studies, however, have shown that a high degree of bacteremia is necessary, but not sufficient, for the development of meningitis and that bacterial binding to and invasion of the BBB is a prerequisite for penetration into the brain. This concept was well demonstrated with E. coli K1, where mutants deleted of the E. coli factors contributing to binding to and invasion of the BBB were significantly less able to traverse the BBB than the parent strain despite causing similar levels of bacteremia.
Bacterial traversal of the BBB requires specific bacterial factors and their interactions with host factors ( Table 31.5 ), although the complete information on microbial-host factors contributing to penetration of the BBB is currently lacking for most meningitis-causing bacteria.
Pathogens | Bacterial Factors | Host Receptors | Host Signaling Molecules |
---|---|---|---|
E. coli | Type 1 fimbriae, OmpA, flagella, NlpI, IbeA, IbeB, IbeC, CNF1, AslA | CD48, gp96, 37LRP, Caspr1 | FAK, paxillin, PI3-K, Src kinase, Rho GTPases, cPLA 2 α, 5-lipoxygenase, cyteinyl leukotrienes, STAT3, PKCα, VEGFR1, EGFR |
Group B streptococcus | Lmb, FbsA, IagA, HvgA, PilA, LTA, β-hemolysin, ScpB, αC protein | Integrin, GAGs | FAK, Rho GTPases, cPLA 2 α, 5-lipoxygenase, and cyteinyl leukotrienes |
L. monocytogenes | InlB | gC1qR, Met, gp96 | PI3-K |
S. pneumoniae | Phosphorylcholine, PspC (CbpA), pneumolysin, NanA | PAF receptor, 37LRP | β-Arrestin, MAPK |
N. meningitidis | Type IV pili, PilC, Opa, Opc, NadA | CD46, CD66, integrin, 37LRP, β 2 -adrenoreceptor | JNK1 and 2, β-arrestin |
Recent studies have also shown that meningitis-causing bacteria exploit specific host cell signaling molecules for promoting host cell actin cytoskeleton rearrangements and bacterial invasion of the BBB (see Table 31.5 ). E. coli invasion of the BBB involves focal adhesion kinase (FAK), paxillin, phosphatidylinositol 3-kinase (PI3-K), Src kinase, Rho guanosine triphosphates, signal transducers and activators of transcription (STAT)3, cytosolic phospholipase A 2 α (cPLA2α), 5-lipoxygenase, cyteinyl leukotrienes, and protein kinase C, whereas group B Streptococcus invasion of the BBB is independent of Src activation and L. monocytogenes invasion of the BBB is independent of FAK and cPLA 2 α. N. meningitidis has been shown to invade the BBB involving type IV pili, PilC, NadA, Opa, and Opc proteins. The detailed information on how meningitis-causing bacteria exploit host cell signaling molecules for invasion of the BBB, however, remains incompletely understood. For example, the invasion of the BBB by unencapsulated meningococcus is mediated by Opc binding to fibronectin, which anchors the bacteria to the integrin receptor on the BBB. However, in the bloodstream, N. meningitidis is encapsulated and the in vivo relevance of Opc-fibronectin–mediated binding to integrin requires clarification, and type IV pili have been shown to contribute to paracellular penetration of N. meningitidis across the BBB. S. pneumoniae has been shown to invade the BBB via complex interaction of phosphorylcholine and CbpA proteins with receptors on activated endothelial cells.
The mechanisms involved in entry of meningitis-causing bacteria into the BBB differ from those involved in the inflammation occurring in response to those bacteria. This concept has been demonstrated for E. coli, group B Streptococcus , and N. meningitidis. N. meningitidis invasion of the BBB involves c-Jun kinases 1 and 2 (JNK1 and JNK2), but the release of interleukin (IL)-6 and IL-8 from the BBB involves the p38-mitogen–activated protein kinase (MAPK) pathways.
BBB Dysfunction and Intracranial Inflammation
Once bacteria enter the CSF, because of insufficient opsonic and phagocytic activity in the CSF, organisms multiply, liberating bacterial cell wall or membrane components (lipopolysaccharide, lipoteichoic acid, peptidoglycan, lipoproteins, bacterial toxins), and induce release of inflammatory mediators and toxic compounds, which leads to CSF pleocytosis and increased BBB permeability, the hallmarks of bacterial meningitis. However, pleocytosis and increased BBB permeability can develop independently of each other in some patients with bacterial meningitis (see later discussion).
Complement and opsonic proteins either are found at very low concentrations or are absent within normal CSF, and the CSF is devoid of the factors required for enhancing bacterial killing. When bacteria first invade the meninges, the lack of complement and opsonic proteins within the sanctuary of the CNS may permit the bacteria to multiply unrestrained for some time.
The specific pathophysiologic changes in bacterial meningitis are the result of the bacterial products and the inflammatory response of the host to those products ( Fig. 31.4 ). Initial bactericidal antibiotic therapy results in a rapid release of bacterial products, such as lipopolysaccharides (endotoxins), teichoic acid, and peptidoglycans. For gram-positive bacterial meningitis, lipoteichoic acid and peptidoglycans are the bacterial surface elements that induce inflammation. The threshold concentration that triggers inflammation is approximately 10 5 bacterial cell equivalents of cell wall pieces. For gram-negative meningitis, lipopolysaccharides (endotoxins) are the major inflammatory components, with peptidoglycan serving as an important cofactor. The inflammatory threshold is approximately 2 pg of endotoxin, or approximately 10 5 bacterial cell equivalents.

Immune activation in the CNS is initiated by the recognition of different bacterial pathogen-associated molecular patterns (PAMPs). Major pattern recognition receptors (PRRs) involved in initial sensing of bacteria in the CNS include Toll-like receptors (TLRs) 2, 4, 5, and 9 and Nod-like receptors (NLRs), which are involved in triggering an intracellular signaling pathway that leads to activation of transcription factors such as nuclear factor-κB (NF-κB) and the subsequent gene transcription of proinflammatory cytokines and chemokines such as tumor necrosis factor-α (TNF-α), IL-1, and IL-8.
Cytokines and chemokines seem to be the primary drivers of the inflammatory response. The following cytokines and chemokines are involved in the inflammatory response noted in bacterial meningitis: IL-1, IL-3, IL-4, IL-6, IL-8, IL-10, IL-12, IL-18, interferon-γ, macrophage inflammatory protein, transforming growth factor-β, and TNF-α.
TNF-α and IL-1β seem to be key mediators in initiation of meningeal inflammation. Both proteins stimulate vascular endothelial cells to induce adhesion and passage of leukocytes into the CNS and trigger inflammatory processes. Astrocytes and microglia are capable of producing TNF-α. TNF-α concentrations are elevated (1) in CSF but not in serum; (2) in animal models of bacterial meningitis; and (3) in patients with bacterial meningitis caused by H. influenzae, N. meningitidis, S. pneumoniae, and Streptococcus agalactiae, but not in patients with culture-proven viral meningitis. TNF-α levels can be correlated with meningeal inflammation and severity of disease, but lack of TNF-α in the animal model is associated with increased mortality and stronger deficits in spatial memory.
IL-1β can be detected in infants and children with bacterial meningitis, and its presence is correlated significantly with CSF inflammatory abnormalities, TNF-α concentrations, and adverse outcome. The role of IL-1β in bacterial meningitis, however, is inconsistent. Levels of IL-1β were not associated with the degree of the BBB disruption in patients with bacterial meningitis.
The chemokines are a superfamily of small chemoattractant cytokines that play an important role in the initiation and modulation of inflammation in bacterial meningitis. IL-8 is one of the well-characterized chemokines in bacterial meningitis and was found to be chemotactic for neutrophils in the CSF of patients with bacterial meningitis.
Complement factors are upregulated in bacterial meningitis. The activated complement cascade in CSF, acting on upregulated complement receptors on brain cells, potentially mediates direct brain damage. Complement factors also are chemoattractants that enhance CSF leukocytosis and indirectly produce brain damage in patients with bacterial meningitis.
Intracisternal administration of antibodies to TNF-α and IL-1β decreased pleocytosis and BBB permeability in response to intracisternal injection of H. influenzae and S. pneumoniae, and blockade of pleocytosis was beneficial in reducing BBB permeability in experimental meningitis induced by intracisternal inoculation of S. pneumoniae, H. influenzae, and N. meningitidis. In contrast, intracisternal inoculation of H. influenzae and S. pneumoniae resulted in increased BBB permeability in both normal and leukopenic animals, indicating that increased BBB permeability in bacterial meningitis can occur in the absence of pleocytosis. However, no change in BBB permeability shown as normal CSF protein concentrations and absence of pleocytosis after bacterial entry into the CNS usually reflect an early stage of bacterial meningitis.
Neuronal Injury
Neuronal damage is caused by vasculitis; focal ischemia; increased intracranial pressure; cytotoxic, vasogenic, and interstitial edema; transmigrating leukocytes; stimulation of glial cells and astrocytes; cortical necrosis and hippocampal neuronal loss stemming from an inflammatory response to bacteria or bacterial products (bacterial cell wall, teichoic and lipoteichoic acids, lipopolysaccharides, lipoproteins, bacterial DNA); and direct effects of bacterial toxins in the CNS. The various host factors contributing to neuronal injury are shown in Fig. 31.4 .
Reactive oxygen species (superoxide and hydrogen peroxide) are secreted by TNF-α–stimulated macrophages, including brain microglia, and leukocytes. Hydrogen peroxide induces extensive neuronal damage. Nitric oxide also can be induced in brain cells in response to bacterial products. Nitric oxide and superoxide radicals react to form peroxynitrite anion, which decomposes and forms nitrogen dioxide, hydroxyl radicals, and strong oxidant compounds. Peroxynitrite seems to be an important neuronal toxin. Neuronal damage caused by reactive oxygen species and reactive nitrate species occurs via at least two separate pathways—via lipid peroxidation and subsequent cell membrane instability and via DNA fragmentation and subsequent activation of poly(adenosine diphosphate ribose) polymerase (PARP) and cellular energy depletion. Reactive oxygen species also activate NF-κB, a transcription factor affecting transcription of cytokines and chemokines, and peroxynitrite initiates activation of caspases.
In addition, macrophages secrete excitatory amino acids, such as glutamate, which potentially kill N -methyl- d -aspartate receptor–positive cells, and glutamate concentrations in the CSF are shown to be increased in patients with bacterial meningitis.
Meningitis causes damage in the cortex and the hippocampal region via different mechanisms. Cortical damage is mainly via cellular necrosis surrounded by an area of caspase-3–dependent apoptosis. Damage in the hippocampus is mediated not only via the classic caspase-3–dependent pathway but also by caspase-independent apoptosis mediated by apoptosis-initiating factor.
The increasing concentrations of chemotactic factors in the subarachnoid space lead to accumulation of large numbers of neutrophils in the CSF, but the growth of bacteria is not slowed significantly by this response. Impaired phagocytosis by neutrophils in the meningeal spaces may be related to weak activity in fluid medium, lack of complement activity and opsonization, and poor penetration of IgM and IgG through the BBB, even during acute H. influenzae and S. pneumoniae meningitis. The contribution of leukocyte entry into the CSF to bacterial meningitis remains inconsistent. Blockage of leukocyte entry into the CSF was associated with poorer prognosis (likely secondary to an increase in bacterial counts in the blood) but did not affect the risk for brain damage, whereas inhibition of leukocyte migration or of products released by leukocytes is shown to interfere with the pathogenesis of bacterial meningitis.
Bacterial cell wall fragments, endotoxin, or both also contribute to vascular permeability. In experimental E. coli meningitis, the CSF endotoxin concentration increased markedly after treatment with β-lactam antibiotics. This increase was associated with an increase in brain water content. This effect could be blocked by polymyxin or a monoclonal antibody, both of which inactivate endotoxin.
The inflammatory and vascular events described earlier act synergistically to produce the clinical symptoms and long-term sequelae that are noted in patients with bacterial meningitis. Altered vascular permeability leads to vasogenic edema, inflammatory and electrolyte changes lead to cytotoxic edema, and alterations in production and absorption of CSF lead to interstitial edema. The cytokines also trigger increased cerebral blood flow and further formation of edema, resulting in increased intracranial pressure. The increased intracranial pressure and vasculitis lead to a subsequent decrease in cerebral blood flow, and maintenance of an appropriate cerebral perfusion pressure is crucial to maintaining cerebral blood flow. Activation of the coagulation cascade predisposes the patient to venous, microvascular, and, rarely, arterial thrombosis. Direct neurotoxic damage by inflammatory cells also may contribute to the neuropathologic changes seen in bacterial meningitis.
Studies in experimental animals and human autopsy cases of bacterial meningitis reveal that neuronal injury occurs mostly as apoptosis in the dentate gyrus of the hippocampus formation and as necrosis in the neocortex and the CA1-4 region of the hippocampus. In other regions of the brain, neurons die with necrotic morphology. Not only cellular destruction but also proliferation of neuronal progenitor cells has been observed in patients after bacterial meningitis. Increased neural progenitor cell proliferation and differentiation were also observed after experimental bacterial meningitis. These findings suggest an endogenous potential of cell renewal in the hippocampal formation in bacterial meningitis. It remains to be determined whether stimulation of endogenous neurogenesis can be used as a potential option in survivors of meningitis.
In the adult brain, neural progenitor cells maintain the ability to proliferate and differentiate into new neurons. The progenitor cells are located predominantly in the subgranular zone of the dentate gyrus and the subventricular zone of the lateral ventricle. In the subgranular zone, the stem cells proliferate and differentiate into progenitor cells. The newly formed cells subsequently migrate into the granular layer of the dentate gyrus, differentiate into mature, functional neurons, and become integrated into the hippocampal network. The hippocampus is therefore potentially equipped for repair. However, because neurofunctional sequelae of bacterial meningitis related to hippocampus function persist throughout childhood and adulthood, the capacity of self-repair seems insufficient to compensate for the brain damage. It remains to be determined whether the regenerative capacity of the hippocampus is compromised by bacterial meningitis.
Factors Predisposing the Host to Bacterial Meningitis
Factors that predispose the host to the development of bacterial infection in other sites also predispose the host to the development of bacterial infection of the CNS. A strong interrelationship exists among factors relating to the host, the organism, and the environment with regard to the pathogenesis and outcome of meningitis. Although presented separately, they must be considered a complex interplay of factors that leads to infection.
An increased incidence of bacterial meningitis is observed in the very young; boys are affected more frequently than girls, and the severity of disease also is increased in these groups. A neonate is predisposed to development of septicemia and meningitis by factors that reflect physiologic deficiencies or immaturity of host defense mechanisms, including (1) decreased phagocytic and bactericidal activity of polymorphonuclear leukocytes, (2) defects in the response of neonatal leukocytes to chemotactic factors, (3) a deficiency in the capacity of leukocytes to support opsonization, and (4) defects in microtubular length and number that decrease the motility of the neonatal leukocytes compared with leukocytes from older children. Deficiencies in serum complement components (C1q, C3, and C5), low levels of serum properdin, and low concentrations of serum IgM and IgA have been documented. Despite transplacental acquisition of IgG, antibodies against specific infective agents may be lacking. The precise age at which each of these factors reaches the concentration and functional activity noted in older children and adults is unclear and undoubtedly varies from individual to individual. In part, meningitis in children aged 1 month to 1 year may reflect qualitative or quantitative differences of the inflammatory and immunologic responses compared with older children.
The increased risk for development of meningitis in the normal host with less than completely mature immunologic and inflammatory responses to infection may be attributable to age alone. This factor is exemplified in the report, where the risk for recurrent bacteremia was studied in young children. Within 18 months of having bacteremic illness, none of 42 children older than 24 months of age had a documented additional episode of bacteremia or systemic infection. However, 15 of 135 children (11%) younger than age 24 months at the time of the initial bacteremic disease had at least one additional documented bacteremic illness. Of these 15 children, 14 contracted both infections before reaching 2 years of age. Seven of these 15 children had meningitis. Only 2 patients had documented congenital or hereditary disorders of immunoglobulin or complement concentration or function.
There is increasing evidence that genetic polymorphisms in the inflammatory response, complement system, coagulation, and fibrinolysis pathways influence the genetic predisposition and outcome in bacterial meningitis. A genetic determination for the predilection of some normal children for the development of bacteremia and meningitis has been suggested. The ability of the host to produce, within the CSF, IL-12 and TNF-α–induced interferon-γ is important in the natural immunity to various microorganisms that may cause meningitis.
Deficiencies and polymorphisms in the PRR downstream signaling cascade have been associated with invasive pneumococcal meningitis. IL-1 receptor–associated kinase 4 (IRAK-4) is an intracellular kinase that is involved in signal transduction in the TLR pathways, and MyD88 is a general adaptor molecule for TLR signaling. Individuals with IRAK-4 and MyD88 deficiency are at a high risk for developing pneumococcal meningitis, with increasing mortality. IRAK-4 and MyD88, however, appear to be important at a young age because no fatal disease after 8 years of age and no invasive bacterial infection after 14 years of age have been reported.
Congenital or acquired abnormalities of the immune system may predispose the host to the acquisition of bacterial infections. Congenital deficiency of the three major immunoglobulin classes may predispose the host to the acquisition of severe bacterial infection. Congenital defects of thymic-dependent, small lymphocyte function or combined T- and B-cell defects are detrimental to host defense. A deficiency of CD4 + helper-inducer T cells in patients with bacterial meningitis has been reported and may contribute to the impaired antibody synthesis to bacterial capsular polysaccharides in this disease. Multiple studies have shown that deficiencies of various components of the complement system and increased consumption or loss of complement have been associated with increased risk for development of bacterial meningitis caused by encapsulated organisms.
In analysis of 103 children with invasive pneumococcal disease including meningitis, the proportion of primary immunodeficiencies was significantly higher in children older than 2 years of age than in younger children (26% vs. 3%; P < .001), suggesting that immunologic investigations are warranted in children with pneumococcal meningitis, particularly in those older than 2 years.
An increased incidence of overwhelming infection, including meningitis, occurs after splenectomy, but the likelihood of developing such infection depends on the age of the child at the time of splenectomy, the time since splenectomy, and the original indication for splenectomy. Congenital asplenia or polysplenia also has been associated with an increased incidence of septicemia and meningitis caused by S. pneumoniae, H. influenzae type b, and gram-negative enteric microorganisms. Children with sickle-cell disease and other hemoglobinopathies have meningitis caused by S. pneumoniae, H. influenzae, and Salmonella spp. more frequently than do normal children.
Children with malignant neoplasms with or without neutropenia seem to be susceptible to developing meningitis caused by organisms of low virulence that pose a minimal threat to healthy children, presumably because of abnormalities in immunologic function. Decreased production of normal immunoglobulins, delayed and defective antibody responses to antigenic stimuli, production of abnormal immunoglobulins, depression in the clearance mechanisms of the reticuloendothelial system, and depression of cellular immunity have been documented in children with malignant neoplasms involving the reticuloendothelial system. In addition, the use of irradiation or immunosuppressive agents and antimetabolites predisposes the host to the development of infection in the CNS. Attributing the occurrence of bacterial meningitis in this population directly to these agents rather than to the disease for which this therapy has been provided may be difficult. Meningitis occurring after neurosurgical manipulation for tumors of the CNS in non-neutropenic children usually develops within 1 month of the neurosurgery.
Malnutrition also predisposes children and adults to infectious disease. Impaired cellular immune responses, low levels of serum complement, impaired phagocytic activity of neutrophils, and decreased serum concentrations of transferrin have been documented in malnourished children.
Patients with systemic diseases, such as diabetes mellitus, renal insufficiency, adrenal insufficiency, cystic fibrosis, hypoparathyroidism, and exudative enteropathy, have an increased frequency and severity of CNS infections. Some type of underlying condition was noted for 21% (37 of 181) of the children with pneumococcal meningitis in a multicenter study. The most common of these conditions was some disorder of the CNS, which occurred in 16 children (9%). Defective chemotaxis, phagocytosis, and bactericidal function accompany these disorders and may explain in part the increased susceptibility of these individuals to infection.
In the normal host, bacterial infections at sites other than the leptomeninges are associated with an increased incidence of CNS infection. Infection may spread hematogenously to the meninges in children with endocarditis, pneumonia, or thrombophlebitis or by direct extension from sinusitis, mastoiditis, or osteomyelitis of the skull.
An increased risk for the development of pneumococcal meningitis also occurs in children after placement of cochlear implants. The incidence of meningitis caused by S. pneumoniae in patients after receiving cochlear implants was 138.2 cases per 100,000 person-years, which represents a more than 30-fold increase over the age-controlled general population. From 1999 to 2002, some children were implanted with cochlear devices, including a positioner, which were subsequently removed from the market as a result of an associated marked increase in incidence of pneumococcal meningitis. After removing the influence of cochlear devices containing a positioner, the incidence of pneumococcal meningitis among children with cochlear implants was still 16 times higher than that of an age-matched control population.
Pathology
The most detailed account in English of the pathologic changes occurring with meningitis was written in 1948, which included the meningeal, cerebral, and vascular changes in 14 patients who died of H. influenzae infection 14 hours to 76 days after the onset of disease. Although most patients in their series received inadequate treatment (effective antibiotic therapy was unavailable), the pathologic findings they describe differ little from the findings of subsequent reports for patients who died despite administration of antibiotics.
Meningeal exudates of variable thickness may be found ( Fig. 31.5 ). Purulent material is distributed widely but may accumulate around the veins and venous sinuses, over the convexity of the brain, in the depths of the sulci, in the sylvian fissures, within the basal cisterns, and around the cerebellum. The spinal cord may be encased in pus. Ventriculitis (purulent material within the ventricles) has been noted in children who died of their diseases ( Fig. 31.6 ). Subsequent experience suggests that ventriculitis may be a common finding in children with bacterial meningitis who survive, particularly neonates. Loss of the ependymal lining and subependymal gliosis may be seen. In some studies, purulent exudates tended to be thicker over the convexity of the brain in patients with pneumococcal meningitis than in patients with other forms of meningitis.


Vascular and parenchymatous changes have been shown at necropsy. Polymorphonuclear infiltrates extending to the subintimal region of small arteries and veins have been associated with the exudative meningeal process. Thrombosis of small cortical veins associated with necrosis of the cerebral cortex may be noted. Occlusion of one of the major venous sinuses, subarachnoid hemorrhage secondary to necrotizing arteritis, and necrosis of the cerebral cortex in the absence of identifiable thrombosis of small vessels rarely may be observed. Reactive microglia and astrocytes may be identified in the cerebral cortex, particularly subadjacent to regions of heavy subarachnoid exudate. Because no bacteria are found in the cerebral cortex, these pathologic changes should be viewed as a noninfectious encephalopathy. “Toxic or circulatory factors” were suggested as possible causes and hypoxia and fever as additional possible causes. An increase in intracranial pressure might interfere with cerebral circulation.
Impaired consciousness, deficits in motor and sensory functions, seizures, and retardation may be observed. Damage to the cerebral cortex, reflecting the effects of vascular occlusion, hypoxia, toxic encephalopathy, bacterial factors, inflammatory mediators, small molecule effectors, or some combination of these factors, provides an adequate explanation for these observations.
Hydrocephalus that develops in patients beyond the newborn period is an uncommon complication of meningitis. Most often, hydrocephalus is communicating and is the result of adhesive thickening of the arachnoid around the cisterns at the base of the brain. Less frequently, the aqueduct of Sylvius or the foramina of Magendie and Luschka are obstructed by fibrosis and reactive gliosis. The ensuing ventricular dilation may be coupled with coexistent necrosis of nervous tissue because of the meningitis itself or because of occlusion of cerebral veins and, rarely, arteries.
Subdural effusions occur frequently during the course of meningitis. The exact pathogenesis is unknown. The high incidence of effusion and the fact that subdural fluid collections may be found early in the course of bacterial meningitis in children suggest, however, that subdural effusions should be considered a concomitant occurrence with meningeal inflammation rather than a complication of the disease. Numerous veins traverse the subdural space, and inflammation of these veins and of the dural capillaries could produce an increase in vascular permeability and loss of albumin-rich fluid into the subdural space. The ratio of albumin to γ-globulin is higher in the subdural fluid of children with meningitis than in serum. When the inflammatory process subsides, formation of fluid generally ceases, but its presence may persist because of a continued transudation through newly formed vessels in the subdural membrane. Subdural empyema, as opposed to subdural effusion, occurs rarely.
Many factors contribute to the increase in intracranial pressure in patients with meningitis. Endotoxin and fragments of the cell wall of gram-positive organisms are capable of inducing the release of IL-1β and TNF-α from macrophages, glial cells, and other sources. These substances, in addition to other ILs and metabolites, affect endothelial cells, affecting the function of the vasculature and its interaction with neutrophils and other inflammatory cells. They also play an important role in the pathogenesis of increased intracranial pressure and cerebral edema in patients with meningitis by altering cerebral blood flow, intracranial blood volume, and permeability of the cerebral vasculature. Experimentally, the main components of the BBB—endothelial cells, astrocyte-end feet, and pericytes—have been found to be sensitive to the effects of such inflammatory mediators. Intercellular junctions, which normally are tight, are open in advanced stages of experimental meningitis, which is associated with an increased permeability to circulating albumin. Pinocytotic vesicles also are noted within the cytoplasm of endothelial cells. Swelling of cellular elements (cytotoxic edema) also has been noted.
Alterations in CSF resorption exacerbate cerebral edema and increase intracranial pressure further. In experimental meningitis, resorption of CSF is diminished because an accumulation of proteins, leukocytes, and other materials interferes with the function of the arachnoid villus.
During the course of meningitis, excess secretion of antidiuretic hormone (ADH) occurs, which induces water retention and exacerbates electrolyte abnormalities already created secondary to the inflammatory process occurring in the CNS. Cellular electrolyte disturbances may depolarize neuronal membranes, predisposing the host to seizure activity. Increased oxidation of glucose, increased lactate production, and depletion of high-energy compounds such as adenosine 5′-triphosphate and phosphocreatine are observed. Hypoglycorrhachia results primarily from decreased transport of glucose across the inflamed BBB and from increased use of glucose by host tissues. Use of glucose by bacteria and polymorphonuclear leukocytes is of less relative importance.
Clinical Manifestations and Pathophysiologic Relationships
The clinical features of bacterial meningitis in infants and children can be subtle, variable, and nonspecific, or even absent. In infants, they might include fever, hypothermia, lethargy, irritability, poor feeding, vomiting, diarrhea, respiratory distress, seizures, or bulging fontanelles. In older children, clinical features might include fever, headaches, photophobia, nausea, vomiting, confusion, lethargy, or irritability. Signs of meningeal irritation are present in 75% of children with bacterial meningitis at the time of presentation. In contrast, a retrospective review of 326 children presenting to a pediatric emergency department in the Netherlands between 1988 and 1998 with meningeal irritation revealed that 30% had bacterial meningitis. Absence of meningeal irritation in children with bacterial meningitis was substantially more common in those younger than 12 months of age ( Table 31.6 ).
Selected Findings | Infants | Children | Adults |
---|---|---|---|
Underlying disorders | 27–32 | 10–21 | 53–92 |
Headache | NA | 15–92 | 31–87 |
Fever | 34–98 | 88–97 | 42–97 |
Neck stiffness | 9–54 | 40–80 | 50–88 |
Bulging fontanelle | 14–42 | NA | NA |
Altered mental status | 34–79 | 53–83 | 32–91 |
Coma | 1–40 | 7–11 | 11–19 |
Seizures | 16–62 | 19–41 | 5–21 |
Focal neurologic deficits | 4–16 | 8–34 | 10–42 |
Cranial nerve palsies | 10 | 10 | 10–21 |
Hearing loss | NA | 23–34 | 9 |
Inflammation of the meninges generally is associated with nausea, vomiting, irritability, anorexia, headache, confusion, back pain, and nuchal rigidity. In many cases, Kernig and Brudzinski signs are noted. The Kernig sign is present when the leg is flexed 90 degrees at the hips and cannot be extended more than 135 degrees. The Brudzinski sign is present if the thighs and legs are flexed involuntarily when the neck is flexed. All of these findings suggest irritation of inflamed sensory nerves, which produces a reflex contraction of certain muscles in an attempt to minimize pain. These findings also can be the result of increased intracranial pressure and an associated distortion of nerve roots. These signs can be accompanied by hyperesthesia and photophobia. Currently no satisfactory pathophysiologic explanation for photophobia exists.
As indicated earlier, signs of meningeal inflammation may be minimal in the infant, but irritability, restlessness, and poor feeding may be noted. Nuchal rigidity and Kernig and Brudzinski signs may occur late in a young child. Nuchal rigidity may not be elicited in comatose patients or when signs of focal or diffuse neurologic impairment are present. At the time of initial evaluation, 60% to 80% of children have a stiff neck. A review of 1064 cases of bacterial meningitis in children beyond the neonatal period revealed that 16 (1.5%) had no meningeal signs during their entire period of hospitalization, despite the presence of CSF pleocytosis. Fever, a hallmark of infection, generally is present; its absence in a patient with signs of meningeal inflammation, although infrequent, is not unusual.
The relative frequency with which selected findings in infants, children, and adults with bacterial meningitis occur are summarized in Table 31.6 .
Increased intracranial pressure is the rule; it may be reflected by complaints of headache in older children and by a bulging fontanelle and diastasis of sutures in infants. Papilledema is an uncommon finding in acute meningitis, presumably because of the brief duration of increased pressure at the time of diagnosis. When papilledema is observed, venous sinus occlusion, subdural empyema, or brain abscess should be sought.
A major contributing factor to increased intracranial pressure in bacterial meningitis is the development of cerebral edema. Vasogenic edema occurs as a consequence of increased permeability of the BBB. Interstitial edema may occur secondary to decreased clearance of CSF at the arachnoid villi and subsequent obstructive hydrocephalus. Cytotoxic cerebral edema mediated by the release of toxic factors from neutrophils, glial cells, and bacteria leads to increased concentration of intracellular water and sodium and loss of intracellular potassium. In many cases, meningitis is associated with the release of ADH, causing water retention and a relative dumping of sodium by the kidney. If the patient is given excessive free water during therapy, a further increase in intracranial pressure may be noted.
Transient or permanent paralysis of cranial nerves may be noted. Deafness or disturbances in vestibular function are common findings; optic nerve involvement with blindness rarely occurs. Involvement of the eighth cranial nerve may reflect disease at the level of the cochlear and vestibular end organs, which may be related to concomitant infection of the inner ear. Paralysis of extraocular and facial nerves may be noted. Torticollis has been reported in two children with partially treated meningitis. Obtundation, stupor, coma, and focal neurologic signs may be seen in children with bacterial meningitis.
Table 31.7 shows data for 235 children with bacterial meningitis in a prospective study analyzed according to the type of organism responsible for meningitis. Overall, 14.9% of children were semicomatose or comatose at the time of admission; rates for children with pneumococcal or meningococcal meningitis were higher than rates for children with H. influenzae disease. Focal neurologic signs were present at the time of admission in 16.5% of the total group (34.3% of children with pneumococcal meningitis). The presence of focal neurologic signs at the time of admission indicated a poor prognosis and could be correlated with persistent abnormal neurologic examinations at 1, 3, and 6 months ( P < .01) and at 1 year after discharge ( P < .03). The presence of focal signs at the time of admission also correlated with the presence of retardation ( P < .001), as determined by detailed psychometric testing after discharge.
Total Group | H. influenzae | S. pneumoniae | N. meningitidis | |
---|---|---|---|---|
No. patients | 235 | 151 | 35 | 26 |
Level of consciousness (%) | ||||
Irritable or lethargic | 184 (78.3) | 117 (77.5) | 24 (68.6) | 21 (80.8) |
Somnolent | 16 (6.8) | 13 (8.6) | 1 (2.8) | 1 (3.8) |
Obtunded-semicomatose | 27 (11.5) | 15 (9.9) | 8 (22.9) | 4 (15.4) |
Comatose | 8 (3.4) | 6 (4) | 2 (5.7) | 0 (0) |
Focal neurologic signs on admission (%) | 37 (16.5) | 22 (14.6) | 12 (34.3) | 2 (7.7) |
Seizures before admission (%) | 48 (20.4) | 35 (23.2) | 8 (23) | 3 (11.5) |
Seizures in hospital (%) | 61 (26) | 43 (28) | 12 (34) | 5 (19) |
Generally when focal signs are noted in the absence of seizures, cortical necrosis, occlusive vasculitis, or thrombosis of cortical veins has occurred. Thrombosis of meningeal vessels or cortical necrosis may be associated with hemiparesis or quadriparesis and with focal seizures. These signs may appear during the first 3 or 4 days of illness or, less commonly, may be noted after the first or second week of infection. A highly significant association ( P < .001) between neurologic signs indicative of cerebral injury and late afebrile seizures (1 to 15 years after the acute infection) has been noted. Ataxia has been a presenting sign of meningitis in children and adults. Adolescents with meningitis may present with behavioral abnormalities that may be confused with drug abuse or psychiatric disorders.
Approximately 20% of children with bacterial meningitis experience seizures before admission, and approximately 26% have them during the first or second day in the hospital. A retrospective review examined the frequency of seizures before or at the time of presentation in children with meningitis. Seizures at or before the time of diagnosis occurred in 111 of 410 (27%) children with bacterial meningitis; 88 of these children had complex seizures (focal, prolonged, or more than one in a 24-hour period). All children with bacterial meningitis who presented with seizures had other signs or symptoms of meningitis, such as altered level of consciousness, nuchal rigidity, or complex seizures and petechial rash. The frequency of seizure activity is similar for children with H. influenzae type b or pneumococcal meningitis; seizures occur in children with meningitis caused by these organisms approximately twice as frequently as in children with meningococcal meningitis. In a multicenter study of 181 children with pneumococcal meningitis, 41 (23%) developed seizures before admission; 74% of preadmission seizures were generalized.
Overall, seizures are noted in approximately 30% of children with bacterial meningitis in the prospective study. Seizures noted before or during the first several days of hospitalization are of no particular prognostic significance. Specifically their occurrence does not herald the development of a permanent seizure disorder. Seizures that are difficult to control or that persist beyond the fourth hospital day and seizures that occur for the first time late in the hospital course may be of greater significance and have been associated with permanent sequelae of meningitis. Children with focal seizures have a greater likelihood for development of sequelae of meningitis than do children with generalized seizure activity. Focal or prolonged seizures probably indicate serious cerebral vascular disturbances or cerebral infarction. Seven percent of patients with bacterial meningitis have focal or generalized seizures 3 months to 15 years after recovery from bacterial meningitis.
Collections of fluid in the subdural space can be shown in 50% of infants and children during acute illness. In a prospective study of infants 1 to 18 months old with bacterial meningitis, subdural effusions were noted in 43% of infants with H. influenzae meningitis, 30% of infants with pneumococcal meningitis, and 22% of infants with meningococcal meningitis. Subdural effusions were found in 24% (25 of 103) of children undergoing neuroimaging in the multicenter pediatric surveillance study of pneumococcal meningitis. No greater incidence of neurologic sequelae or developmental delay was found on long-term follow-up in patients with effusion compared with patients with bacterial meningitis who did not have effusion. Subdural effusions may cause enlargement in head circumference or may be responsible for abnormal transillumination of the skull, and vomiting, seizures, a full fontanelle, focal neurologic signs, or persistent fever sometimes may be noted.
Blindness and optic atrophy may be related to optic arachnoiditis or infarction of the occipital lobe. Spastic paraparesis with sensory loss in the lower extremities may be secondary to meningomyelitis, spinal cord infarction, or both.
Shock may be associated with any form of overwhelming bacteremia, but it occurs most often in patients with fulminant meningococcemia. In a prospective study, 3.8% of children with meningococcal meningitis developed profound hypotension. In the same study, shock occurred in 5.5% of children with H. influenzae meningitis. Endotoxin has been detected by limulus lysate assay in the blood and CSF of children with meningococcal and H. influenzae meningitis. Sixteen percent of the children in the multicenter pneumococcal meningitis study were in shock on admission. Signs of disseminated intravascular coagulation may accompany hypotension in these patients.
Facial cellulitis (including buccal and periorbital cellulitis), epiglottitis, endophthalmitis, and other suppurative manifestations can manifest at the time of admission in any patient with bacterial meningitis. In one study of children with buccal cellulitis, less than 10% (7 of 73 children) had concomitant bacterial meningitis documented by lumbar puncture as part of their initial evaluation. Five of the seven children had no clinical evidence of meningeal irritation. H. influenzae and S. pneumoniae were cultured from two patients with periorbital cellulitis, and no clinical evidence of meningeal irritation or abnormal CSF cell counts or results of chemistries was found. In the largest review of facial cellulitis caused by S. pneumoniae, 15 of 52 children had a lumbar puncture performed; 2 (13.3%) had a pleocytosis (18 white blood cells [WBCs]/mm 3 —52% polymorphonuclear leukocytes, and 9 WBCs/mm 3 —91% polymorphonuclear leukocytes). The Gram stain and cultures of the CSF specimens were negative. Lumbar puncture should be considered for children with facial cellulitis who are younger than 18 months of age and possibly bacteremic with S. pneumoniae.
Arthralgia and myalgia are noted in many patients with bacterial meningitis, reflecting the systemic nature of the disease. Arthritis also may occur and does so most commonly during the course of meningococcal disease; generally it is transient. Early findings of arthritis may be related to direct invasion of the joint by the meningococcus. Arthritis that develops late in the course of meningococcal or H. influenzae meningitis may be an immune complex–mediated event. Petechial or purpuric lesions may be seen in 50% of patients with meningococcal meningitis but also may accompany any infectious or noninfectious disease process in which vasculitis occurs. Purpura, shock, and hypothermia indicate a poor prognosis.
Pericardial effusions may be present; they generally resolve during the course of antibiotic therapy. In some cases, pericardial effusions are the cause of persistent fever, and pericardiocentesis or an open drainage procedure may be required.
Differential Diagnosis
The signs and symptoms described earlier suggest meningeal or intracranial pathologic processes but are not pathognomonic of acute bacterial infection. Tuberculous meningitis, fungal meningitis, aseptic meningitis, brain abscess, intracranial or spinal epidural abscesses, cranial osteomyelitis, subdural empyema, bacterial endocarditis with embolism, ruptured dermoid cysts, ruptured spinal ependymomas, and brain tumors may show similar signs and symptoms. Differentiation of these disorders depends on careful examination of CSF obtained by lumbar puncture and additional immunologic, radiographic, and imaging studies, as delineated later.
Recurrent bacterial meningitis may be the result of a bacterial infection in the setting of an immunocompromised condition or a communication between the nasal passage or ear and the meninges from congenital anatomic defect or the result of trauma. If rhinorrhea or otorrhea is present, a leak may be suspected, but documenting that CSF is present and locating the site of leakage are difficult when the sample is small or contaminated. Sectional (2 mm) coronal cranial computed tomography (CT) is a noninvasive method for delineating anatomic abnormalities in children with recurrent meningitis.
Protein electrophoresis of CSF revealed an extra band of transferrin located in the β 2 -fraction. This extra β 2 -transferrin band could not be shown in serum, nasal secretions, saliva, tears, or perilymph and endolymph, suggesting that this immunochemical method is useful for documenting that fluid found draining from the nose or ear was CSF. The amount of sample required is small (<50 µL). Differential suction may permit demonstration of the site of the anatomic communication among the nose, the ear, and the meninges. Moderate contamination with other body fluids does not invalidate the method. β-trace protein has also shown to be useful for the reliable diagnosis of CSF leaks, and the method is noninvasive and safe for the patient.
Noninfectious causes of meningitis include medications such as nonsteroidal antiinflammatory drugs (NSAIDs), trimethoprim-sulfamethoxazole, isoniazid, metronidazole, intravenous immune globulin treatment, Behçet syndrome, systemic lupus erythematosus, mixed connective tissue disease, Kawasaki disease, sarcoidosis, familial Mediterranean fever, Vogt-Koyanagi syndrome, procedures involving the CNS (neurosurgery, spinal anesthesia, intrathecal injection), subarachnoid hemorrhage, vein of Galen aneurysm, Mollaret meningitis, intracranial/intraspinal tumors, and cysts.
Diagnosis
Early diagnosis and treatment of bacterial meningitis are imperative in reducing mortality rates and morbidity. Physicians must perform a lumbar puncture on any child in whom they suspect the diagnosis after a careful history and physical examination have been performed, unless specific contraindications to this procedure are present (e.g., clinical signs of increased intracranial pressure in a patient with a closed fontanelle and closed sutures, evidence of mass lesions, cardiopulmonary compromise).
Measurement of opening pressure, often neglected in infants and young children, is an important component of each CSF examination. When the pressure is very high, only enough fluid to permit a careful examination should be removed. Compression of the jugular vein should be avoided unless compression of the spinal cord is suspected. The CSF appearance can be cloudy, stemming from high concentrations of bacteria, protein, WBCs, and/or red blood cells (RBCs). Xanthochromic CSF derives its color primarily from bilirubin pigment. Hemorrhage, bilirubin staining in icteric patients who have meningitis (i.e., neonates, patients with leptospirosis), or an elevated protein concentration of CSF may be associated with xanthochromia.
CSF should be examined immediately. The total number of WBCs should be counted in a counting chamber, and, after cytocentrifugation, a differential cell count should be done on a Wright-stained smear of the sediment. The normal CSF of children age 3 months or older contains less than 6 WBCs/mm 3 . Ninety-five percent of children older than age 3 months have no polymorphonuclear leukocytes in the CSF; the presence of a polymorphonuclear leukocyte in the CSF may be regarded as abnormal. When a lumbar puncture has been performed in a febrile child and a single polymorphonuclear leukocyte has been noted, careful clinical observation is imperative, and treatment should be considered until the results of the culture of the CSF are known.
If the lumbar puncture has been traumatic, a total cell count can be done in a counting chamber. The RBCs can be lysed by acetic acid, and a cell count can be repeated. If the total number of WBCs compared with the number of RBCs is greater than that in whole blood, one can assume the presence of CSF pleocytosis. One simple way to estimate the WBC count in the presence of RBCs is to allow 1 WBC per 1000 RBCs/mm 3 . However, this adjustment cannot be reliably performed in the presence of clot. CSF protein should be measured (usually elevated in bacterial meningitis), and the CSF glucose concentration should be compared with the blood glucose concentration that has been obtained concomitantly. In patients with bacterial meningitis, depression of CSF glucose and of the ratio of CSF to blood glucose (normally 40% to 60%) is the rule ( Table 31.8 ).
Condition | Pressure (mm H 2 O) | Leukocytes/µL | Protein (mg/dL) | Sugar (mg/dL) | Specific Findings |
---|---|---|---|---|---|
Acute bacterial meningitis | Usually elevated; average 300 | Several hundred to >10,000; usually a few thousand; occasionally <100 (especially meningococcal or early in disease); PMLs predominate | Usually 100–500, occasionally >1000 | <40 in more than half the cases | Organism usually seen on smear or recovered on culture in >90% of cases |
Subdural empyema | Usually elevated; average 300 | <100 to a few thousand; PMLs predominate | Usually 100–500 | Normal | No organisms on smear or by culture unless concurrent meningitis |
Brain abscess | Usually elevated | Usually 10–200; fluid rarely is acellular; lymphocytes predominate | Usually 75–400 | Normal | No organisms on smear or by culture |
Ventricular empyema (rupture of brain abscess) | Elevated | Several thousand to 100,000; usually >90% PMLs | Usually several hundred | Usually <40 | Organisms may be seen on smear or cultured |
Cerebral epidural abscess | Slight to modest elevation | Few to several hundred or more cells; lymphocytes predominate | Usually 50-200 | Normal | No organisms on smear or by culture |
Spinal epidural abscess | Usually reduced with spinal block | Usually 10–100; lymphocytes predominate | Usually several hundred | Normal | No organisms on smear or by culture |
Thrombophlebitis (often associated with subdural empyema) | Often elevated | Few to several hundred; PMLs and lymphocytes | Slightly to moderately elevated | Normal | No organisms on smear or by culture |
Bacterial endocarditis (with embolism) | Normal or slightly elevated | Few to <100; lymphocytes and PMLs | Slightly elevated | Normal | No organisms on smear or by culture |
Acute hemorrhagic encephalitis | Usually elevated | Few to >1000; PMLs predominate | Moderately elevated | Normal | No organisms on smear or by culture |
Tuberculous infection | Usually elevated | Usually 25–100, rarely >500; lymphocytes predominate except in early stages when PMLs may account for 80% of cells | Nearly always elevated; usually 100–200; may be much higher if block in CSF flow | Usually reduced; <50 in 75% of cases | Acid-fast organisms may be seen on smear of protein coagulum (pellicle) or recovered from inoculated guinea pig or by culture |
Cryptococcal infection | Usually elevated | Average 50 (0–800); lymphocytes predominate | Average 100; usually 20–500 | Reduced in more than half of cases; often higher in patients with concomitant diabetes mellitus | Organisms may be seen in India ink preparation and on culture (Sabouraud medium); usually grow on blood agar; may produce alcohol in CSF from fermentation of glucose |
Syphilis (acute) | Usually elevated | Average 500; usually lymphocytes; rarely PMLs | Average, 100; γ-globulin often high, with abnormal colloidal gold curve | Normal (rarely reduced) | Positive reagin test result for syphilis; spirochete not demonstrable by usual techniques of smear or by culture |
Sarcoidosis | Normal to considerably elevated | 0 to <100 mononuclear cells | Slight to moderate elevation | Normal | No specific findings |
Separate smears should be made, and one smear should be Gram-stained for bacteria. A Kinyoun stain for mycobacteria is performed if tuberculous meningitis is suspected. The probability of visualizing bacteria on a Gram stain of CSF depends on the number of organisms present. The percentage of positive smears is 25% with less than 10 3 CFU/mL, 60% in the range of 10 3 to 10 5 CFU/mL, and 97% with greater than 10 5 CFU/mL. Gram stain is positive in about 90% of children with pneumococcal meningitis, about 80% of children with meningococcal meningitis, half of patients with gram-negative bacillary meningitis, and a third of patients with Listeria meningitis. Cytospin centrifugation increases the chances of detecting organisms in Gram-stained CSF. A low CSF WBC count with positive Gram stain is a risk factor for an unfavorable outcome. Quellung and agglutination reactions can provide immediate identification of various organisms if the appropriate type of specific antisera is available.
Treating a child with bacterial meningitis with an antibiotic before performing initial lumbar puncture usually does not alter markedly the cell counts or chemical results obtained ( Table 31.9 ). Even when children received appropriate antibiotics for meningitis intravenously for 44 to 68 hours, the bacterial character of the chemical and cellular findings could be discerned in most cases. CSF culture, however, can be negative in children who receive antibiotic treatment before CSF examination. Complete sterilization of N. meningitidis occurred within 2 hours of giving a parenteral third-generation cephalosporin and the beginning of sterilization of S. pneumoniae from CSF by 4 hours into treatment. In such children, pleocytosis and an increased CSF protein concentration are usually sufficient to establish the diagnosis of bacterial meningitis. Blood cultures or nonculture diagnostic tests can help in identifying the infecting organism.
Untreated | Pretreated | |
---|---|---|
No. patients | 143 | 91 |
Total white blood cell count: 10 3 | ||
Mean ± 1 SD | 4.9 ± 6.5 | 4.1 ± 5 |
Range | 0–55 | 0.006–25.5 |
Percentage polys | ||
Mean ± 1 SD | 84 ± 21 | 81 ± 25 |
Range | 0–100 | 0–100 |
Glucose (mg/dL) | ||
Mean ± 1 SD | 35 ± 28 | 32 ± 25 |
Range | 0–109 | 0–100 |
CSF/blood glucose (%) | ||
Mean ± 1 SD | 29 ± 21 | 29 ± 21 |
Range | 0–78 | 0–94 |
Protein (mg/dL) | ||
Mean ± 1 SD | 226 ± 228 | 174 ± 193 |
Range | 13–2290 | 10–1640 |
Culture positive | 135 | 71 |
Gram positive | 114 | 62 |
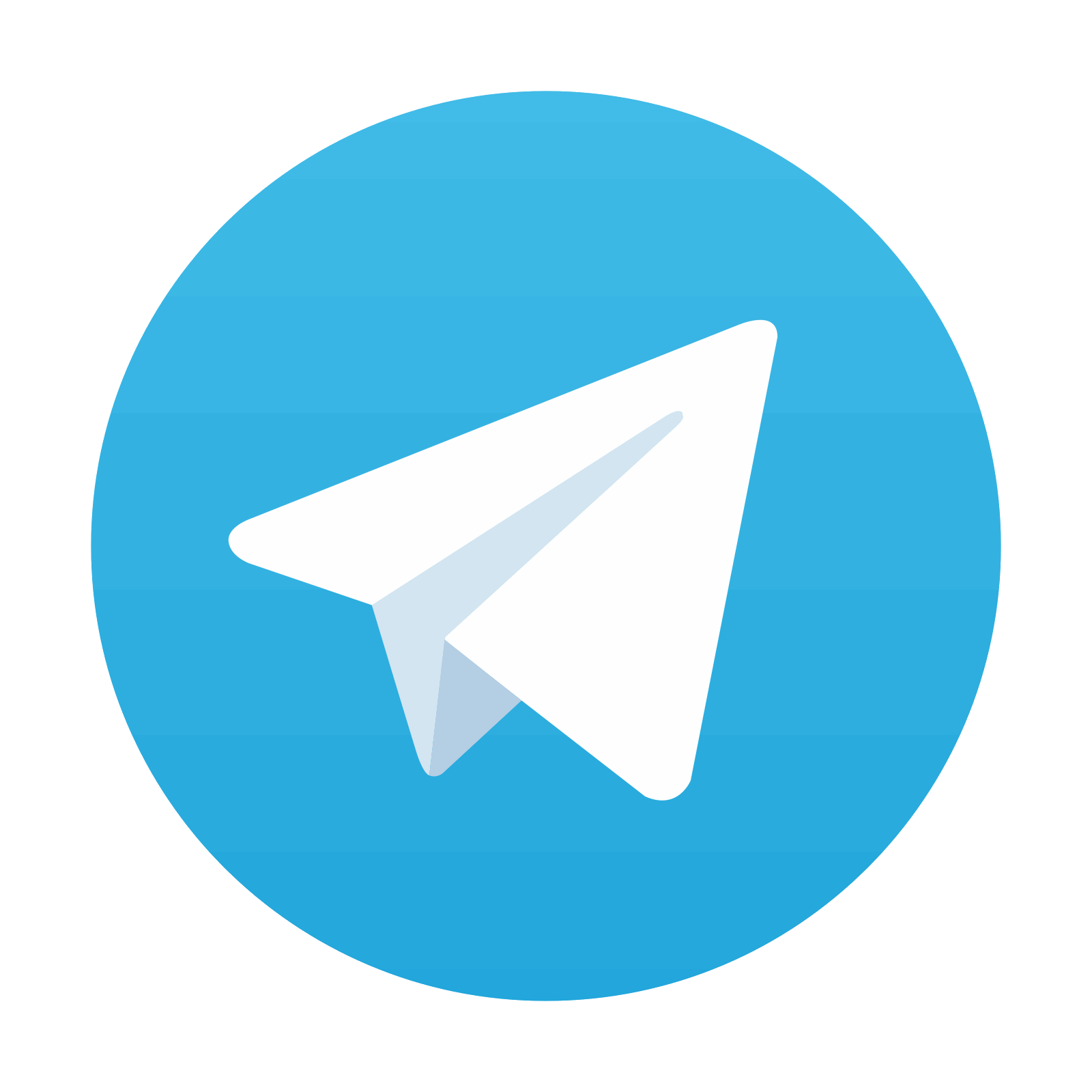
Stay updated, free articles. Join our Telegram channel

Full access? Get Clinical Tree
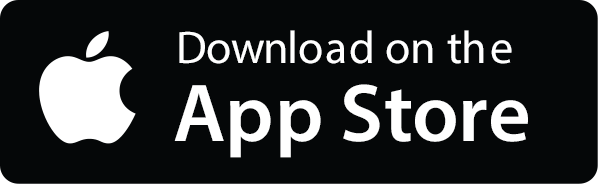
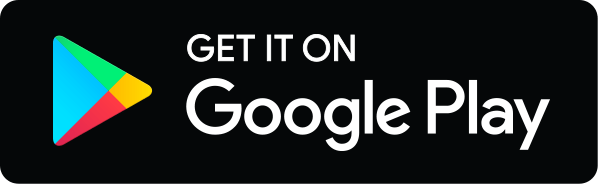
